In-Depth Analysis of Myocytes: Structure and Function
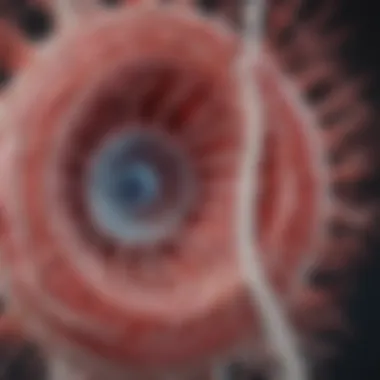
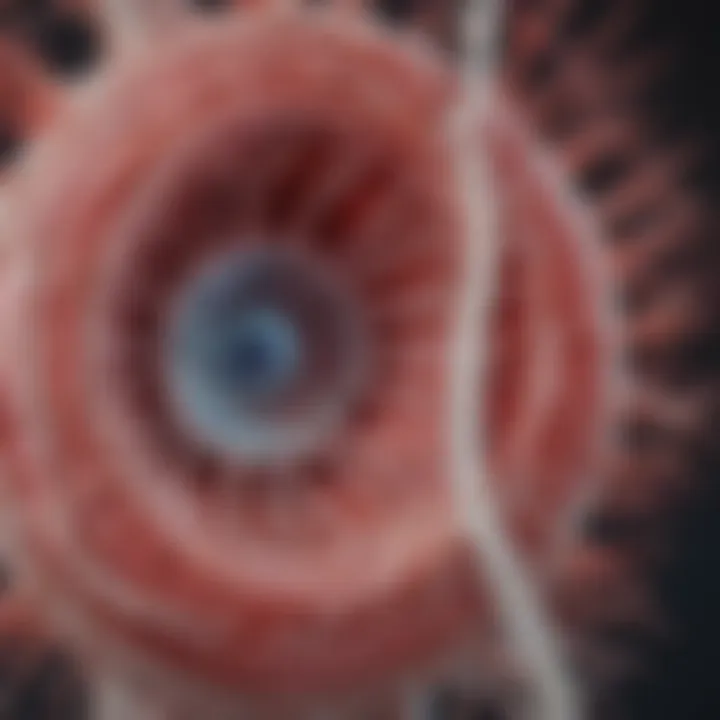
Intro
Myocytes are fundamental to the muscular systems across various organisms. These cells, responsible for muscle contraction, contribute to diverse physiological functions. An understanding of their structure and function is crucial for both basic biology and advanced medical research. This article delves into the intricate world of myocytes, offering insights into their variations and roles.
Methodology
The exploration of myocytes involves various research methods to gather data and analyze findings.
Overview of research methods used
The study of myocytes typically encompasses a combination of histological, anatomical, and molecular biology techniques. Histological methods help in visualizing the structure of myocytes while anatomical studies provide insights on their spatial organization within tissues. Molecular biology techniques enable researchers to investigate the gene expression profiles associated with different myocyte types and conditions.
Data collection techniques
Data collection for myocyte research often involves:
- Tissue samples: Obtained from animal models or human biopsies.
- Imaging techniques: Including microscopy to assess cellular structure.
- Genetic analysis: Employing techniques such as PCR to study gene expression levels.
- Functional assays: Measuring aspects like contraction strength and fatigue resistance.
These methods allow researchers to build a comprehensive understanding of myocyte biology and its implications in health.
Structure of Myocytes
Myocytes exhibit distinct structural characteristics depending on their type. There are three primary types of myocytes: skeletal, cardiac, and smooth. Each type has unique structural elements.
- Skeletal myocytes: These are long, striated, and multinucleated. They attach to bones and facilitate voluntary movements.
- Cardiac myocytes: Found in the heart, these cells are striated but usually contain one or two nuclei. They are connected by intercalated discs, allowing synchronized contractions.
- Smooth myocytes: These are non-striated, spindle-shaped, and contain a single nucleus. They are found in the walls of hollow organs and involuntary muscles.
Understanding these structures is vital for comprehending their functions and interactions in health and disease.
Function of Myocytes
Myocytes have essential roles in muscle contractions and various physiological processes.
- Skeletal myocytes: These cells facilitate movement through voluntary contractions. They respond to nervous input, making movements precise and controlled.
- Cardiac myocytes: Their role is critical in maintaining blood circulation. Rhythmic contractions occur automatically under cardiac pacemaker control.
- Smooth myocytes: These cells regulate involuntary movements, such as peristalsis in the digestive system and vasoconstriction in blood vessels.
Each type of myocyte contributes to the overall functionality of the organism, emphasizing their importance in health and disease contexts.
Implications for Health and Disease
Myocyte dysfunction can lead to a variety of health issues, including muscular dystrophies and heart diseases. Understanding these implications is crucial for effective treatment approaches and preventive measures. Areas such as regenerative medicine are actively studying the potential of myocytes in tissue repair and disease management.
"The study of myocytes not only enhances our understanding of muscular systems but also informs therapeutic strategies for muscle-related diseases."
Research continues to unveil critical connections between myocyte biology and clinical applications. Addressing these implications can lead to significant advancements in medical science.
Future Directions
The field of myocyte research is rapidly evolving. Several areas warrant further investigation.
Upcoming trends in research
- Gene therapy: Advanced research into gene editing technologies could pave the way for new treatments of myocyte-related diseases.
- Stem cell research: Exploring the potential of stem cells to differentiate into myocytes holds promise for regenerative medicine.
Areas requiring further investigation
- Myocyte metabolism: Understanding energy consumption in different myocyte types can reveal insights into performance and fatigue.
- Myocyte interaction: Further study into how myocytes communicate with surrounding cells might unlock new therapeutic targets for muscle diseases.
In summary, myocytes are complex cells integral to muscular function, with significant implications for health. Ongoing research will continue to elucidate these connections, shaping future medical advancements.
Definition of Myocytes
Myocytes are the fundamental muscle cells that play a significant role in enabling movement and maintaining vitality in living organisms. Understanding the definition of myocytes is crucial for appreciating their many functions in muscle physiology. These cells are specialized for contraction, and thus, their structure is intricately designed to support this primary role.
Myocytes can be classified into three main types: cardiac, smooth, and striated. Each type has distinct characteristics and functions that are essential to different systems within the body. This classification not only highlights the diversity within myocytes but also emphasizes their functional significance across various tissues. Knowing these details allows researchers and medical professionals to tailor interventions and understand muscle-related conditions more effectively.
Overview of Muscle Cells
Muscle cells or myocytes are unique in their ability to contract. This contractile property is vital for all forms of movement in organisms. There are different muscle tissues, which are specific to the function and control of movement. They can be voluntary, like skeletal muscle, or involuntary, as seen in cardiac and smooth muscles. The cellular organization reflects the specific needs of each muscle type. Understanding the organization and properties of these cells brings a better comprehension of muscular functions in health and disease.
Types of Myocytes
Cardiac Myocytes
Cardiac myocytes are essential for the heart's functioning. These cells are striated and branched, allowing for synchronized contractions. The unique characteristic of cardiac myocytes is their automaticity; they can generate their own electrical impulses. This feature makes them vital for maintaining a consistent heartbeat. Their interconnectedness through intercalated discs facilitates rapid signal transmission, which is crucial in coordinating heart contractions.
However, this capacity can result in vulnerabilities; cardiac myocytes can be affected by diseases like cardiomyopathy, which can disrupt regular heartbeat and functionality.
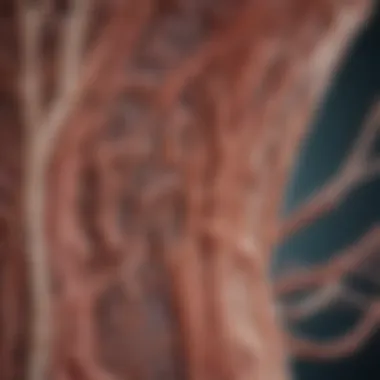
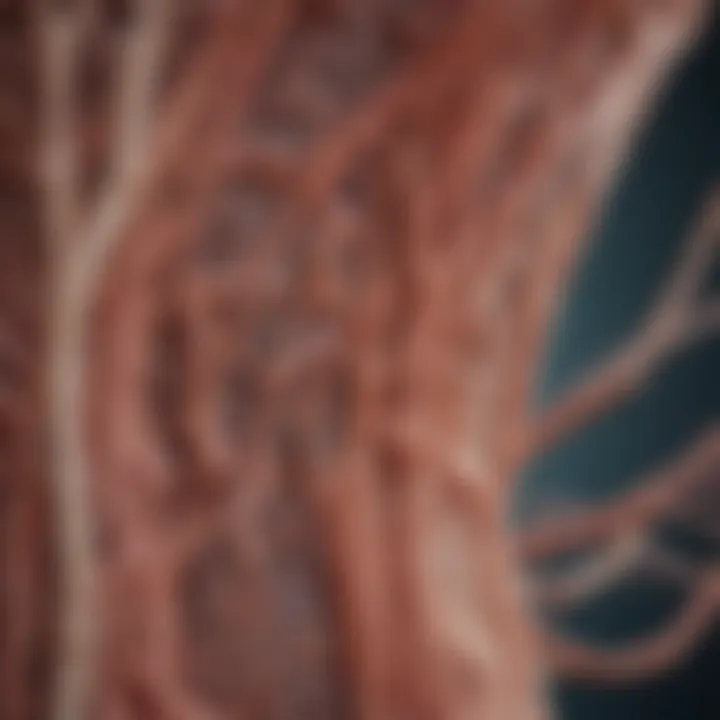
Smooth Myocytes
Smooth myocytes are found in various organs and are responsible for involuntary movements, such as digestion. These cells are spindle-shaped and lack striations, a key feature that differentiates them from other muscle types. Smooth myocytes contract more slowly than cardiac or striated myocytes. The primary advantage of this type is its ability to sustain prolonged contractions without tiring, making it essential for functions like peristalsis in the intestines. However, these cells do not follow a regular pattern of control, which can make their regulation challenging in pathological conditions.
Striated Myocytes
Striated myocytes are primarily found in skeletal muscles, which are under voluntary control. They are long, cylindrical, and striated, allowing for rapid and forceful contractions. The key aspect of striated myocytes is their multi-nucleated nature, which contributes to their strength and ability to generate substantial force. They are essential for movement and maintaining posture.
The major disadvantage is their tendency to fatigue, especially during prolonged activities. This limitation necessitates the need for adequate rest and recovery to maintain functionality and prevent muscle damage.
Understanding the various types of myocytes helps elucidate their specific roles in physiology and pathology, paving the way for better therapeutic strategies.
Structural Composition of Myocytes
The structural composition of myocytes is essential for understanding their functionality and overall importance in muscle physiology. Myocytes are not mere cells; they are sophisticated units capable of contraction and force generation. Each component within a myocyte plays a specific role in supporting these vital functions. Understanding how these structures interact provides insight into muscular health, performance, and abnormalities.
Cell Membrane and Sarcolemma
The cell membrane, particularly the sarcolemma, is crucial in myocyte structure. The sarcolemma is not just a boundary; it permeates signals essential for muscle contraction. It contains receptors for neurotransmitters, ensuring the proper initiation of muscle action. This membrane is also responsible for maintaining the ionic gradients necessary for electrical excitability. This characteristic is vital in transmitting action potentials, which lead to muscle contractions.
Additionally, the sarcolemma features specialized invaginations called T-tubules that allow for deep penetration of the cell. This adaptation facilitates efficient excitation-contraction coupling, ensuring timely communication between the surface membrane and internal components.
Cytoplasm and Sarcoplasm
Cytoplasm in muscle cells is termed sarcoplasm. This gel-like substance contains numerous organelles and myofibrils, which are crucial for contraction. Sarcoplasm is rich in enzymes, ions, and mitochondria, which support energy production and metabolic processes. The abundance of mitochondria is particularly significant, as they supply adenosine triphosphate (ATP), the energy currency for muscle contractions.
Moreover, the sarcoplasm houses the calcium ions that play a pivotal role in muscle contraction. Calcium ions released from the sarcoplasmic reticulum bind to troponin, initiating the contraction process. This interplay demonstrates the importance of the sarcoplasm in the metabolic and functional capabilities of myocytes.
Myofibrils and Contractile Proteins
Myofibrils are the contractile units of myocytes, composed of a series of repeating units called sarcomeres. Within these sarcomeres, actin and myosin filaments work in tandem to produce muscle contraction. Understanding these proteins is crucial as they define how muscle cells operate on a molecular level.
Actin Filaments
Actin filaments are thin filaments that provide the framework for muscle contraction. They have a critical role in the sliding filament theory. This theory explains how muscle fibers shorten during contraction. The primary advantage of actin filaments is their ability to interact with myosin filaments, facilitating the contraction process.
A unique feature of actin filaments is their capability to rapidly polymerize and depolymerize, allowing for quick adjustments needed during various activities. This dynamic nature of actin is beneficial as it enables myocytes to respond promptly to stimuli, which is crucial for muscle function.
Myosin Filaments
Myosin filaments are thick, bipolar filaments crucial for muscle contraction. They possess protruding heads that attach to actin filaments, forming cross-bridges during the contraction cycle. A key characteristic of myosin is its ATPase activity, allowing it to convert ATP into mechanical work.
What stands out about myosin is its power stroke mechanism, which generates force during muscle contraction. However, the reliance on ATP can be a disadvantage, especially in conditions of limited energy supply. Understanding the intricate balance of actin and myosin interactions elucidates the complexities of muscle function and the implications of dysfunction in disease states.
In summary, the structural composition of myocytes can be observed as a synergy of various elements working together harmoniously to enable contraction and maintain muscle health.
"Understanding myocyte structure is not just a biological curiosity; it holds the key to advancing muscle health in clinical settings."
For further reading on sarcolemma, mitochondria function, and contractile proteins, check out Wikipedia and Britannica.
Physiological Roles of Myocytes
The physiological roles of myocytes are crucial for the efficient functioning of muscular systems across various organisms. This section will elaborate on two primary functions: contraction mechanisms and energy metabolism. Both areas are fundamental to understanding how myocytes contribute to muscle function and overall health.
Contraction Mechanisms
Myocytes are specialized for contraction, a process that enables movement and stability in the body. The contraction mechanism involves several steps, primarily hinging on the sliding filament theory. When stimulated, myocytes experience a rapid increase in intracellular calcium concentration. This influx triggers the interaction between actin and myosin filaments, which results in muscle shortening and generation of force.
A significant aspect of contraction is the role of sarcomeres, the structural units within myofibrils. Sarcomeres are composed of overlapping actin and myosin. The ability of myocytes to contract and relax is essential for movements ranging from delicate hand gestures to heavy lifting. Moreover, the contraction process is not solely dependent on physical interactions. It integrates neural signals and metabolic processes.
Understanding these mechanisms provides insight into various conditions where muscle function is compromised. For example, impaired signaling can lead to muscle atrophy, where muscle fibers deteriorate due to lack of activity or neural innervation. Thus, studying contraction mechanisms adds important details to our comprehension of muscular diseases and rehabilitation strategies.
Energy Metabolism
Energy metabolism in myocytes is pivotal for sustaining contraction and maintaining cellular health. It primarily occurs through two pathways: aerobic respiration and anaerobic glycolysis. Both processes provide ATP, the principal energy currency in cells, but they operate under different conditions and yield varying energy outputs.
Aerobic Respiration
Aerobic respiration involves using oxygen to convert glucose and fatty acids into ATP. This process occurs in the mitochondria of myocytes and is characterized by high efficiency. The main advantage of aerobic respiration is its ability to produce a large amount of ATP over an extended duration.
- Key characteristic: Sustained energy production.
- Unique feature: It utilizes oxygen and can metabolize different substrates.
- Advantages: Supports prolonged physical activities, such as endurance running or cycling.
- Disadvantages: Requires adequate oxygen supply, which can be a limitation during intense exercise.
This method of energy production is particularly advantageous for athletes engaged in endurance sports, making it a significant focus in performance training and recovery.
Anaerobic Glycolysis
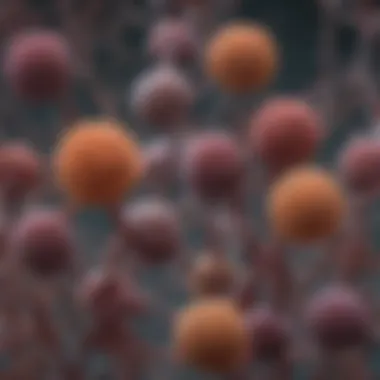
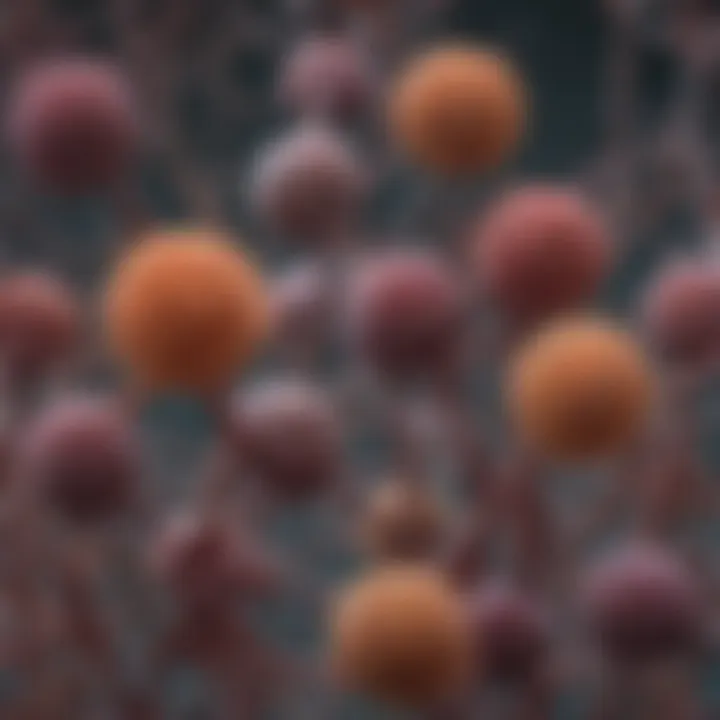
Anaerobic glycolysis is another metabolic pathway that occurs in myocytes when oxygen availability is limited. It converts glucose into ATP quickly, also producing lactic acid as a byproduct. This process is essential during short bursts of high-intensity exercise when energy demand exceeds the supply of oxygen.
- Key characteristic: Rapid ATP production.
- Unique feature: Functions without oxygen.
- Advantages: Supplies immediate energy for activities like sprinting or heavy lifting.
- Disadvantages: Leads to lactic acid buildup, which can cause muscle fatigue and pain.
In summary, myocytes are equipped with specific metabolic pathways tailored for different energy demands, illustrating their adaptive capabilities in various physiological contexts. Understanding these roles enhances our approach toward sports science, rehabilitation, and the treatment of metabolic disorders.
The interplay between contraction mechanisms and energy metabolism within myocytes highlights their significance not just for movement, but also for overall health and homeostasis.
Role in Homeostasis
Myocytes play a critical part in maintaining homeostasis within the body. This concept refers to the body’s ability to maintain stable internal conditions despite external changes. Myocytes, as the primary muscle cells, are involved in multiple functions, from movement to blood circulation. Their role in homeostasis extends beyond mechanical activity, influencing various physiological processes that are essential for survival.
Adaptation to Stress
Myocytes exhibit remarkable adaptability to various stressors, including mechanical overload or metabolic demands. When subjected to physical activity or resistance training, these cells can enhance their performance. This adaptation manifests in several ways:
- Increased Mitochondrial Content: Myocytes can increase the number and efficiency of mitochondria, which ultimately boosts energy production.
- Protein Synthesis: Under stress, there is a rise in protein synthesis, specifically for contractile proteins. This process contributes to muscle growth and functional capacity.
- Cell Signaling Pathways: Myocytes activate specific signaling pathways that promote survival and repair. For instance, pathways involved in hypertrophy can help muscle cells grow to accommodate new demands.
This adaptability is vital not only for muscle performance but also for the overall homeostasis of the organism. Without that capacity, the risk of injury or disease would increase.
Muscle Hypertrophy and Atrophy
The processes of muscle hypertrophy and atrophy are crucial in understanding the dynamic nature of myocytes. Muscle hypertrophy refers to the increase in muscle mass due to physical training or other stimuli. In contrast, muscle atrophy is the decrease in muscle size, often caused by disuse, aging, or disease.
- Hypertrophy: When myocytes undergo hypertrophy, they experience several physiological changes:
- Atrophy: On the other hand, muscle atrophy leads to adverse effects:
- Increased Cross-sectional Area: Larger muscle fibers provide greater strength.
- Greater Vascularization: Enhanced blood supply supports the increased metabolic demands of the enlarged muscle cells.
- Metabolic Efficiency: Hypertrophic myocytes show improved performance in energy utilization during contraction.
- Reduced Muscle Strength: Smaller fibers are less capable of generating force, impacting overall function.
- Increased Risk of Injury: Weaker muscles are more susceptible to strains and tears.
- Hormonal Changes: Atrophy may trigger metabolic changes that affect overall health, raising concerns about obesity or diabetes.
Understanding these processes of hypertrophy and atrophy is essential. They illustrate the profound influence of myocytes on muscular health and the fundamental balance needed for homeostasis in our bodies.
Both hypertrophy and atrophy emphasize the spectrum of adaptability and responsiveness of myocytes due to internal and external factors. This control over muscle size and strength is integral to maintaining not only movement but overall body functionality.
Myocyte Regeneration and Repair
Myocyte regeneration and repair represent a crucial area of study in understanding muscle biology. This aspect is particularly important given the challenges faced in muscle recovery following injury or disease. Myocytes exhibit limited intrinsic regenerative capacity. However, certain processes can enhance healing and adaptation. The ability to regenerate is essential not just for recovery but also for maintaining muscle functionality over time. This section will delineate the roles of satellite cells and the overall regenerative capacity of myocytes.
Satellite Cells
Satellite cells are specialized stem cells located near the muscle fibers. Their primary function is to assist in muscle repair and regeneration. When muscle fibers are injured, satellite cells become activated. They undergo proliferation and differentiation to form new muscle fibers or repair existing ones. This process is critical following muscle injuries, strains, or diseases such as muscular dystrophy.
Key points about satellite cells include:
- Location: They reside between the basal lamina and the sarcolemma of muscle fibers.
- Activation: Trauma, stress, and signaling from inflammatory cells trigger their activation.
- Differentiation: Once activated, these cells can become fully functional myocytes, directly participating in muscle repair.
The importance of satellite cells cannot be overstated. They play an essential role in muscle hypertrophy, the increase in muscle mass. For instance, resistance training stimulates satellite cell activity. As a result, this process contributes significantly to the muscle adaptation necessary for strength building.
Regenerative Capacity
The regenerative capacity of myocytes is influenced by various factors. While adult skeletal muscle has some ability to regenerate, it is not as robust as in other tissues. Understanding this capacity is vital for developing treatments for muscle-related disorders.
When myocytes are damaged, the natural repair process involves:
- Activation of Satellite Cells: As mentioned earlier, satellite cells are crucial for initiating muscle repair.
- Inflammation: A well-regulated inflammatory response aids in muscle healing. This phase clears damaged cells and signals for regeneration.
- Collagen Deposition: Proper formation of extracellular matrix is necessary for supporting new muscle fibers.
- Muscle Fiber Formation: Newly formed myocytes fuse with existing fibers or create entirely new ones.
Despite these mechanisms, there are limitations to the regenerative capacity of myocytes. For example, age can diminish the number and functionality of satellite cells. Moreover, chronic conditions such as diabetes and heart disease can impair muscle repair. Exploring strategies to enhance myocyte regeneration holds substantial clinical significance.
"Understanding the mechanisms behind myocyte regeneration can lead to innovative therapies for muscle disorders."
In summary, myocyte regeneration and repair are vital processes. They involve complex interactions between satellite cells and other cellular components. Future research should aim at uncovering methods to enhance these processes, particularly in aging populations and individuals suffering from muscle diseases.
Myocytes in Health and Disease
The role of myocytes in health and disease is crucial for understanding how muscle function can be affected by various conditions. These cells are not just fundamental to muscle contraction; they also play vital roles in maintaining overall health and responding to pathological states. Myocytes can exhibit changes that lead to diseases, impacting both their structure and function, which can ultimately affect the entire muscular system. Exploring these aspects gives insights into possible interventions and therapeutic strategies for various disorders.
Cardiomyopathies
Cardiomyopathies are a diverse group of conditions that affect the heart muscle, specifically cardiac myocytes. These conditions disturb the structural integrity and function of myocytes, leading to impaired cardiac output. Pathological changes in myocytes can result from genetic mutations, chronic stress, inflammatory processes, or ischemic injury.
The mechanisms involved in cardiomyopathies include:
- Hypertrophy: In this condition, myocytes enlarge, which can initially help compensate for increased workload. However, persistent hypertrophy leads to dysfunction and heart failure.
- Dilation: Dilation of the heart chambers can result in weakened myocytes, leading to reduced contractility.
- Fibrosis: Excessive scar tissue formation within the cardiac muscle disrupts normal electrical conduction and mechanical function.
Understanding these changes in cardiac myocytes helps identify potential therapeutic targets. For instance, treatments can be aimed at promoting myocyte survival, inducing regeneration, or addressing underlying metabolic dysfunctions. Patients with cardiomyopathy often benefit from medication, lifestyle modifications, and in severe cases, surgical interventions or heart transplants.
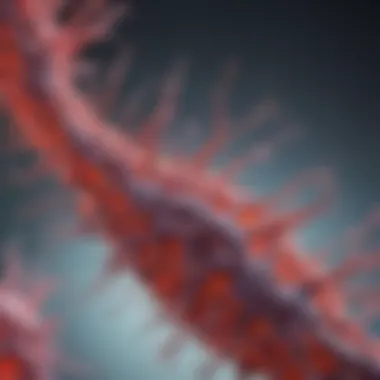
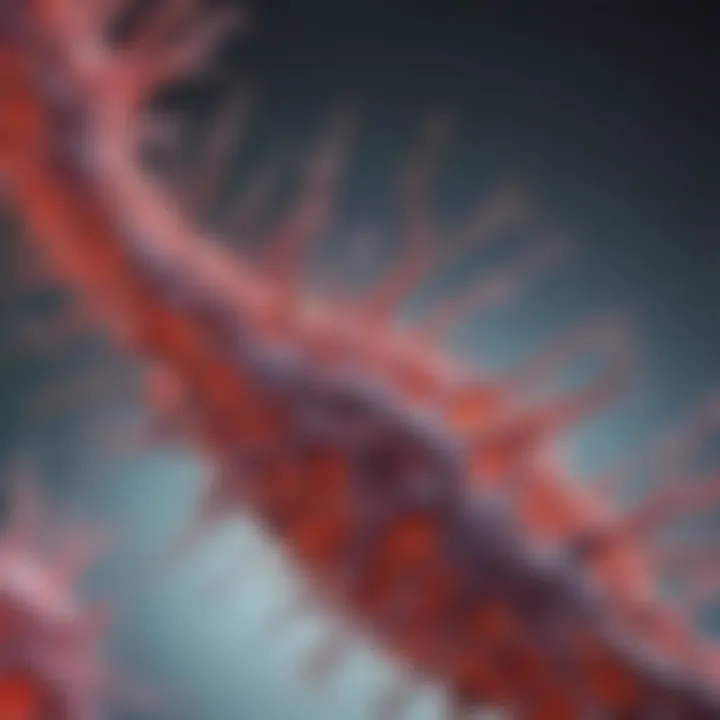
Recent research highlights the importance of early diagnosis and management to prevent the progression of heart diseases related to myocyte dysfunction.
Skeletal Muscle Disorders
Skeletal muscle disorders encompass a variety of conditions that impact skeletal myocytes, including muscular dystrophies, myopathies, and inflammatory diseases such as polymyositis. These disorders often arise due to genetic factors or autoimmune mechanisms, leading to marked muscle weakness and atrophy.
Key features of skeletal muscle disorders include:
- Duchenne Muscular Dystrophy: A severe and progressive disorder caused by dystrophin gene mutations leading to the damage of myocyte membranes. This results in degeneration and replacement of muscle tissue with fibrous and adipose tissue.
- Myotonic Dystrophy: Characterized by delayed muscle relaxation after contraction due to defective ion channel function in myocytes.
- Inflammatory Myopathy: Here, the immune system mistakenly attacks the muscular fibers, leading to notable weakness but often with more fluid and reversible myocyte damage.
Management of these disorders requires a multidisciplinary approach, often combining physical therapy, pain management, and potential pharmacological interventions. Gene therapy and advancements in regenerative medicine hold great promise for treating or even reversing these debilitating conditions in the future.
Understanding the cellular mechanisms behind myocyte health provides pathways for innovative treatments. Progress in medical science offers hope for recovery and improved quality of life for many patients affected by myocyte-related diseases.
Research Advances
In the realm of myocyte research, significant strides have been made in recent years. These advances are crucial to understanding the behavior, healing, and functionality of muscle cells under various conditions. Progress in this field offers promising implications for treating degenerative diseases and muscle injuries. This section delves into important facets of research advances, particularly focusing on stem cell therapies and gene editing techniques that are reshaping our approach to muscle health.
Stem Cell Therapies
Stem cell therapies constitute a groundbreaking area within myocyte research. They harness the unique ability of stem cells to differentiate into various cell types, including myocytes. This capability holds substantial potential for regenerative medicine. Now, researchers are investigating how to effectively deploy stem cells to restore function in injured or diseased muscle tissue.
Some key elements include:
- Source of Stem Cells: Various sources like embryonic stem cells and induced pluripotent stem cells (iPSCs) are being explored for their myogenic properties.
- Application in Therapy: By using stem cells in therapeutic protocols, there is potential for significant improvement in muscle repair post-injury, especially in conditions like muscular dystrophy.
- Considerations: Challenges remain regarding ethical sourcing and the risk of tumorigenesis. Rigorous evaluation and testing are necessary for ensuring safety and efficacy in clinical applications.
“Harnessing stem cells for muscle repair represents an innovative leap towards redefining treatment strategies in muscle degenerative diseases.”
Gene Editing Techniques
Gene editing techniques have sparked a new era of possibilities for myocyte research. The ability to modify genetic material accurately opens doors to repairing genetic defects that contribute to myocyte dysfunction. Technologies such as CRISPR/Cas9 are at the forefront, allowing precise alterations in DNA sequences within myocytes.
Key aspects of gene editing in this context include:
- Targeting Genetic Disorders: Gene editing can potentially correct mutations responsible for various myopathies, providing a direct method for treating the root cause of the disease.
- Precision Medicine: By tailoring interventions to the specific genetic makeup, researchers aim to enhance the effectiveness of therapies while minimizing side effects.
- Ethical and Regulatory Considerations: The path to implementing gene editing technologies in clinical scenarios comes with significant regulatory scrutiny. Ethical implications must be thoroughly evaluated as this technology progresses.
Overall, these research advances serve as a foundation for future breakthroughs in the field of myocytes, contributing to innovative treatments that can profoundly impact the quality of life for individuals with muscle disorders.
Clinical Applications
The clinical applications of myocyte research are vast and crucial. Understanding myocytes, their functions, and how they respond to various stimuli paves the way for new therapeutic avenues. These applications range from rehabilitation strategies to pharmacological interventions, each playing a significant role in the management of muscle-related disorders.
Rehabilitation Strategies
Rehabilitation strategies targeting myocytes focus on restoring muscle function and strength after injury or disease. Various techniques can be employed, including:
- Physical Therapy: Tailored exercises enhance muscle recovery. They also aim to improve coordination and mobility.
- Resistance Training: This approach promotes muscle hypertrophy, encouraging healthy growth of myocytes. Progressive overload is a key component.
- Electrostimulation: Utilizing electrical currents can stimulate myocyte contraction. This is especially useful for patients unable to engage in traditional exercises.
- Nutritional Support: Adequate protein intake supports myocyte repair and growth. Supplements may be considered to aid recovery.
Considering the patient's specific condition is essential in determining the most effective strategy. Individualized protocols can optimize recovery trajectories and enhance functional outcomes.
Pharmacological Interventions
Pharmacological interventions play a pivotal role in modifying myocyte function, especially in pathological conditions. Various classes of drugs can be used:
- Anabolic Steroids: These enhance muscle mass and strength by increasing protein synthesis within myocytes. They are largely used in clinical settings but also have potential misuse in sports.
- Selective Androgen Receptor Modulators (SARMs): SARMs offer similar benefits to anabolic steroids but with a potentially lower side-effect profile. Their effects on myocyte growth are under investigation in clinical trials.
- Beta-Agonists: Used to increase myocyte permeability and stimulate muscle mass growth, these agents could facilitate recovery in specific conditions like heart failure.
- Myostatin Inhibitors: Myostatin is a negative regulator of muscle growth. Inhibition can lead to muscle hypertrophy. This approach is gaining traction in research for conditions like muscular dystrophy.
The consideration of side effects and patient contexts is crucial. Ongoing research seeks to find optimal therapeutic strategies while minimizing adverse effects.
Key Insight: The interplay between rehabilitation and pharmacological interventions harnesses the full potential of myocyte biology, promoting healthier outcomes for patients.
Overall, clinical applications involving myocytes are continuously evolving. As researchers uncover more about myocyte behavior under various conditions, the potential for innovative therapies grows. This inevitably enhances the quality of life for patients suffering from muscle-related conditions, aligning medical advancements with patient care needs.
Future Directions in Myocyte Research
In contemporary biological and medical research, understanding myocytes has led to significant advancements as well as new inquiries. Future directions in myocyte research hold substantial promise for enhancing medical treatment options and improving physical rehabilitation strategies. With the ongoing developments in both technology and methodologies, there are several specific elements to consider, such as the expansion of regenerative medicine, the refinement of therapeutic applications, and the implications for various muscle disorders and diseases.
Emerging Technologies
Emerging technologies are shaping the future of myocyte research in profound ways. Stem cell therapy stands out as a prominent avenue. Researchers are investigating how to harness pluripotent stem cells to generate myocytes for regenerative therapies. This approach not only has the potential to repair damaged tissues but also to restore function in individuals suffering from myocyte-related ailments.
Another notable technology is gene editing. Techniques like CRISPR-Cas9 allow precise modifications to myocyte genetic material. By correcting genetic defects, these technologies may provide new solutions to treat genetic myopathies, enhancing the overall vitality of muscle cells. Moreover, biomaterials and 3D printing are being explored to create scaffolds that can support myocyte survival and function when implanted into damaged muscle tissues.
The use of high-throughput sequencing and single-cell analyses enables researchers to delve deeper into the cellular mechanisms of myocytes. By understanding their molecular signatures and pathways, scientists can identify novel therapeutic targets, which may lead to improved treatments for muscle diseases.
Interdisciplinary Collaborations
Interdisciplinary collaborations are pivotal for advancing myocyte research. The integration of knowledge from various fields such as biology, engineering, and medicine accelerates innovation. For instance, collaboration between engineers and biologists is crucial in developing cutting-edge biomaterials that mimic the extracellular matrix of muscle tissue, thus optimizing tissue repair processes.
Furthermore, partnerships with clinical researchers provide valuable insights into how myocyte research translates to patient care. By sharing data and findings, clinicians and researchers can identify the most promising therapeutic scenarios for muscle injury rehabilitation or chronic muscle diseases.
Additionally, the collaboration between academic institutions and industry has proven beneficial. Industry often brings resources and technology that academic researchers may not have, allowing for faster translation of basic research into practical applications. The shared knowledge from various disciplines fuels a comprehensive understanding of myocytes and their role in health and disease.
In summary, future directions in myocyte research not only promise to unlock new therapeutic possibilities but also highlight the importance of collaborative efforts across disciplines to achieve groundbreaking discoveries.