Neutron Activation Analysis: Principles and Applications
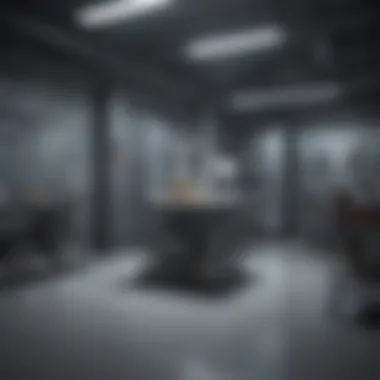
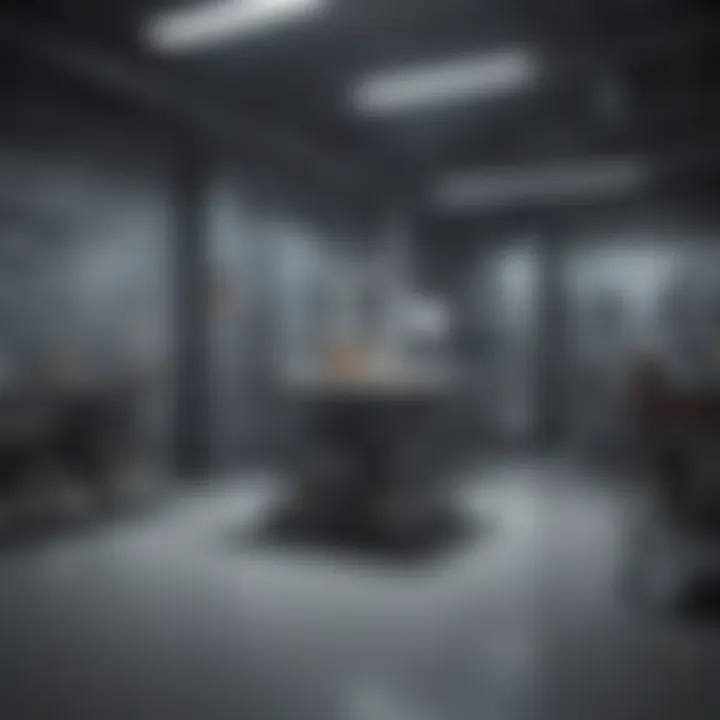
Intro
Neutron Activation Analysis (NAA) stands out as a highly sophisticated technique employed for measuring elemental concentrations in various materials. The beauty of NAA lies in its sensitivity, allowing researchers to identify trace elements that might be overlooked by other methods. As the scientific community grapples with complex challenges in fields such as archaeology, environmental science, and forensic analysis, understanding the principles and operations of NAA becomes increasingly indispensable.
The goal of this article is to provide a thorough insight into the inner workings of NAA, from its foundational concepts to real-world applications across diverse disciplines. This exploration will not only illuminate the method itself but also highlight its practical implications and the ongoing conversations about its evolution in research and industry.
NAA relies on neutron bombardment of samples, causing specific isotopes to become activated. These activated isotopes then emit gamma rays, which are characteristic of the element present. By measuring these emissions, scientists can ascertain the concentration of various elements within a sample. This concept of utilizing neutron-induced radioactivity to glean information has far-reaching consequences, paving the way for breakthroughs in numerous scientific inquiries.
Prelims to Neutron Activation Analysis
Understanding Neutron Activation Analysis (NAA) is like peeling an onion—each layer reveals more about its significant role in analytical technology. This method is not just another analytical procedure; it's a powerful tool in various scientific fields. By allowing researchers to identify and quantify trace elements with unparalleled precision, NAA finds its importance stretched across archaeology, environmental studies, and forensic investigations.
NAA operates on a principle as intriguing as it is efficient. When samples are exposed to neutrons, they become radioactive, emitting gamma rays that hold the key to elemental identification. This technique doesn’t just scratch the surface; it dives deep into the elemental composition of materials, thus providing insights that other techniques might miss. In talks among professionals, you might hear NAA referred to as a “silent workhorse” of analytical methods, a nod to its reliability and sensitivity.
In this section, we explore foundational concepts that inform the rest of the discussion, setting the stage for a more intricate understanding of its processes and applications.
Definition and Importance
Neutron Activation Analysis can be defined as a sensitive analytical procedure used for determining the concentrations of elements in various materials through their radiation characteristics post neutron bombardment. This process is critical as it allows for the detection of elements at very low concentrations, often in the parts per billion range. The method’s significance lies not merely in its quantitative capabilities but also in its qualitative finesse; it identifies multiple elements simultaneously without requiring extensive sample preparation.
To grasp why NAA is considered a standout choice for scientists, let’s highlight several of its vital importance:
- High Sensitivity: NAA excels in its ability to detect trace amounts of various elements, making it a go-to for environmental and geological samples where concentrations are typically low.
- Non-Destructive Testing: This is particularly crucial for historical artifacts or biological specimens where minimization of damage is a priority.
- Wide Applicability: From detecting illegal substances in forensic studies to analyzing pollutants in environmental samples, the versatility of NAA is noteworthy.
Each of these aspects underlines why NAA plays an indispensable role in modern analytical chemistry—not merely a tool but an essential part of the investigative process.
Historical Context
The story of Neutron Activation Analysis is akin to the unfolding of an intricate tapestry. Its threads intertwine through the history of nuclear science. This technique first emerged in the mid-20th century when scientists sought new ways to explore the mysteries of atomic interactions. The concept was initially introduced in the 1930s by researchers like Herbert Anderson and his team at the University of California, Los Angeles, who explored neutron physics and its applications.
Fast forward to the 1940s, when significant advancements in nuclear reactors brought about improved neutron sources, effectively enhancing the feasibility of NAA. This period saw NAA being applied to a variety of scientific inquiries, such as isotope research and tracking environmental changes—work that today seems fundamental but was groundbreaking at the time.
By the 1960s and 1970s, with the development of gamma spectroscopy techniques, NAA began to gain traction among researchers and established itself as a reliable, standardized method. The ability to analyze different materials with a single irradiation and detection process set NAA apart from contemporaneous techniques.
Today, as we stand on the shoulders of these scientific giants, the evolution of NAA continues, bringing with it discussions on its future and its role in pioneering research. Understanding its history not only enlightens us about its current applications but also highlights the ingenuity that drives scientific exploration—a journey that is as relevant now as it ever was.
Fundamental Principles of NAA
Neutron Activation Analysis (NAA) is a sophisticated technique resting on a set of fundamental principles that make it a vital player in the world of elemental analysis. By grasping these principles, one can appreciate NAA's unique capabilities—especially its sensitivity and multi-element detection. Understanding these core components is essential for anyone delving into this method, be it students, researchers, or professionals in related fields.
Neutron Sources
The integrity of neutron activation analysis heavily relies on the sources from which neutrons are derived. Various neutron sources can be employed, each with its own nuances and trade-offs. Commonly, reactors or particle accelerators are used.
- Nuclear Reactors: These are perhaps the most prevalent sources for neutron activation. Reactors generate neutrons through fission reactions, and they can produce a significant flux of neutrons, providing an ideal environment for effective activation of samples.
- Radioisotope Neutron Sources: Alternatives like californium-252 or americium-beryllium sources are compact and can be used in portable applications. They're particularly useful in fieldwork but might lack the neutron flux required for larger sample sets.
- Particle Accelerators: These are another route, generating neutrons via the collision of accelerated particles, such as protons, with target materials. While costly, they can provide very specific energy ranges for neutron production.
Each of these sources brings about distinct benefits suited for various applications. For instance, if precision and control over neutron energy are priorities, particle accelerators hold the advantage. On the flip side, nuclear reactors might be best for large-volume, high-throughput scenarios. This variety allows researchers to tailor their approach depending on their specific objectives.
Activation Process
Once the sample is exposed to neutrons, a series of interactions occur leading to activation. This step is where the magic of NAA truly unfolds. When neutrons collide with the nuclei of certain isotopes, they can be captured, forming unstable isotopes. This interaction kicks off the activation process.
- Neutron Capture: Primarily, neutrons interact with target nuclei, often resulting in an isotope's transformation. It’s a delicate exchange, influencing the elemental composition significantly.
- Decay of Activated Nuclei: After capturing, activated isotopes begin to decay back to stability, emitting gamma rays in the process. The timing of activation and subsequent decay can be crucial for ensuring accurate measurements.
- Elemental Specificity: Different elements emit distinct gamma rays, acting as fingerprints for identification. This elemental specificity lays the groundwork for analysis post-activation.
The activation process is quite intricate yet pivotal. Its efficiency determines the success of the subsequent analysis, dictating how well one can identify and quantify materials in a sample.
Gamma Ray Spectroscopy
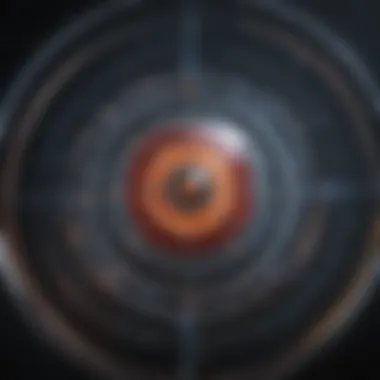
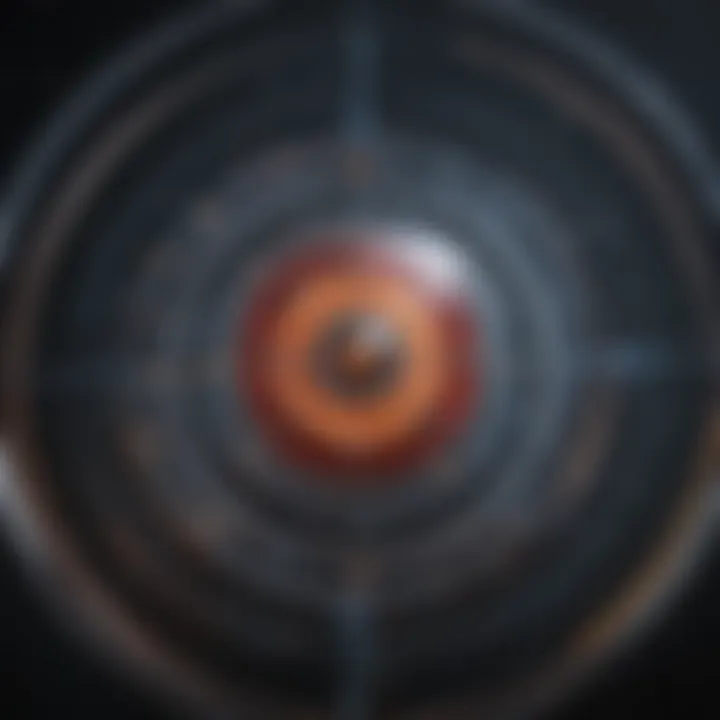
The next essential piece in this analytical puzzle is gamma ray spectroscopy. It allows researchers to decipher the complex data generated from the activated samples. Gamma rays emitted during decay carry invaluable information about the elements present in a sample.
- Spectroscopic Detection: Advanced detectors capture gamma emissions, translating them into spectral data for interpretation. Each peak in the spectrum corresponds to a specific elemental composition.
- Energy Resolution: The quality of detection relies heavily on the resolution capabilities of the spectroscopy equipment in use. High energy resolution allows for better differentiation between gamma-ray energies, leading to more accurate identification.
- Quantitative Analysis: By measuring the intensity of gamma emissions, one can deduce the concentration of elements within a sample. A well-calibrated system can transform a wealth of data into meaningful insights.
Gamma ray spectroscopy isn’t a mere add-on; it serves as the bridge connecting neutron activation to tangible analytical results. Its role underscores the importance of precision and accuracy in NAA methodologies.
"Understanding the principles of NAA is like holding a key to unlock the secrets held within various materials."
Through these principles, one can see why NAA remains a cornerstone in elemental analysis, providing capabilities that few other techniques can match.
Step-by-Step Process of Neutron Activation Analysis
In the realm of elemental analysis, the step-by-step process of Neutron Activation Analysis (NAA) is akin to following a detailed recipe. Each step is critical, ensuring the integrity and accuracy of the results. Understanding this process is pivotal, as it not only illuminates the methodology behind NAA but also underscores its significant impact across various scientific fields.
Sample Preparation
Sample preparation is where the journey begins. This phase is crucial because the quality of the sample directly influences the reliability of the analysis. It often involves several meticulous steps:
- Selection: The material that will be analyzed must be carefully chosen. It could range from a simple piece of soil to complex biological tissues.
- Handling and Cleanliness: Contamination must be avoided at all costs. This means using clean containers and possibly even gloves to prevent residue from interfering with the outcome.
- Sizing: Samples are generally reduced to a smaller, uniform size to ensure even exposure to neutrons during irradiation. Homogeneity is essential; if one part of the sample is denser or larger than another, it may yield misleading results.
It’s important to note that proper sample preparation can make or break the analysis. As the saying goes, "a chain is only as strong as its weakest link," and in NAA, the sample is that crucial link.
Neutron Irradiation
Once samples are prepared, the next stage is neutron irradiation. In this phase, samples are subjected to a neutron source, such as a nuclear reactor or a neutron generator. Here, the magic happens:
- Activation: When certain isotopes within the sample capture neutrons, they become unstable and emit gamma rays. The specific isotopes activated depend on the neutrons' energy levels and the nature of the materials.
- Timing: The duration of irradiation must be carefully calibrated. Too short a time may not activate enough elements, while excessive irradiation can lead to unnecessary complications, such as unwanted nuclear reactions.
- Safety Measures: Given the radioactive nature of this process, strict protocols must be observed. This entails proper shielding and monitoring to protect personnel and the surrounding environment.
“Safety isn’t just a practice; it’s a culture,” reminds every scientist involved in neutron activation analysis.
Counting and Analysis
The final phase in this step-by-step procedure is known as counting and analysis. This is where raw data becomes meaningful information:
- Gamma Ray Spectroscopy: Specialized detectors are employed to capture and analyze the gamma rays emitted during activation. Each element produces a unique spectrum, allowing scientists to ascertain its presence and concentration.
- Data Processing: The raw data collected needs to be interpreted. This involves calibration against standards and applying statistical methods to identify elemental quantities with high precision.
- Reporting Results: Finally, the findings are compiled into reports that communicate the elemental makeup of the samples. Clarity in reporting is essential to ensure that results can be replicated and understood by others in the field.
Applications of Neutron Activation Analysis
Neutron Activation Analysis (NAA) stands out as an essential tool in various fields, owing largely to its precision and sensitivity. This technique, honed over decades, has found its niche in a multitude of applications that extend from uncovering the past in archaeology to monitoring our environment and solving complex forensic puzzles. By harnessing the principles of nuclear interactions, NAA can furnish researchers and professionals with crucial information that may not be easily observable through other methods. Here, we delve into the significant applications of NAA, emphasizing its relevance and benefits across diverse domains.
Archaeological Research
In the realm of archaeology, neutron activation analysis acts as a time machine of sorts, allowing scientists to study artifacts and materials with unparalleled depth. One of the standout uses is in the analysis of trace elements within pottery, minerals, and metals. By assessing the elemental composition, researchers can not only determine the origin of these materials but also draw conclusions about trade routes and cultural exchanges that existed centuries ago. For instance, archaeologists have successfully used NAA to analyze ancient clay pots, revealing that they were crafted from clay sourced hundreds of miles away, indicating sophisticated trade networks.
To amplify the value of NAA in archaeology, consider its non-destructive nature. Unlike some techniques that may damage artifacts, NAA allows for precise analysis without compromising the integrity of historical items. This gentle approach makes it a favored choice for museums and research institutions, preserving artifacts for future generations while unearthing their untold stories.
Environmental Monitoring
The environmental field has embraced neutron activation analysis as a key player in monitoring pollutants and assessing ecosystem health. Researchers can deploy NAA to detect trace elements in soil, water, and air samples, where levels of heavy metals or other hazardous substances can be quantified. This capability is essential for environmental protection agencies and organizations focused on public health.
For instance, by determining the concentration of mercury in fish populations, scientists can alert communities about potential health risks linked to fish consumption from contaminated waters. Furthermore, NAA's ability to analyze particulates in air samples aids in tracking pollution sources, thereby guiding regulations and remediation efforts.
Another commendable aspect is how NAA can engage in multi-element analysis. This means that with a single irradiation, a researcher can identify numerous elements present in a sample, allowing for a comprehensive overview of the environmental state. As a result, it delivers both efficiency and extensive data, essential for informed decision-making regarding environmental policies.
Clinical and Forensic Analysis
In clinical laboratories and forensic environments, neutron activation analysis offers a scientific edge. In medicine, it is employed to measure trace elements in biological samples such as blood and urine. These measurements can provide predictive markers for various health conditions. Deficiencies or excesses of trace elements can be critical for diagnosing diseases, emphasizing how NAA can contribute to personalized medicine strategies.
In forensic science, the application of NAA can be likened to an investigative toolkit for detectives. It can identify elements in seized materials, linking suspects to crime scenes through residue analysis. For example, NAA has been utilized for gunshot residue analysis, helping to establish whether an individual discharged a firearm. Moreover, it fits well into the modern approach of using multi-dimensional methods for forensic investigations, complementing other analytical techniques to build a holistic view of the evidence.
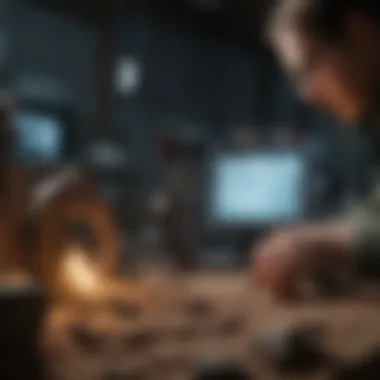
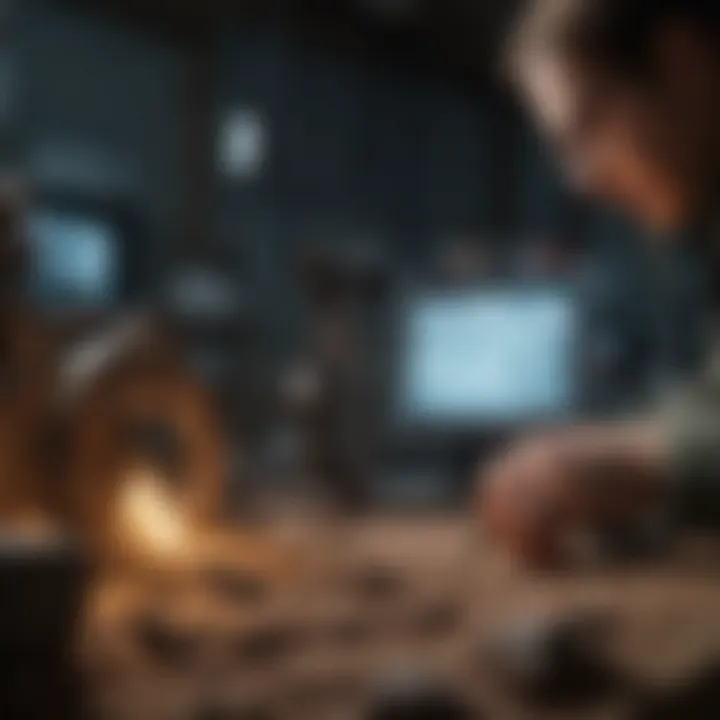
NAA proves vital across fields, bridging gaps between disciplines through its versatile applications.
Advantages of Neutron Activation Analysis
Neutron Activation Analysis (NAA) stands out as a premier analytical method, prized for its ability to deliver precise information about elemental concentrations across a spectrum of materials. Understanding the advantages of NAA is vital, especially for those who engage in scientific research and various industrial applications.
High Sensitivity and Precision
One of the hallmark features of NAA is its remarkable sensitivity. This technique allows for the identification and quantification of elements at trace levels, often in the parts per billion (ppb) range. This capacity makes it particularly advantageous in fields like environmental monitoring, where pollutants may exist in low concentrations that are nonetheless crucial for risk assessments. Furthermore, the precision of NAA derived from its reliance on well-defined nuclear interactions ensures that the obtained measurements are both accurate and reproducible.
"The sensitivity of NAA enables scientists to detect elements they might not even consider, making it a crucial tool in uncovering hidden traces of materials in samples – a small wonder in a big world."
Moreover, NAA benefits from a low detection limit for nearly all elements in the periodic table. The methodology utilized in this analysis—where fast neutrons interact with the nuclei of the sample—further enhances accuracy by minimizing the influence of physical or chemical forms of the sample on the final results. This means that scientists can trust their measurements without the nagging suspicion of unyielded errors, especially when conducting comparative studies.
Multi-Element Analysis Capability
Another significant advantage of NAA is its ability to analyze multiple elements simultaneously. Unlike many traditional methods that focus on a single element at a time, NAA can provide a comprehensive elemental profile from a single irradiation event. This characteristic is particularly beneficial in applications that demand a holistic understanding of materials, such as in archaeology when assessing artifacts or in environmental science during ecological studies.
This multi-element capability stems from the diverse energy levels at which each activated isotope emits gamma rays. By employing gamma ray spectroscopy in conjunction with NAA, researchers can distinguish between the signatures of various elements, effectively building a complete elemental fingerprint from one sample.
The ability to conduct analyses of multiple elements concurrently not only saves time but also reduces the total costs associated with sample preparation and handling. The efficiency gains realized through such a multipronged approach are indispensable, particularly when one considers the resources that modern research often demands.
In summary, the advantages of Neutron Activation Analysis—its high sensitivity, precision, and capacity for multi-element analysis—render it a powerhouse in analytical chemistry, fortifying its position as a preferred method among researchers and practitioners in diverse fields. With ongoing advancements, the relevance of NAA is set to further expand, paving the way for new discoveries and insights.
Limitations of Neutron Activation Analysis
Understanding the limitations of Neutron Activation Analysis (NAA) is crucial for those who are considering its application in various fields. While NAA offers impressive sensitivity and multi-element capabilities, it's not without its caveats. Recognizing these limitations is essential for researchers and professionals to make informed decisions regarding their methodologies.
Equipment Requirements
One of the more significant hurdles when utilizing NAA is the equipment requirements. This process relies heavily on specialized tools, including neutron sources, gamma-ray spectrometers, and other auxiliary devices. Let’s highlight some key points here:
- Neutron Source: A nuclear reactor or a neutron generator is necessary for the activation process, and these do not come cheap. Operating such facilities requires adherence to stringent regulatory frameworks, which can complicate access.
- Shielding Needs: Due to the radiation involved, adequate shielding is required, which adds to the overall costs and complexity of setup. Facilities that host NAA typically need reinforced structures to protect personnel and equipment from radiation exposure.
- Calibration and Maintenance: Equipment for NAA must be regularly calibrated and maintained. This involves both time and money which can pose challenges especially for institutions with limited budgets.
Overall, the initial investment in NAA equipment can be considerable and may deter potential users, particularly in smaller laboratories or institutions.
Potential Interferences
Another critical consideration involves potential interferences that can affect the accuracy and reliability of NAA results. This can include a range of elements that may skew analytical outcomes:
- Matrix Effects: The composition of the sample matrix can influence the neutron absorption and activation efficiency. Elements present in high concentrations may interfere during the detection step, making it tricky to assess the concentration of target elements accurately.
- Nuclear Isomerism: Some isotopes may have long-lived metastable states, which can complicate the interpretation of the spectral data. Researchers might find themselves in a conundrum trying to differentiate between signals generated from various isotopes.
- Environmental Factors: Variability in environmental conditions such as temperature and humidity during sample handling might also introduce errors. If samples are not controlled properly, the activation and detection processes may yield inconsistent results.
Comparison with Alternative Analytical Techniques
In the realm of elemental analysis, Neutron Activation Analysis (NAA) stands as a potent tool, yet it doesn't exist in isolation. To fully appreciate its capabilities and limitations, it is imperative to compare it with alternative techniques. This section delves into the characteristics, benefits, and drawbacks of two prominent methods: X-ray Fluorescence (XRF) and Inductively Coupled Plasma Mass Spectrometry (ICP-MS).
X-ray Fluorescence (XRF)
X-ray Fluorescence, or XRF, is a non-destructive technique widely employed for elemental analysis. It involves irradiating a sample with X-rays, causing the sample to emit secondary (or fluorescent) X-rays characteristic of the elements present. Unlike NAA, which requires neutron sources and nuclear reactions, XRF can be performed with simpler equipment. This factor can be a game changer for labs looking to save on operational costs.
Moreover, XRF is incredibly fast. Sample analysis is usually completed in mere minutes, offering quick turnaround times for urgent analyses. Its capacity to analyze solids, liquids, and even powders makes it exceptionally versatile. However, XRF has its shortcomings. One significant limitation is its sensitivity to certain elements. For instance, heavier elements tend to be detected more efficiently than lighter ones, potentially skewing results depending on the nature of the sample.
- Pros of XRF:
- Cons of XRF:
- Non-destructive nature
- Rapid analysis
- Versatile across different states of matter
- Sensitivity varies across elements
- May require sophisticated calibration to ensure accuracy
Inductively Coupled Plasma Mass Spectrometry (ICP-MS)
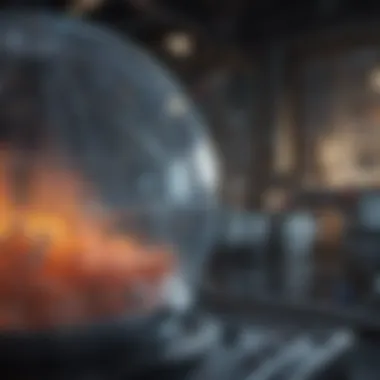
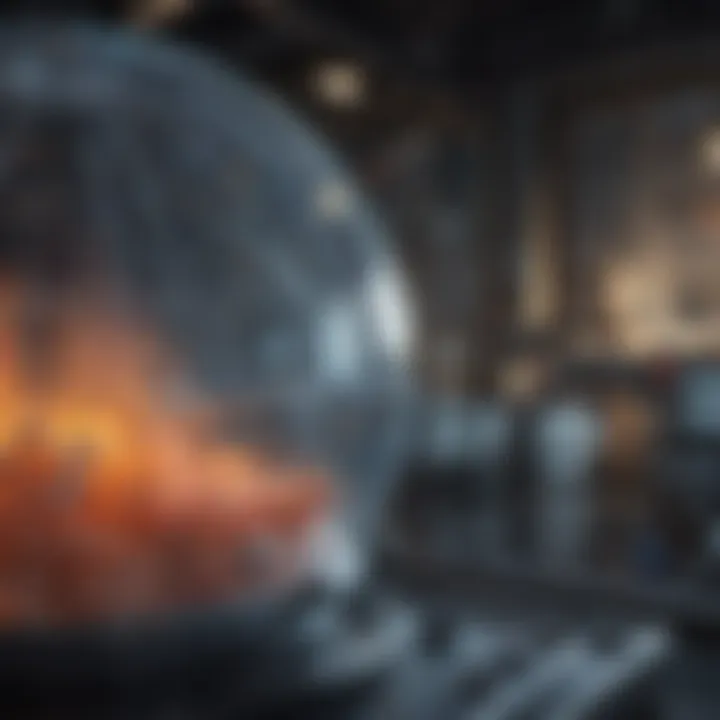
Inductively Coupled Plasma Mass Spectrometry (ICP-MS) is another heavyweight contender in the world of analytical techniques. It employs a high-temperature plasma to generate ions, which are then detected and analyzed. The key allure of ICP-MS lies in its extraordinary sensitivity and capacity for multi-element analysis. NAA might have multi-element capabilities, but in terms of sheer sensitivity, ICP-MS often leads the pack, detecting elements at parts per trillion.
That said, the equipment needed for ICP-MS is typically more complex and costly than that for XRF and even NAA. Moreover, the preparation of samples usually involves digestion processes that can complicate operations. Unlike NAA, ICP-MS can experience matrix effects, where the composition of the sample can significantly influence the readings, leading to potential inaccuracies if not properly accounted for.
- Pros of ICP-MS:
- Cons of ICP-MS:
- Unmatched sensitivity, able to detect low abundances
- Capability for analyzing numerous elements simultaneously
- High initial equipment and operational costs
- Requires intricate preparation of samples
Comparing NAA with these two analytical methods reveals important considerations for researchers and professionals. Each technique brings unique strengths to the table while also harboring limitations that may impact decision-making based on project requirements. As technology advances, the interplay between NAA, XRF, and ICP-MS may evolve, potentially leading to innovations that harness the best of each method.
"Understanding the nuances between various analytical techniques paves the way for more informed decisions in research and industry."
Thus, as professionals in the field navigate their choices, a clear grasp of how these methods differ—and complement—each other is invaluable for maximizing analytical efficiencies.
Future Trends in Neutron Activation Analysis
The landscape of Neutron Activation Analysis (NAA) is constantly evolving, promising bright opportunities and challenges ahead. Understanding the future trends in this analytical technique is crucial, not only for keeping pace with scientific innovations but also for recognizing its burgeoning potential across various sectors. This section aims to shed light on the technological advancements that are reshaping NAA and the expanding applications that highlight its versatility in addressing modern scientific questions.
Technological Advancements
When we think about the future of NAA, a significant part of the conversation revolves around technological advancements. These changes enhance our ability to perform analyses with increased efficiency and reliability. For instance, improvements in neutron source technology, such as the development of compact neutron generators, offer safer and more accessible alternatives to traditional nuclear reactors. These generators can provide on-site testing capabilities, thus saving both time and resources for researchers.
Moreover, advancements in gamma-ray spectroscopy detectors have been astonishing. Contemporary high-purity germanium (HPGe) detectors are becoming more sensitive and faster, enabling the detection of trace elements in a variety of samples. This high level of precision ensures that even the smallest concentrations can be measured with great accuracy, which is particularly beneficial in disciplines like archaeology and forensic science.
Key benefits of these technological improvements include:
- Enhanced sensitivity: Modern devices can detect lower concentrations of elements than previously achievable.
- Faster analysis times: Rapid response times mean that research cycles can be shortened, leading to quicker conclusions.
- Increased portability: Smaller equipment can be transported easily, making NAA accessible in various locations.
"The advent of advanced detector technology not only boosts measurement sensitivity but also allows for real-time data analysis, a game changer in many fields of applications."
These advancements in technology are essential, as they not only make NAA more efficient but also enable researchers to tackle issues that were once thought too complex or infeasible.
Expanding Applications
As NAA becomes more refined, its applications also continue to expand. One notable area gaining traction is environmental monitoring. With growing concerns about pollution and environmental sustainability, NAA’s ability to provide precise elemental analysis makes it a valuable tool for assessing soil, water, and air quality. For example, researchers can analyze sediment samples to trace heavy metals back to specific pollution sources, providing crucial information for remediation efforts.
In the field of medicine, NAA has shown promises in clinical research, particularly in studying trace elements in human health. It enables the identification of deficiencies or toxicities of various elements which can lead to better understanding and treatment of diseases. The capability to analyze biological samples with high sensitivity offers a golden opportunity in personalized medicine.
Applications are expanding into several fields, including:
- Nutritional studies: Understanding micronutrient status in populations.
- Historic preservation: Analyzing artifacts without damaging them helps in conservation efforts.
- Food safety: Determining elemental composition of food products to ensure consumer safety.
Overall, the evolving capabilities of NAA are opening doors to innovative applications. As this analytical method progressively integrates with fields as diverse as environmental science and public health, researchers are looking at a future where NAA could significantly influence how we understand and interact with our world.
End
Neutron Activation Analysis (NAA) stands out as a pivotal analytical technique that provides numerous benefits across a multitude of disciplines. Its robustness in measuring elemental concentrations enables researchers to unearth valuable insights that would be otherwise elusive with traditional methods. This concluding section recaps some critical elements and examines the implications of NAA for future research and industry applications.
Summary of Key Insights
In summary, Neutron Activation Analysis is not just a method; it's a window into the microscopic world of elemental composition. It allows for:
- High Sensitivity: NAA can detect parts per billion levels of various elements, making it invaluable for trace analysis.
- Multi-Element Capability: A single irradiation process can yield data on multiple elements simultaneously, minimizing sample processing time.
- Versatility Across Fields: From archaeology to the environmental sciences, its applicability spans various sectors, offering comprehensive analytical capability.
Through this article, we've covered the principles of neutron interaction, the detailed process of NAA, its wide-ranging applications, and the advantages that make it a go-to method. Moreover, the identified limitations and comparative techniques serve to contextualize NAA's position in the broader field of analytical sciences.
Implications for Research and Industry
The implications of NAA extend beyond the laboratory. As the technique continues to evolve, future advancements promise to refine its application further. Key implications include:
- Enhanced Research Quality: In academics and industry, NAA's robust data generation supports more accurate conclusions, fostering trust in scientific studies.
- Innovation in Environmental Monitoring: With ongoing global environmental challenges, NAA can assist in assessing pollutant levels and tracking their origins, enabling better policy-making and remediation efforts.
- Interdisciplinary Collaboration: Its diverse application encourages collaboration among fields, enriching research and combining expertise to solve complex problems.
In essence, NAA furthers the pursuit of knowledge across boht research and industrial landscapes. The continuous adaptation and advancement of NAA in response to growing analytical demands signal a bright trajectory, promising a deeper understanding of matter on a microscopic scale.