Nanonics Imaging: Innovations and Impact on Science
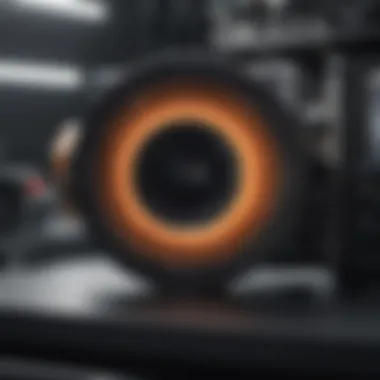
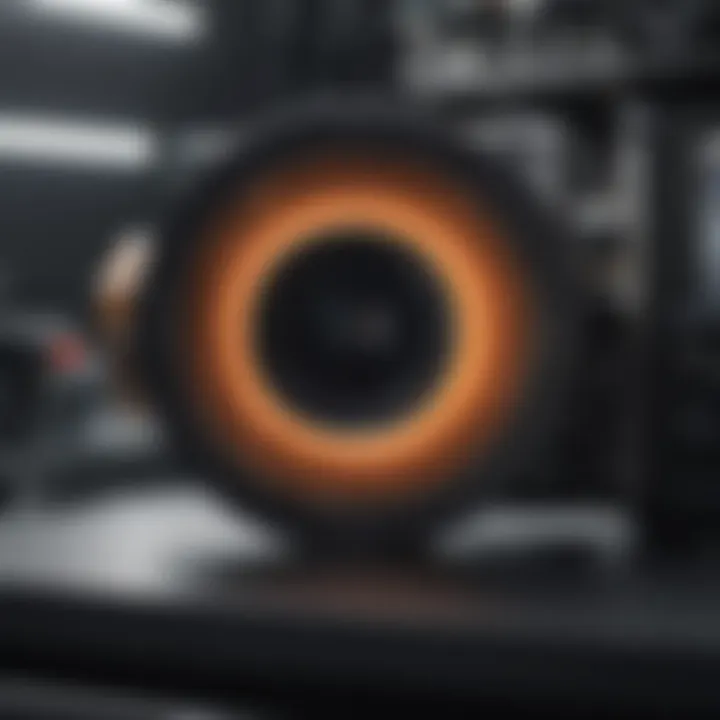
Intro
Nanonics imaging stands at the intersection of advanced technology and intricate scientific inquiry. As we explore this evolving field, it is crucial to consider how technological advancements enhance our ability to understand materials and biological organisms at the nanoscale. Modern imaging techniques provide researchers with the tools needed to engage with the finer details of matter, revealing insights that were once beyond reach. The relevance of this area lies not only in its scientific applications but also in its potential to transform industries.
Nanonics imaging encompasses a variety of methods that allow for heightened resolution and contrast. These methods are particularly valuable in scientific research, where minute details can be pivotal. By focusing on the mechanisms that underpin these imaging technologies, we can better understand their implications for fields such as biology, material science, and nanotechnology.
Understanding the challenges, benefits, and future directions of nanonics imaging helps clarify its role in advancing scientific knowledge. As such, the following sections will delve into the methodology employed in this field, upcoming trends, and areas that require further investigation.
Prolusion to Nanonics Imaging
Nanonics imaging represents a significant leap in the field of microscopy, offering unprecedented detail and resolution at the nanoscale. This introduction attempts to frame this complex subject within its broader scientific context by breaking down its essential components. As we explore these elements, it becomes apparent that understanding nanonics imaging is crucial for anyone engaged in research and development in fields such as materials science, biology, and nanotechnology.
Defining Nanonics Imaging
Nanonics imaging refers to a set of imaging techniques that allow for the visualization of structures and materials at an extremely small scaleโtypically at the nanoscale level. This involves methods which enhance the resolution beyond traditional optical imaging limits. Key technologies used in nanonics imaging include scanning probe microscopy, electron microscopy, and atomic force microscopy. Each of these techniques plays an important role, contributing to the ability to observe and analyze materials with high precision.
Nanonics imaging is not just about achieving high resolution; it also encompasses various contrast enhancement methods that bring out specific features of the samples being studied. By fine-tuning parameters such as illumination and detection, researchers can extract valuable information about the structural and chemical properties of materials. This enhanced understanding is vital for advancing scientific knowledge and fostering innovations across disciplines.
Historical Context
The concept of imaging at the nanoscale has evolved significantly since the advent of microscopy. Early techniques allowed scientists to observe objects at larger scales but struggled with details at the atomic level. The development of electron microscopy in the 1930s was a crucial milestone, enabling researchers to visualize cellular structures with much greater clarity. Over the decades, successive advancements, including the refinement of scanning tunneling and atomic force microscopy, brought nanonics imaging closer to reality. Today, these developments culminate in sophisticated platforms that integrate multiple techniques, paving the way for comprehensive imaging solutions.
The historical trajectory highlights not only technological advancements but also shifts in scientific focus, where understanding systems at the nanoscale become increasingly critical. This historical backdrop sets the stage for the transformative impact that nanonics imaging has on various fields.
Importance in Modern Science
In contemporary research, nanonics imaging plays an indispensable role. Its applications extend far beyond mere visualization; they underpin major breakthroughs in nanotechnology, materials science, and biomedical research. For example, in materials science, the ability to characterize nanostructures leads to the development of new materials with enhanced properties.
In biomedical applications, nanoscale imaging allows for detailed cellular observations, which is vital for drug delivery studies and understanding disease mechanisms.
"Nanonics imaging not only provides insights into the structure of materials but also aids in deciphering their functions and interactions."
In addressing complex scientific challenges, nanonics imaging bridges the gap between theoretical concepts and practical applications. As various industries seek to innovate and improve their processes, the importance of nanonics imaging cannot be overstated. Its multifaceted nature significantly enhances our understanding of both natural and engineered systems, offering insights that drive scientific advancement and industrial progress.
Fundamental Principles of Nanonics Imaging
The field of nanonics imaging relies heavily on its fundamental principles, as these techniques and methodologies lay the groundwork for exploring the nanoscale world. Understanding these principles is crucial for the advancement of technology and applications, ensuring precise imaging and analysis. The importance of these foundational elements cannot be overstated. They enable scientists and researchers to push the boundaries of what is observable and measurable at the nanoscale. Looking into these principles will demonstrate their relevance across various scientific disciplines, including biology and materials science.
Nanoscale Resolution Techniques
Nanoscale resolution techniques are pivotal in achieving high fidelity in imaging at the atomic and molecular levels. These techniques include various methodologies such as scanning tunneling microscopy (STM) and scanning electron microscopy (SEM).
- Scanning Tunneling Microscopy (STM) operates by exploiting the quantum tunneling effect. This technique allows for the visualization of surfaces at the atomic level by measuring the tunneling current between a sharp tip and the sample.
- Scanning Electron Microscopy (SEM) uses focused beams of electrons to create detailed images. It excels in surface topology visualization, providing three-dimensional images with exceptional resolution.
These techniques are essential as they provide unparalleled detail that conventional imaging methods cannot achieve, thus allowing scientists to study the properties and behaviors of materials and biological structures in finer detail than was previously possible.
Detection Methods
The efficiency of nanonics imaging is largely dependent on the detection methods employed. Key detection technologies include photodetectors and charge-coupled devices (CCDs). These methods play a significant role in converting qualitative data into quantitative information.
- Photodetectors offer sensitivity across various wavelengths. They are crucial for applications that require light detection, allowing nanonics imaging to gather data from ultraviolet to infrared spectra.
- Charge-Coupled Devices (CCDs) have become standard in capturing high-resolution images in many microscopy techniques. Their ability to convert photons into electronic signals makes them invaluable in achieving accurate imaging results.
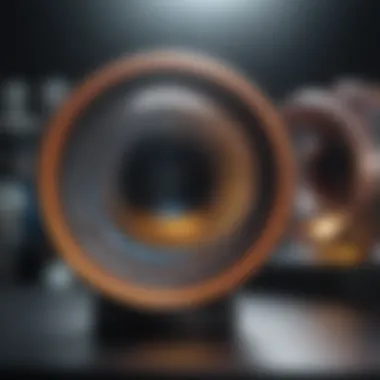
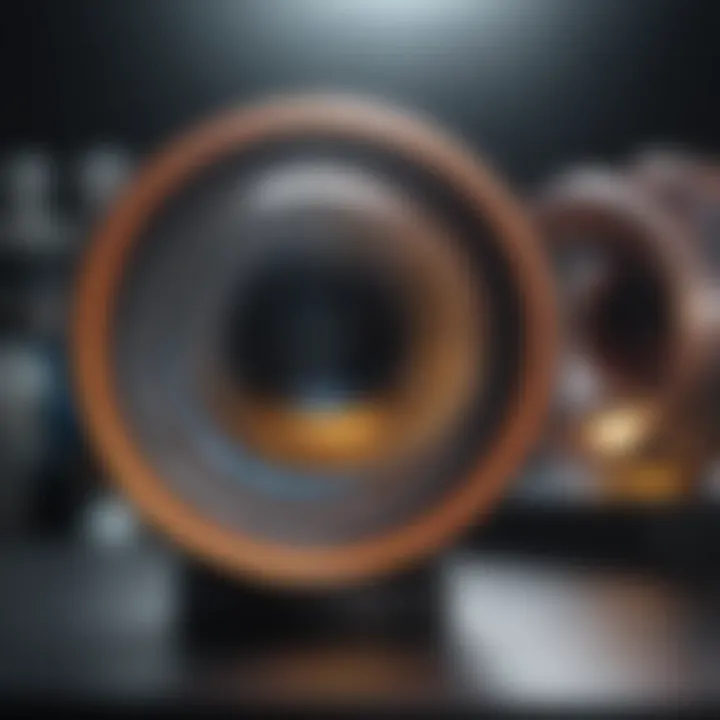
Combined, these methods enhance the ability to observe and measure the nanoscale features and dynamics of different materials.
Contrast Enhancement Techniques
Contrast enhancement is vital for improving the visibility of features within nanoscale images. Various techniques are employed to enhance image quality, thus making analysis more reliable. These techniques include phase contrast microscopy and fluorescence imaging.
- Phase Contrast Microscopy emphasizes differences in refractive index, making it easier to distinguish between various structures without needing to stain samples. This technique is particularly valuable in biological applications, allowing for the observation of live cells.
- Fluorescence Imaging utilizes specific wavelengths of light to excite fluorescent probes attached to the target samples. This method significantly enhances visibility, enabling the study of specific components within complex mixtures, such as cellular organelles.
Techniques like these not only improve the clarity of images but also facilitate more profound insights into the fundamental properties of biological and synthetic structures at the nanoscale.
"The accuracy in nanoscale imaging significantly contributes to the advancements in research fields, making it pivotal for new discoveries."
Overall, understanding and implementing these fundamental principles in nanonics imaging form the backbone of modern scientific inquiry, pushing the limits of what can be visualized and analyzed in various domains, from materials science to biomedical applications.
Nanonics Imaging Technologies
Nanonics imaging technologies play a crucial role in the advancement of scientific research and industrial applications. They enable scientists to visualize structures at the nanoscale, offering insights into materials and biological systems that were previously unattainable. The ability to observe matter at such minute dimensions opens avenues for innovation in various fields, including biomedical research, materials science, and environmental studies.
These technologies enhance resolution and contrast, which are key for accurate interpretation of nanoscale phenomena. Each imaging method offers unique advantages and challenges, making it essential to choose the appropriate technique based on the specific requirements of the study. This section will explore three prominent nanonics imaging technologies: Scanning Probe Microscopy, Electron Microscopy, and Atomic Force Microscopy.
Scanning Probe Microscopy
Scanning Probe Microscopy (SPM) includes a variety of techniques, such as Scanning Tunneling Microscopy (STM) and Atomic Force Microscopy (AFM). SPM allows for imaging, measuring, and manipulating matter at the nanoscale with high resolution.
One notable benefit of SPM is its ability to provide three-dimensional imaging of surfaces. This characteristic is crucial for applications in materials science, as it allows researchers to study the topography and properties of materials in their natural state. Furthermore, SPM can function in various environments, including air, vacuum, or liquid, which expands its usability in biological applications.
Despite its advantages, SPM does face some limitations. Instrument sensitivity can vary, potentially leading to inconsistent results. Additionally, sample preparation can be demanding, as samples must often be flat and stable to ensure accurate measurements.
Electron Microscopy
Electron Microscopy (EM) employs a beam of electrons to illuminate a sample, providing detailed images at high magnifications, sometimes exceeding a million times. This technology is vital for observing the internal structure of materials and the morphology of biological specimens at atomic resolution.
The major advantage of Electron Microscopy is its unparalleled resolution compared to optical methods. For instance, Transmission Electron Microscopy (TEM) allows for imaging at the atomic scale, making it indispensable for nanomaterials characterization. However, high operational costs and the requirement for significant sample preparation can hinder its accessibility for some researchers.
Atomic Force Microscopy
Atomic Force Microscopy (AFM), a subset of scanning probe microscopy, utilizes a cantilever with a sharp tip to scan the surface of a sample. As the tip moves over the surface, interactions between the tip and the surface allow for precise imaging and measurement. AFM provides not only topographical maps but also mechanical properties, such as stiffness and adhesion forces.
AFM's strengths lie in its versatility. It can be used on a diverse array of samples, from biological specimens to polymers and metals. It operates in various environments, allowing for real-time observation of dynamic processes. However, like SPM, sample preparation remains a challenge, and maintaining tip cleanliness is critical for obtaining high-quality data.
Applications of Nanonics Imaging
The applications of nanonics imaging play a crucial role in advancing various scientific fields. Understanding how these imaging techniques translate into practical uses is essential for grasping their overall significance.
Biomedical Applications
Cellular Imaging
Cellular imaging using nanonics techniques has transformed the way researchers study biological cells. The ability to visualize cells at nanoscale resolution allows for detailed analysis of cellular structures and functions. This precision helps scientists understand cell behavior in healthy and diseased states. A key characteristic of cellular imaging is its spatial resolution, which enables the observation of fine cellular details, such as organelles and receptors.
This imaging approach is especially beneficial for studying diseases like cancer, where understanding tumor microenvironments is crucial. The unique feature of cellular imaging lies in its combination of high resolution and molecular specificity, allowing for the observation of specific cellular components. While the advantages are clear, such as enhanced understanding of cell dynamics, challenges remain, including complex sample preparation and the need for expensive equipment.
Drug Delivery Studies
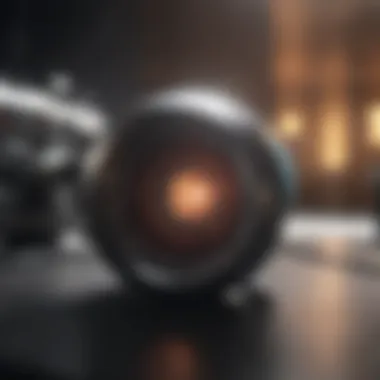
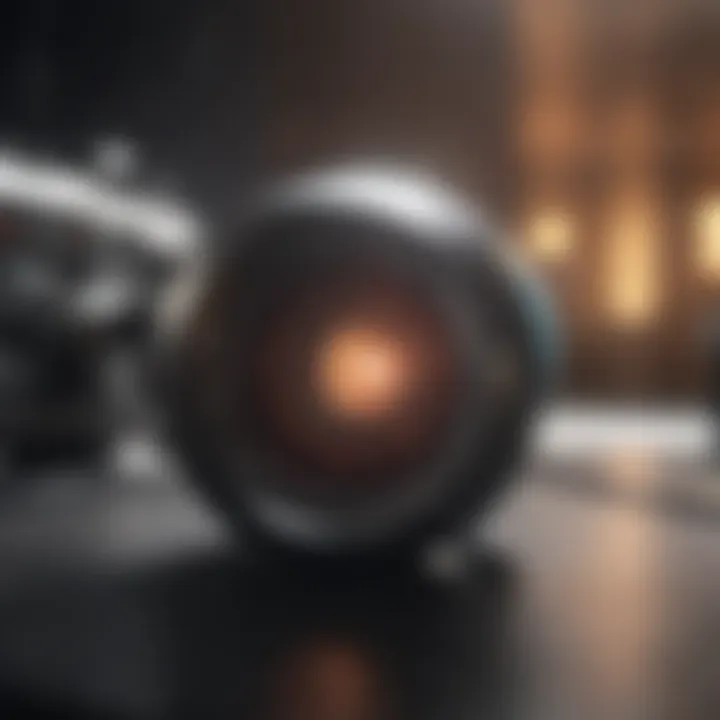
Drug delivery studies that utilize nanonics imaging techniques offer insights into the mechanisms of drug action and distribution within biological systems. By visualizing how drugs interact with cellular components, researchers can develop more effective therapies. A significant advantage of using nanonics imaging is the ability to study drug transport at a nanoscale level, revealing details about drug release and uptake in real-time.
This method is popular among pharmaceutical researchers because it helps in refining drug formulations and improving targeted delivery systems. The unique feature here is the real-time tracking capability, which allows scientists to monitor drug behavior in vivo effectively. However, the intricacies involved in designing imaging setups may pose challenges and can limit wider application.
Materials Science Applications
Nano-structuring Materials
In materials science, nanonics imaging plays an essential role in the nano-structuring of materials. The methods allow scientists to visualize and manipulate materials at the nanoscale, facilitating the development of advanced materials with tailored properties. A key aspect is the ability to achieve nanoscale precision in structuring, which is beneficial for creating materials with specific functionalities.
Researchers frequently employ these imaging techniques to produce nanostructured surfaces, enhancing properties like strength, conductivity, and reactivity. The unique feature of this application is its potential for customization, which can lead to innovative material solutions. Despite the benefits, the processes involved can be costly and complex, which can hinder broader adoption in industry.
Characterization of Nanomaterials
Characterization of nanomaterials using nanonics imaging methods is crucial for understanding their properties and behavior. This aspect of nanonics imaging focuses on identifying the structural, chemical, and physical attributes of nanomaterials. The key characteristic of these methods is the comprehensive analysis they provide, enabling researchers to conclude the suitability of nanomaterials for specific applications.
This characterization is critical in developing nanomaterials used in electronics, medicine, and catalysis. The unique feature is the high level of detail that allows scientists to observe defects or impurities within the material. However, challenges include standardizing measurement techniques and interpreting complex data sets that can be cumbersome.
Environmental Monitoring
Pollution Tracking
Pollution tracking through nanonics imaging techniques has become increasingly vital in environmental science. These methods enable scientists to monitor pollutants at the nanoscale, leading to better assessment and management of environmental risks. A significant advantage of this approach is the improved sensitivity and specificity in detecting environmental contaminants, making it a valuable tool for researchers.
The unique feature of pollution tracking is the ability to provide real-time data on pollutant dispersion and interaction with ecosystems. Though effective, challenges exist in ensuring accurate data collection and developing techniques that can be integrated into existing monitoring systems.
Microbial Studies
Microbial studies benefit significantly from nanonics imaging, allowing for a deeper understanding of microbial behavior and interactions. This application focuses on visualizing microbial communities and their environments at an unprecedented level of detail. The main characteristic of this approach is its ability to distinguish between different microbial species and their metabolic activities.
This level of detail is beneficial in studying interactions between microbes and their hosts or ecosystems, which is essential for applications in health and environmental science. The unique feature is the ability to analyze microbial dynamics over time, although challenges remain in sample preparation and imaging conditions, which can affect results.
"Nanonics imaging technologies have made it possible to explore previously hidden dimensions within biological and material sciences, broadening the scope for innovation and discovery."
Challenges in Nanonics Imaging
Challenges within the field of nanonics imaging play a pivotal role in shaping its development and application. Understanding these challenges is crucial for researchers and practitioners. Several significant issues hinder the progression and practical use of this technology, making it important to recognize and address them.
Technical Limitations
Technical limitations present substantial obstacles in nanonics imaging. This section explores two primary challenges: instrument sensitivity and sample preparation issues.
Instrument Sensitivity
Instrument sensitivity is a key factor that affects the overall efficacy of imaging processes. High instrument sensitivity allows for the detection of minute signals, enabling accurate measurements at the nanoscale. This is significant because nanonics imaging often deals with extremely small structures where traditional microscopy may fail to provide clear information. However, achieving this sensitivity is difficult.
A notable characteristic of sensitive instruments is their ability to detect variations in signal strength. This can greatly benefit imaging outcomes, as it provides clearer and more detailed images. Nonetheless, sensitive instruments are also prone to noise, which can complicate data interpretation. This makes the balance between sensitivity and noise management an essential consideration in this field. Therefore, while high instrument sensitivity is critical, it must be managed carefully to improve nanonics imaging results.
Sample Preparation Issues
Sample preparation issues are another significant challenge in nanonics imaging. Proper sample preparation is essential for obtaining high-quality images. Inadequate preparation can lead to artifacts or misinterpretations of data. Researchers must take great care when preparing samples, as this stage can impact the entire imaging process. A crucial aspect of sample preparation is the need to maintain the integrity of the sample post-processing.
Specific techniques may include coating the sample or using chemical treatments that can alter its natural state. While these methods may enhance imaging quality, they can also introduce complications that lead to inaccurate results. Thus, balancing preparation methods with the original sample conditions remains a challenge in nanonics imaging.
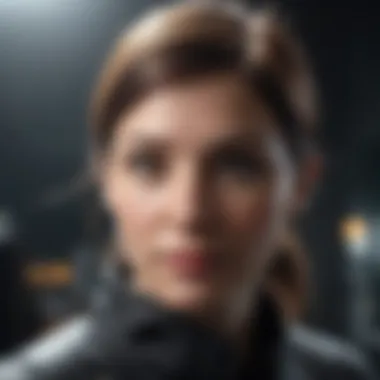
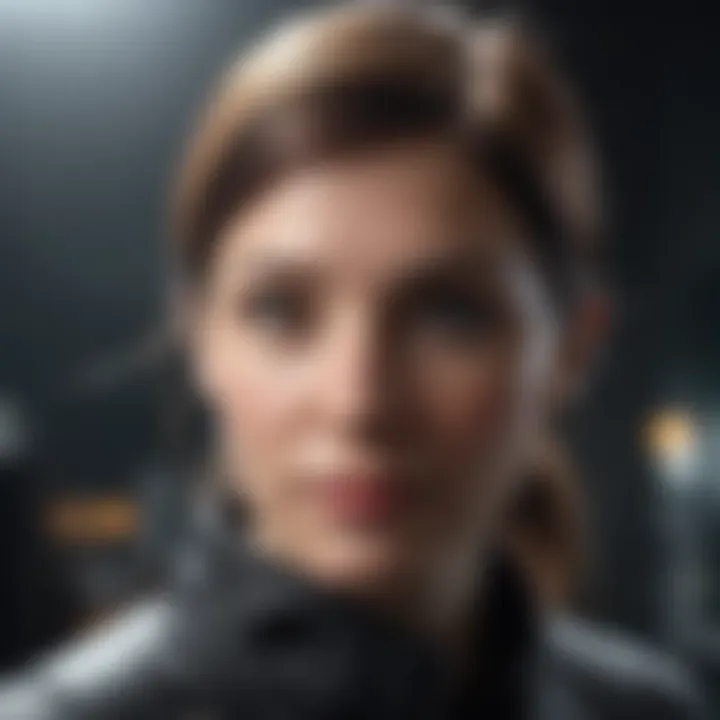
Costs and Accessibility
The financial aspects of nanonics imaging can also be limiting. The cost of advanced imaging equipment can be prohibitively high. This expense restricts access, particularly for smaller institutions or individual researchers, which may hinder scientific progress in the field. Advances in technology require substantial investment, and this can create a barrier to entry for many potential users.
Data Interpretation Challenges
Data interpretation remains a significant challenge in nanonics imaging. The complexity of the data generated by high-resolution imaging techniques can lead to difficulties in analysis. Researchers must possess a solid understanding of imaging principles and the underlying technology to draw valid conclusions. As imaging techniques evolve, they often produce more data than can easily be interpreted. This oversaturation of information can lead to potential misinterpretations. The need for advanced analytical tools and training in data interpretation is evident to handle the growing complexity of results obtained through nanonics imaging.
The combination of technical limitations, costs, and data interpretation challenges creates a multifaceted landscape that researchers must navigate to utilize nanonics imaging effectively.
Future Directions in Nanonics Imaging
The field of nanonics imaging continues to evolve, pushing the boundaries of what is achievable at the nanoscale. In this context, exploring future directions is crucial for scientists and researchers aiming to expand applications across various disciplines. As new technologies emerge and existing ones are improved, the potential benefits and considerations of these advancements deserve attention.
Integration with Other Technologies
Integrating nanonics imaging with complementary technologies offers exciting opportunities. For example, combining atomic force microscopy with optical imaging can provide multi-modal datasets. This integration enhances imaging capabilities, allowing for a more comprehensive understanding of complex biological systems and material properties. The synergy between techniques leads to improved spatial and time resolution in experiments.
Additionally, interfaces with artificial intelligence can greatly enhance data analysis. With advanced algorithms, researchers can derive meaningful insights from large datasets. This collaboration is expected to streamline the workflow, reduce errors, and expedite the research process.
Advancements in Computational Imaging
Advancements in computational imaging are fundamental to the future of nanonics. The use of algorithms in reconstructing images from raw data allows for enhanced resolution without physically altering the imaging hardware.
Techniques such as super-resolution imaging and machine learning can refine the data collection process. They provide high-quality images with greater detail and less noise. As computational power increases, the ability to handle more complex datasets will grow, leading to better interpretations of nanoscopic phenomena.
Potential for New Discoveries
The continuing refinement of nanonics imaging practices has significant implications for scientific discovery. Fields such as materials science and biology stand to benefit from technologies that facilitate deeper investigations into the nanoscale realm.
For instance, understanding the behavior of proteins at a molecular level can lead to breakthroughs in medical therapies. Similarly, examining how nanoparticles interact within various environments could drive innovations in product development.
Without a doubt, the possibilities for new discoveries due to the advancements in nanonics imaging are expansive. Each technological evolution unveils previously unseen details, opening doors to knowledge that can transform industries.
"The future of nanonics imaging is intertwined with other advancements in technology and computation, leading to unparalleled insights in science."
End
In summarizing the advancements in nanonics imaging, it is crucial to recognize its profound impact on the fields of science and industry. The unique capabilities of this imaging methodology enable researchers to observe and manipulate matter at the nanometer scale, which ultimately enhances our understanding of fundamental processes in materials and biological systems.
Summary of Findings
Nanonics imaging encapsulates a range of technologies and techniques that deliver impressive resolution and contrast when examining nanoscale structures. Key findings include:
- Technological Innovation: The integration of scanning probe microscopy, electron microscopy, and atomic force microscopy forms a comprehensive toolkit for precise imaging.
- Biomedical Applications: Techniques such as cellular imaging provide insights into physiological processes and disease mechanisms.
- Materials Science Impact: Nanonics imaging facilitates the characterization of nanomaterials, essential for developing next-generation materials with tailored properties.
- Environmental Importance: Applications in monitoring pollution and studying microbial life contribute significantly to ecological research and sustainability efforts.
Overall, these findings highlight the importance of nanonics imaging in bridging gaps in our knowledge and fostering innovation across interdisciplinary domains.
Implications for Science and Industry
The implications of advancements in nanonics imaging extend beyond academia and into various industries. Here are significant considerations:
- Enhanced Research Capabilities: Scientists can leverage these imaging technologies to produce high-resolution images that lead to breakthrough discoveries in nanotechnology and biomedicine.
- Industrial Applications: Companies can utilize these methods in quality control, ensuring the performance of nanostructured materials in their products.
- Education and Training: Institutions must adapt their curricula to include nanonics imaging, preparing the next generation of scientists and engineers.
Ultimately, the journey of nanonics imaging is a testament to human ingenuity. Its continual evolution promises to unearth new scientific principles and applications, fostering an era of unprecedented innovation in multiple fields.
"The most exciting discoveries often come from understanding what lies at the nanoscale."
This observation serves as a guiding principle as we move forward in exploring the potential of nanonics imaging.