Understanding DNA Annealing Buffers in Molecular Biology
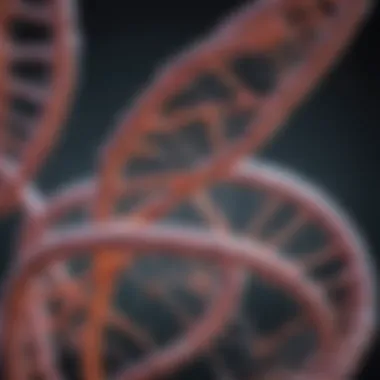
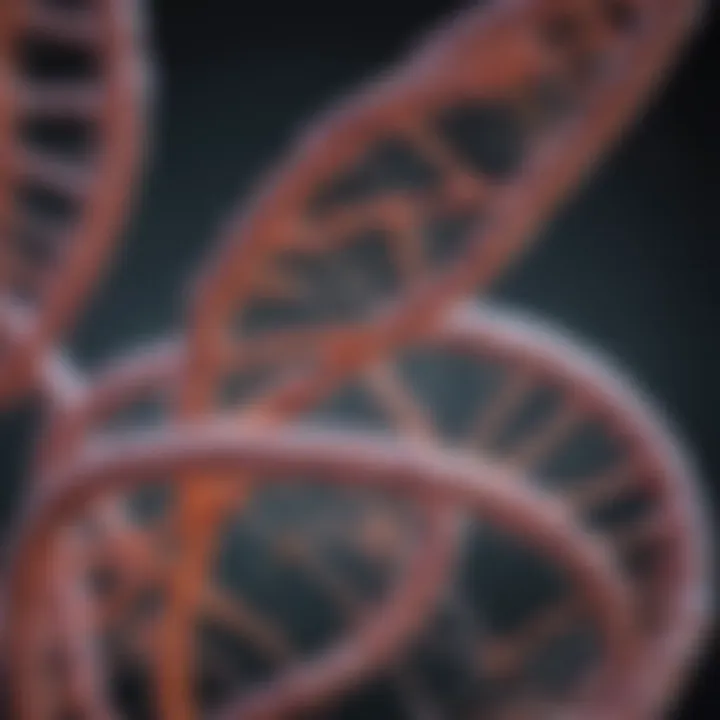
Intro
In the field of molecular biology, the manipulation and study of DNA are fundamental processes. A critical component that surfaces frequently in experiments such as Polymerase Chain Reaction (PCR), cloning, and sequencing is the DNA annealing buffer. Understanding these buffers enables researchers to optimize the conditions under which DNA strands can successfully bind together, leading to accurate and reproducible results. This section will present an overview of methodology related to the use and study of DNA annealing buffers, setting the stage for a deeper exploration of their significance.
Methodology
Overview of Research Methods Used
Research involving DNA annealing buffers typically employs a blend of experimental approaches, including comparative studies and control tests. These methods assess the effectiveness of various buffer compositions in promoting efficient DNA annealing. Researchers carefully design their experiments to establish baseline conditions, which involve controlling variables such as temperature, pH levels, and ionic strength. Specific techniques like agarose gel electrophoresis serve to visualize the results of these experiments, allowing for a clear assessment of annealing efficiency and specificity. Understanding the method behind these experiments is key to deciphering complex data.
Data Collection Techniques
The data collection process in these studies often includes both quantitative and qualitative methods. Here are common techniques:
- Spectrophotometry: This technique is used to measure the concentration of DNA and assess purity.
- Fluorescence assays: These allow for monitoring DNA interactions in real-time, providing insights into kinetics and dynamics during annealing.
- Gel electrophoresis: As mentioned earlier, this is widely used to confirm the success of annealing by separating DNA products based on size and charge.
Researchers systematically gather data across multiple experiments, ensuring that findings are robust and reproducible. By compiling this information, they pave the way for better understanding the role of annealing buffers in DNA manipulation.
Future Directions
Upcoming Trends in Research
As molecular biology continues to evolve, there are several key trends in research involving DNA annealing buffers to watch for:
- Integration with Next-Generation Sequencing: The demand for optimized buffers that support high-throughput methods is on the rise.
- Customized Buffer Solutions: Innovative efforts are underway to develop bespoke buffers tailored for specific DNA sequences or types of experiments.
- Automation in Buffer Preparation: Advancements in robotics may create opportunities for greater consistency in buffer composition.
Areas Requiring Further Investigation
While much is understood about DNA annealing buffers, several areas remain for exploration. The impact of non-standard additives, variations in buffer composition on different types of DNA, and the long-term stability of these buffers are topics that warrant additional research. Improved understanding in these areas can enhance a researcher's capability to design effective experiments.
"The choice and preparation of DNA annealing buffers is a crucial step that can significantly influence experimental outcomes."
In summary, DNA annealing buffers represent an essential component of molecular biology protocols. A thorough comprehension of their functionality, preparation, and impact will deepen researchers' understanding and improve techniques across various applications.
Preamble to DNA Annealing Buffers
DNA annealing buffers are essential components in various molecular biology techniques. They facilitate the annealing of DNA strands, a crucial step in processes like Polymerase Chain Reaction (PCR), cloning, and DNA sequencing. Understanding the role and composition of these buffers can significantly enhance the efficiency of experimental outcomes. This section will provide a comprehensive overview of why DNA annealing buffers are fundamental in the realm of molecular biology.
Definition and Importance
DNA annealing buffers are solutions that create the optimal environment for complementary DNA strands to hybridize or anneal. These buffers usually include salt solutions and various other components that stabilize the DNA structure and enhance the effectiveness of the annealing process.
Their importance cannot be overstated. Without appropriate buffers, DNA strands may not anneal correctly, leading to suboptimal results. Consequently, researchers must pay close attention to buffer selection and preparation when conducting experiments. The ability to produce consistent and reproducible results relies heavily on using the right annealing buffer.
Application in Molecular Biology
The applications of DNA annealing buffers extend across a wide range of molecular biology methodologies. They are integral in:*
- Polymerase Chain Reaction (PCR): In PCR, annealing buffers help in the correct binding of primers to target DNA sequences, influencing the efficiency and specificity of the amplification process.
- Cloning Techniques: For successful cloning, DNA fragments must anneal properly to vectors. Buffers aid in creating the ideal conditions for this to occur, ensuring high cloning success rates.
- Sequencing Applications: In DNA sequencing, annealing buffers assist in maintaining the integrity of DNA templates, which is critical for accurate sequence determination.
These applications underline the significance of understanding and utilizing DNA annealing buffers effectively. They are not merely a complementary aspect of experiments but are vital for achieving high-quality results.
Chemical Composition of DNA Annealing Buffers
The chemical composition of DNA annealing buffers is crucial for the success of molecular biology techniques. These buffers provide the appropriate environment for the DNA strands to interact effectively. Each component plays a significant role in facilitating hybridization, as well as maintaining the integrity of the DNA. The right balance and choice of these components can drastically improve results in experiments such as PCR, cloning, and sequencing. Understanding what makes up these buffers helps researchers achieve optimal conditions that support their experimental goals.
Primary Components
The primary components of DNA annealing buffers typically include salts, buffering agents, and sometimes stabilizers. Each element serves a specific purpose. Salts like sodium chloride are essential for maintaining ionic strength and help stabilize the DNA structure. Commonly used salts include Tris (tris(hydroxymethyl)aminomethane) and EDTA (ethylenediaminetetraacetic acid), which is a chelating agent that prevents degradation of nucleic acids.
There are other components, such as glycerol or DMSO (dimethyl sulfoxide), which may be added to enhance the solubility and stability of the DNA.
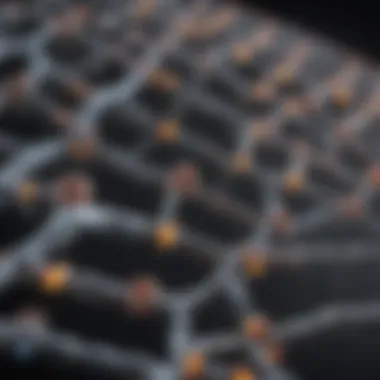
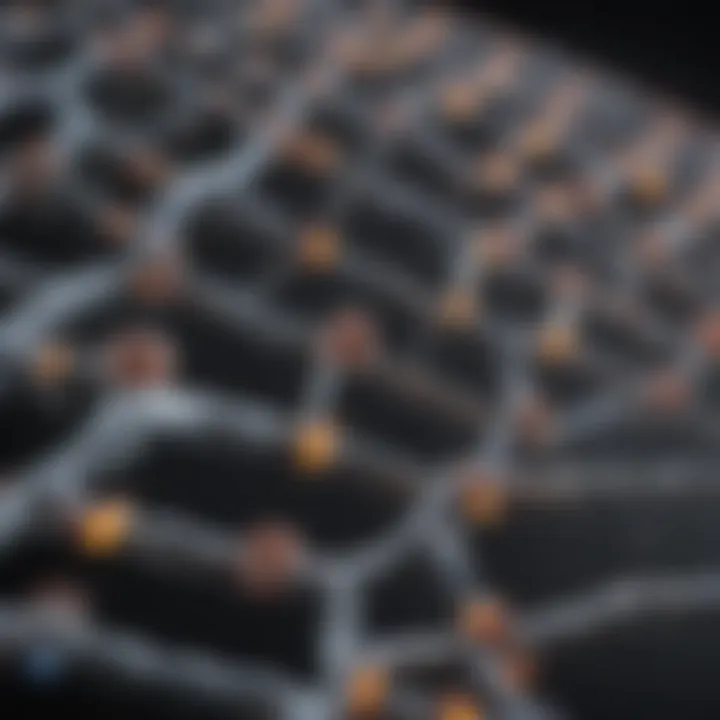
These primary components, combined in a specific ratio, create a buffer system that maintains a suitable pH range for various experiments. For example, a common buffer might consist of Tris at a concentration of 10 mM, 50 mM NaCl, and adjusted to a pH around 8.0. This composition ensures an optimal environment for DNA interactions.
Role of Salts
Salts are vital in DNA annealing buffers. They influence the conductivity of the solution and help to shield the phosphate backbones of the DNA strands from repelling each other. The presence of monovalent cations, like sodium and potassium ions, helps facilitate base pairing between complementary strands. Meanwhile, the specific concentration of sodium chloride can affect the melting temperature (Tm) of the DNA duplex, which is critical for hybrids to form correctly.
Salts contribute directly to the stability of DNA duplexes and overall reaction efficiency.
In practical terms, adjusting salt concentrations can lead to success or failure of a reaction. Too low a salt concentration may result in non-specific binding of DNA, while too high can lead to poor annealing efficiency.
Buffering Agents
Buffering agents maintain the pH of the solution during reactions. Tris, as mentioned earlier, is one of the most commonly used buffers in molecular biology. It has a buffering range that is suitable for biological reactions, typically between pH 7-9. Maintaining this pH range is crucial for DNA stability, as extreme pH can cause denaturation or degradation of the nucleic acids.
Other buffering agents like HEPES (4-(2-hydroxyethyl)-1-piperazineethanesulfonic acid) might also be used for specific protocols, as they can maintain consistent pH levels better under certain conditions, especially when enzymes are involved.
Mechanism of DNA Annealing
Understanding the mechanism of DNA annealing is crucial for grasping how DNA molecules come together during various molecular biology techniques, particularly PCR and cloning. This process involves the hybridization of single-stranded DNA to form a double-strand structure. The efficiency and precision of this mechanism directly impact the results of experimental assays, which makes it a center point of analysis in our guide.
Principles of DNA Hybridization
DNA hybridization is the process where two complementary strands of DNA bind to form a double helix. This process is governed by the complementarity of the nucleotide bases - adenine pairs with thymine, and guanine pairs with cytosine. The degree of binding depends on the concentration of DNA, temperature, and buffer conditions.
A crucial point is the melting temperature, or Tm, which dictates how stable the hybridized product will be. The closer the temperature is to Tm during the annealing phase, the more specific the binding between the strands. Understanding the principles behind DNA hybridization aids researchers in designing more effective experiments.
Factors Affecting Annealing Efficiency
Several factors influence the efficiency of DNA annealing, shaping the success of molecular experiments. Here we explore the three most significant considerations: temperature, concentration of DNA, and buffer composition.
Temperature
The specific aspect of temperature plays a vital role in the DNA annealing process. Temperature affects both the kinetics and thermodynamics of hybridization. For instance, increasing temperature can destabilize hydrogen bonds, while lowering it can promote the formation of these bonds.
A key characteristic of temperature is its influence on the reaction progress. Higher temperatures can reduce non-specific bindings but may also lower the chances of specific bindings if not optimized properly. Understanding the unique features of temperature allows researchers to find a suitable balance between stability and specificity in their protocols.
Concentration of DNA
Another fundamental aspect of the concentration of DNA in the annealing buffer is its significant contribution to hybridization efficiency. A higher concentration of DNA increases the likelihood of molecules colliding with complementary strands, thus promoting effective annealing.
The unique feature of varying concentrations means researchers must find an optimal level. Too low a concentration will result in poor yields, while excessively high concentrations can lead to non-specific binding. Careful considerations of DNA concentration are essential for achieving desired outcomes during molecular experiments.
Buffer Composition
The buffer composition is crucial for maintaining the appropriate environment during annealing. The presence of salts and pH levels significantly influences the stability of the DNA duplex formed. Buffer systems help maintain ionic strength, which impacts the repulsion between negatively charged DNA backbones.
A key characteristic of buffer composition is the tailored nature of different buffers for specific applications. Some buffers are designed to enhance stability, while others might contribute to the specificity of binding. Knowing how different buffers influence the annealing process is beneficial for selecting the right one for specific molecular biology applications.
Understanding these factors helps in fine-tuning the experimental conditions. Adjusting one can often lead to necessity of altering others, creating a delicate balance that ensures successful DNA annealing.
This section provided insights into the underpinning mechanisms of DNA annealing and relevant factors, guiding researchers towards effective methodologies in their experiments.
Preparing DNA Annealing Buffers
Preparing DNA annealing buffers is a crucial process in molecular biology. The quality and composition of the buffer can dramatically affect the success of experiments such as PCR (Polymerase Chain Reaction), cloning, and DNA sequencing. Understanding how to prepare these buffers ensures that researchers can manipulate DNA effectively and obtain reliable results.
Several elements contribute to successful buffer preparation. These include precision, cleanliness, and knowledge of the specific requirements of the task at hand. Small errors in concentration or contamination can lead to inefficient DNA annealing or unexpected results. Thus, having a clear method and following standardized protocols are imperative.
Standard Preparation Protocols
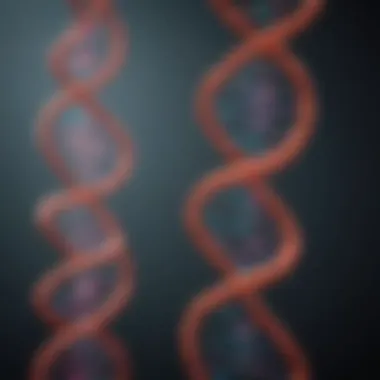
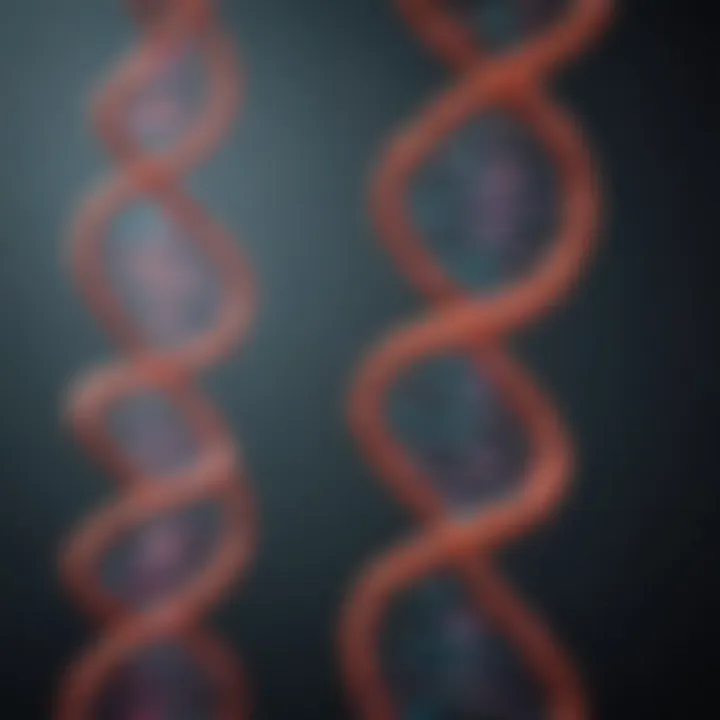
Standard preparation protocols for DNA annealing buffers offer a guideline that scientists can follow. Typically, the procedure may involve the following steps:
- Select appropriate reagents: This includes salts like sodium chloride and buffering agents such as Tris or HEPES. The choice of components will depend on the specific application.
- Calculate concentrations: It's vital to determine the concentrations of each component based on the desired final volume of the buffer.
- Dissolve the reagents: In a clean container, dissolve the components in distilled water with gentle mixing to ensure thorough incorporation.
- Adjust pH as needed: Using a calibrated pH meter, the pH may need to be adjusted to suit the experimental conditions. Many buffers work optimally between pH ranges of 7 to 8.
- Sterilize the buffer: If the buffer will come into contact with sensitive biological materials, filtration through a 0.22-micron filter is recommended.
Optimizing Buffer Conditions
Optimization of buffer conditions is an essential step to enhance the effectiveness of DNA annealing. The buffer must cater to the specific requirements of the reaction conditions. Considerations include:
- Ionic Strength: The ionic strength of the buffer can affect the annealing temperature and stability of DNA duplexes. Increasing ionic strength generally promotes more stable binding of DNA strands.
- Buffer Concentration: Sometimes a higher buffer concentration may be required to ensure effective interactions in complex reactions. This should be tailored based on the precise experimental needs.
- Temperature Adjustment: Setting the buffer at a temperature that matches the DNA sample conditions can promote optimal annealing. It is important to pay attention to the melting temperature (Tm) of the DNA primers used in PCR.
Regularly validating and experimenting with buffer conditions can lead to improved experimental outcomes and higher reproducibility. By adhering to these practices, researchers can finely tune their approaches, ensuring that the buffers serve their intended roles effectively.
Specific Types of DNA Annealing Buffers
DNA annealing buffers are not just one-size-fits-all solutions. Understanding the specific types of these buffers helps researchers navigate their applications more effectively. Each buffer is tailored for particular processes, and choosing the right one can significantly impact experimental outcomes.
Tm Calculators and Adjustments
The concept of melting temperature (Tm) is pivotal in the design and utilization of DNA annealing buffers. Tm refers to the temperature at which half of the DNA strands are in the double-helix state and half are in the "melted" single-stranded state. This temperature varies based on the nucleotide composition and length of the DNA strands involved.
Computing the Tm is essential when setting up reactions, especially in techniques such as PCR. It ensures that annealing occurs efficiently, allowing primers to bind to the target DNA effectively. Researchers often use online Tm calculators that take into account various factors, including:
- Nucleotide composition: GC-rich sequences generally have a higher Tm than AT-rich ones.
- Length of the primers: Longer primers have higher Tm values.
- Salt concentration: The presence of salts can stabilize the DNA duplex, affecting Tm.
By adjusting these parameters, a researcher can predict and modify the annealing conditions appropriately. It is advantageous to experimentally verify Tm values when precision is critical, particularly in sensitive applications such as cloning.
Commercially Available Buffers
Research and industry provide various commercially available DNA annealing buffers designed to simplify the preparation process. These ready-to-use solutions save time and ensure consistency across experiments. Some notable products include:
- Thermo Fisher Scientific’s DreamTaq™ Buffer: This buffer is formulated for optimal results in PCR applications and aids in efficient primer annealing.
- New England Biolabs’ Phusion™ High-Fidelity Buffer: This buffer enhances thermal stability and ensures precise amplification, particularly in challenging templates.
- Promega's GoTaq® Reaction Buffer: Known for its versatility, it supports a range of applications while maintaining high specificity.
Benefits of using commercially available buffers:
- Convenience: Ready-to-use formats eliminate guesswork in preparation.
- Consistency: Standardized buffers minimize variability between experiments.
- Expert formulation: These buffers are optimized based on extensive research and user feedback, providing assurance of reliability.
Selecting the appropriate buffer can affect not only yield but also the specificity of the desired products. Therefore, it is worthwhile to evaluate options in relation to specific experimental needs.
Applications of DNA Annealing Buffers in Research
DNA annealing buffers serve as a cornerstone in many molecular biology applications. Their significance transcends simple laboratory practice; they are essential in achieving successful experimental outcomes in various research fields. Improper buffer conditions can lead to experiments failing, creating a barrier to effective research. Understanding the applications of DNA annealing buffers is crucial for researchers, educators, and students alike.
Polymerase Chain Reaction (PCR)
Polymerase chain reaction, commonly known as PCR, is one of the main applications of DNA annealing buffers. This technique amplifies specific DNA sequences, allowing for detailed analysis and experimentation. The annealing phase in PCR relies heavily on the buffer's composition. Optimal concentration of salts, such as magnesium chloride, and appropriate pH levels in these buffers are critical for binding primers to the target DNA sequence.
If the annealing temperature is too low, non-specific binding may occur, while too high may prevent successful binding. Therefore, selecting the right annealing buffer affects the specificity and yield of the PCR products. Additionally, using commercially available buffers can help in standardizing results across different laboratories, although optimizing one's own buffer may bring better specificity and sensitivity in certain cases.
Cloning Techniques
Cloning techniques utilize DNA annealing buffers in various protocols, primarily in the process of inserting DNA fragments into vectors. The proper use of annealing buffers facilitates the ligation of DNA ends, allowing for successful incorporation of foreign DNA into host organisms. Distinct phases in cloning, such as restriction enzyme digestion and ligation, significantly benefit from optimized buffer composition.
Moreover, the buffer conditions can influence the efficiency of cloning. It is crucial to ensure that the buffer composition promotes a high concentration of DNA inserts for improved ligation rates. Inadequate buffer conditions may result in low cloning efficiency, leading to wasted resources and time. Consequently, the application of specialized buffers can enhance the success rate of cloning experiments, making them more reliable.
Sequencing Applications
In sequencing, the role of DNA annealing buffers also cannot be understated. Both Sanger sequencing and next-generation sequencing techniques rely on these buffers during the amplification of target DNA. The key is the buffer's ability to stabilize single-stranded DNA and facilitate proper hybridization with sequencer primers. The choice of buffer directly impacts the fidelity and accuracy of the resulting sequence data.
Furthermore, in sequencing contexts, it is important to consider potential inhibitors that may arise from buffer components. Careful selection and optimization of the buffer can help mitigate such issues. Ultimately, choosing the right DNA annealing buffer not only ensures efficient amplification but also contributes to the reliability of the data obtained from sequencing experiments.
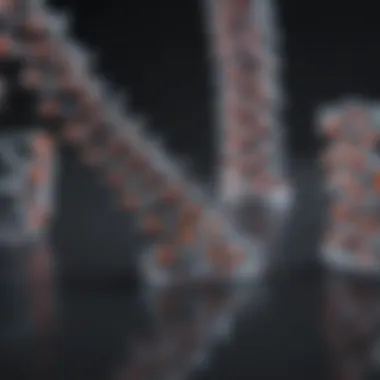
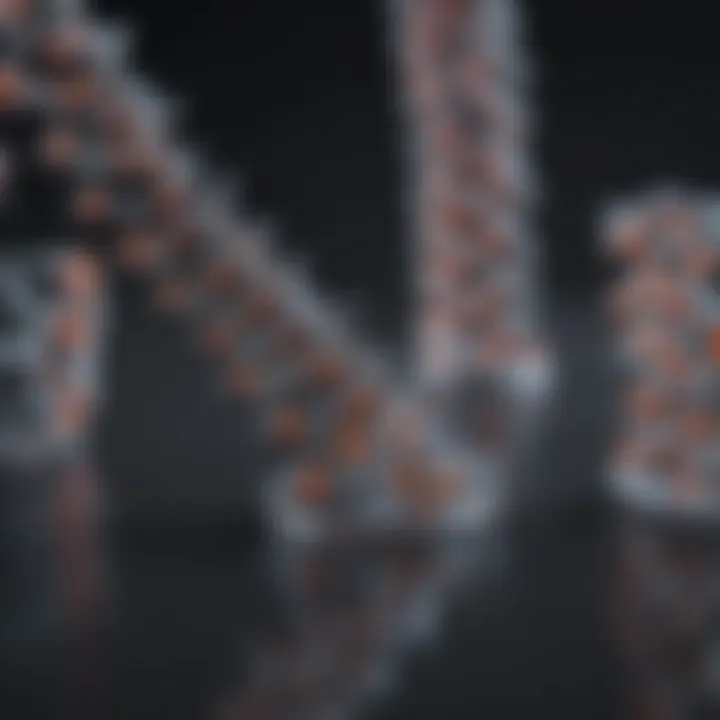
Understanding the specific needs of each application allows for the strategic selection of DNA annealing buffers, which can significantly enhance the robustness and accuracy of research outcomes.
Troubleshooting Common Issues
The process of DNA annealing can be intricately complex, often leading to challenges that impact results in molecular biology experiments. Understanding and addressing these common issues is crucial for optimizing outcomes. This section explores two notable problems: inefficient annealing and non-specific binding. Both require careful examination and adjustments in protocols to enhance the reliability of experimental results.
Inefficient Annealing
Inefficient annealing can significantly affect the success of nucleic acid hybridization. When DNA strands do not come together effectively, downstream applications can yield poor results. Several factors can contribute to inefficient annealing. These include the quality and concentration of the DNA templates, the specificity of the primers used, and the temperature conditions during the reaction.
To diagnose this issue, researchers should first evaluate the concentration of the DNA being used. If the amount is too low, it may lead to insufficient binding. Additionally, the purity of the DNA samples can play a critical role. Contaminants can hinder the hybridization efficiency.
Temperature is another significant factor. If the annealing temperature is too high, it can prevent proper pairing of the complementary strands. On the other hand, too low of a temperature may result in non-specific binding, contributing to background noise in the results.
Researchers may consider the following steps when troubleshooting inefficient annealing:
- Verify DNA Quality: Assess the integrity and purity of the DNA.
- Adjust Concentration: Increase the concentration of the DNA to ensure sufficient amounts are present for hybridization.
- Optimize Temperature: Employ a gradient PCR method to determine the best annealing temperature.
"Efficient DNA annealing is fundamental for successful molecular biology applications, affecting everything from PCR yield to sequencing accuracy."
Non-Specific Binding
Non-specific binding occurs when primers or probes attach to unintended regions of the DNA template. This can complicate interpretations of results, producing unexpected bands in gel electrophoresis or erroneous amplification in PCR assays. Such occurrences reduce the clarity and specificity of results, making it essential to identify and rectify this problem.
One common cause of non-specific binding is the sequence similarity between primers and non-target sequences in the template DNA. If there are regions of overlap, unintended binding can occur. Another contributing factor is the presence of contaminants that facilitate preferential binding to non-specific sites.
To minimize non-specific binding issues, several strategies can be implemented:
- Redesign Primers: Ensure that primers are unique to the target sequence. Using Tm calculators can help optimize the design.
- Increase Specificity: Introduce additives like DMSO or betaine, which can stabilize the correct hybridization while reducing non-specific interactions.
- Control Reaction Conditions: Fine-tune buffer composition and ionic strength, as these elements can impact binding specificity.
By approaching these troubleshooting strategies thoughtfully, researchers can improve their experimental designs and achieve more reliable data in their molecular biology studies.
Future Trends in DNA Annealing Buffers
The exploration of DNA annealing buffers is evolving rapidly. Future trends in this area will enhance research efficiency and accuracy. Understanding these trends is vital for researchers and students. Innovations in buffer technology focus on improving performance. Additionally, custom buffer solutions are gaining attention. These advancements may significantly alter protocols and improve outcomes in various applications.
Advancements in Buffer Technology
Buffer technologies have seen several advancements in recent years. Enhanced formulas aim to optimize DNA hybridization. One key focus is on buffer components that provide improved stability for longer experiments. Many researchers are developing buffers that resist degradation over time. For example, some researchers are using more stable salt concentrations. Salt concentrations that adapt to environmental shifts can lead to better results in experimental scenarios.
Moreover, researchers are looking into buffers with adjusted pH levels. These are designed for specific applications such as PCR. The increased specificity may lead to more reproducible results. With the introduction of high-throughput screening methods, buffer composition can be optimized quickly. These innovations highlight the importance of ongoing research into buffer formulations.
Potential for Custom Buffers
The shift towards custom buffers presents exciting prospects. Tailored solutions may meet the specific needs of experiments. Adaptability in buffer recipes allows for changes based on particular assays. For instance, custom buffers can be tailored for specific DNA sequences. This approach increases the success rates of hybridization. Furthermore, customization can address issues related to temperature and ionic strength.
The idea of allowing researchers to create their unique buffers opens avenues for innovation. In addition, researchers can assess their experiments with greater precision. This capability may lead to breakthroughs in fields such as synthetic biology. Embracing the potential of customized solutions will likely enhance research outcomes and drive collaboration among scientists.
Custom buffers represent an essential step towards optimizing experimental conditions and advancing molecular biology techniques.
Closure
The conclusion of this article is pivotal in synthesizing the knowledge presented while acknowledging the wide-ranging relevance of DNA annealing buffers in molecular biology. This section serves as a reflective summarization, highlighting how various aspects discussed contribute to the practical application and theoretical understanding of these buffers. It is essential to reinforce the idea that DNA annealing buffers are not merely supportive tools; they are fundamental components that influence experimental outcomes in molecular biology.
The benefits of understanding DNA annealing buffers include improved experiment design, enhanced efficiency in techniques like PCR and cloning, and the ability to troubleshoot common issues. By grasping the key elements about chemical composition, mechanisms, and preparation protocols, researchers can innovate and optimize their methodologies.
Summarizing Key Takeaways
Understanding the function and composition of DNA annealing buffers is crucial for any molecular biology work. Here are some key points to consider:
- Composition Matters: The specific salts and buffering agents used in these buffers play a significant role in their effectiveness.
- Annealing Temperature: Awareness of optimal annealing temperatures can drastically affect hybridization and subsequent results.
- Common Applications: These buffers are employed across various molecular biology techniques such as PCR, cloning, and DNA sequencing, influencing the success rate of experiments.
- Troubleshooting: Identifying issues like non-specific binding or inefficient annealing can help refine methods and enhance the overall quality of the research.
Encouraging Continued Research
The field of molecular biology is continuously evolving, and research into DNA annealing buffers is no exception. Further investigation into the development of novel buffers tailored for specific applications can lead to significant advancements.
Encouraging researchers to explore custom buffer formulations could yield valuable results, particularly in more complex experiments involving different DNA types or molecules. Future studies might also examine the impact of buffer components on long-term stability and performance, thus enabling better experimental planning and execution.