Exploring the Mitochondrial Genome: Insights and Impact
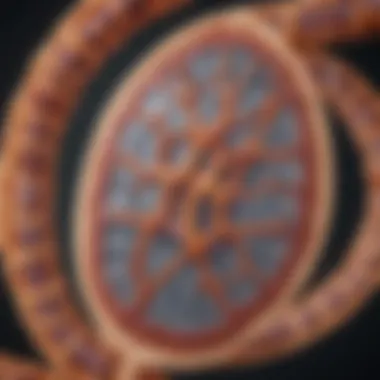
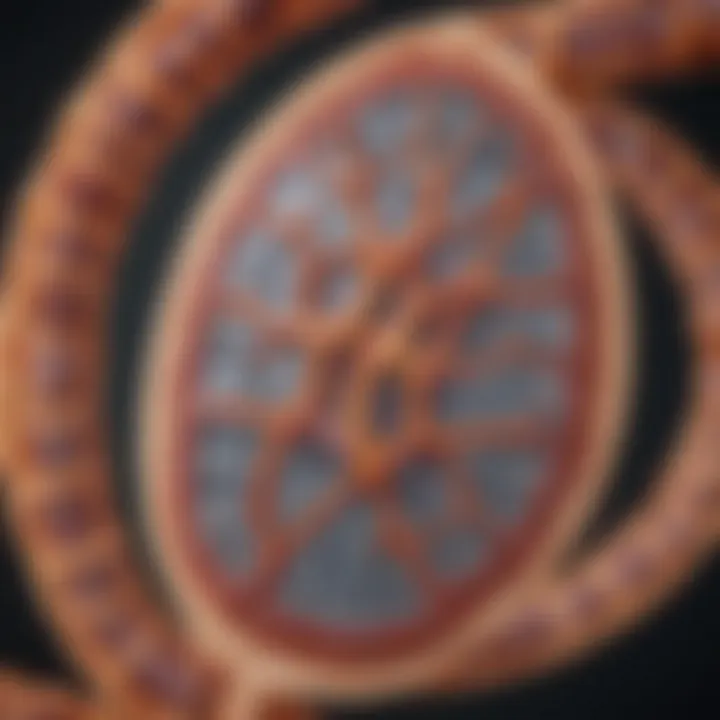
Intro
The mitochondrial genome holds a pivotal position within cellular biology, impacting various functions related to energy production and genetic inheritance. As the powerhouse of the cell, mitochondria are responsible for generating adenosine triphosphate (ATP), a critical energy source for numerous cellular processes. Understanding the intricate aspects of the mitochondrial genome is essential for grasping its implications on human health, evolutionary biology, and genetic research. This article aims to provide an in-depth exploration of the mitochondrial genome, elucidating its structure, functional significance, and potential implications for advancements in health and medicine.
The study of mitochondrial genetics involves several key areas, from the organization of mitochondrial DNA to its unique inheritance patterns. The maternal inheritance of the mitochondrial genome raises questions about its role in disease transmission and evolution. Additionally, recent methodological advancements have enriched our understanding, making it necessary to evaluate not only the technologies but also the ethical considerations in mitochondrial research.
In this article, we will delve into the methodology used in current mitochondrial research, the latest trends shaping the field, and the promising future directions for investigations that span the complexities of mitochondrial function and its implications for human health. By presenting these key points, readers will gain a well-rounded perspective on the significance and implications of the mitochondrial genome.
Prologue to the Mitochondrial Genome
The mitochondrial genome is an essential topic in cellular biology. It encodes critical genes responsible for the production of adenosine triphosphate (ATP), the primary energy currency of cells. Understanding this genome aids in exploring various biological processes and their implications for health and disease. The significance of the mitochondrial genome extends beyond basic energy metabolism; it serves as a cornerstone for understanding inheritance patterns, evolutionary history, and genetic disorders. Moreover, recent advancements in research techniques have opened new avenues for therapeutic applications, creating a compelling need for a thorough examination of this subject.
Definition and Function
The mitochondrial genome consists of a circular strand of DNA that exists in multiple copies within the mitochondria of cells. Unlike nuclear DNA, which is inherited from both parents, mitochondrial DNA is predominantly acquired from the mother. This unique inheritance pattern can provide insights into maternal lineage and evolutionary studies.
The primary function of the mitochondrial genome is to encode proteins that are vital for the process of oxidative phosphorylation. This process, occurring in the inner mitochondrial membrane, enables cells to generate ATP efficiently. In addition to protein coding, the mitochondrial genome also includes regions that are non-coding but play essential roles in regulating gene expression and maintaining mitochondrial function.
Historical Context
The history of mitochondrial research dates back to the mid-20th century when scientists discovered that mitochondria contained their own DNA. This revelation led to debates about the origins of mitochondria. The endosymbiotic theory, proposed in the 1970s, suggested that mitochondria evolved from free-living bacteria that were engulfed by ancestral eukaryotic cells. This theory reshaped our understanding of cellular evolution and demonstrated the close relationship between mitochondria and prokaryotic organisms.
Significant advancements in sequencing technologies over the past few decades have further advanced our understanding of mitochondrial genetics. With the ability to sequence more mitochondrial genomes, researchers have begun to uncover the connections between mitochondrial mutations and various diseases.
In summary, understanding the mitochondrial genome is crucial as it plays a significant role in fundamental biological processes and in the evolution of species. Such knowledge is key to unraveling the complexities of genetics and offers implications for health and disease management.
Mitochondrial Genome Structure
Understanding the structure of the mitochondrial genome is foundational to appreciating its role in cellular function and energy metabolism. Mitochondria, often referred to as the powerhouses of the cell, contain their own distinct DNA that is vital for their operation. This section will explore the genetic composition of mitochondrial DNA, its functional capabilities, and its importance in health and disease.
Genetic Composition
Single Circular DNA
The most notable characteristic of mitochondrial DNA is its single circular structure. Unlike nuclear DNA, which is linear and structured within a nucleus, mitochondrial DNA exists as a closed loop. This configuration aids in efficient replication and transcription, making it a highly effective model for studying genetics. A key benefit of this structure is that it allows for rapid adaptations, which can enhance evolutionary processes. The primary disadvantage, however, is its susceptibility to mutations. With its limited repair mechanisms, any damage can lead to significant dysfunction within the mitochondria.
Genes Encoding Mitochondrial Proteins
Mitochondrial DNA encodes several key proteins essential for mitochondrial function, particularly those involved in energy production. These genes are crucial for processes like the electron transport chain. Their presence directly impacts the efficiency of ATP production, underscoring the significance of these genes in overall cell metabolism. A unique feature is that many mitochondrial genes are inherited maternally, ensuring a direct lineage of mitochondrial function. The downside of this maternally driven inheritance is the potential for inherited disorders that stem from mitochondrial dysfunction.
Non-coding Regions
In addition to coding genes, mitochondrial DNA contains non-coding regions that play significant regulatory roles. These regions are essential for the control of gene expression and contribute to the overall stability of the mitochondrial genome. They serve as sites for transcription and may also regulate replication. Non-coding regions can be beneficial as they provide a wide range of functions beyond mere genetic information. However, they can also complicate genetic studies, as their functional roles are often less understood than those of coding genes.
Mitochondrial Gene Function
Mitochondrial genes have several critical functions that are essential for cellular vitality. Understanding these functions can offer insights into mitochondrial health and its implications for various diseases.
ATP Synthesis
One of the primary functions of the mitochondrial genome is ATP synthesis. This process is achieved via oxidative phosphorylation, where energy derived from nutrients is converted into adenosine triphosphate. ATP serves as the main energy currency of the cell. The ability of mitochondria to efficiently produce ATP is vital for sustaining cellular activities. However, any impairment in this process can lead to significant health issues, highlighting the critical role of mitochondrial genes in energy metabolism.
Oxidative Phosphorylation
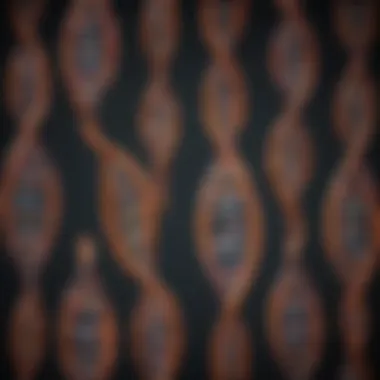
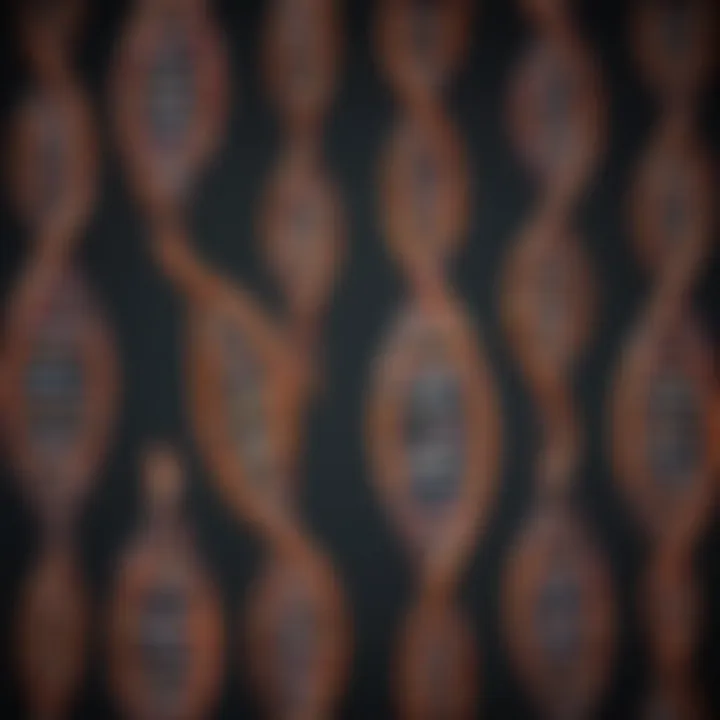
Oxidative phosphorylation is the process through which ATP synthesis occurs, relying heavily on proteins encoded within the mitochondrial DNA. This mechanism is critical as it maximizes energy extraction from nutrients. It represents a key advantage of mitochondrial function, as it permits various metabolic pathways to converge effectively. On the other hand, dysfunctions in oxidative phosphorylation can cause a range of metabolic disorders, emphasizing the need for further research in this area.
Mitochondrial Biogenesis
Mitochondrial biogenesis pertains to the process by which new mitochondria are formed within cells. This is governed by a range of nuclear and mitochondrial genes working in unison. The ability to adapt and increase mitochondrial numbers is crucial for meeting the cellular energy demands, especially during periods of high metabolic activity such as exercise. However, excessive biogenesis can lead to challenges in cellular function, leading to a need for careful regulation of this process.
Mitochondrial genome structure serves as a pivot around which cellular energy metabolism revolves, directly influencing health and disease outcomes.
Mitochondrial Inheritance
Understanding mitochondrial inheritance is crucial. This genetic mechanism greatly affects how traits associated with the mitochondrial genome are passed from one generation to the next. As noted, mitochondria are inherited almost exclusively from the mother. This peculiar pattern means any mitochondrial mutations will be passed down the maternal line. With more focus on mitochondrial inheritance, researchers can unlock various health insights, especially regarding mitochondrial diseases.
Maternal Inheritance
Mitochondrial DNA is predominantly inherited from the mother. During fertilization, the sperm contributes very little mitochondrial content. Thus, the mitochondrial genome of the offspring is that of the mother. This mechanism has significant implications. For instance, maternal inheritance patterns allow health professionals to trace mitochondrial diseases through maternal lineage. If a mother has a mitochondrial disorder, there is a chance her children will inherit the same condition. Some disorders, such as Leber's Hereditary Optic Neuropathy, demonstrate a clear maternal inheritance. This emphasizes the importance of maternal family history when assessing risk.
Paternal Contribution and Exceptions
While predominant, maternal inheritance does not always provide a complete picture. There are exceptions where paternal contribution occurs. In some species, paternal mitochondria can be inherited, but this is rare in humans. Additionally, certain paternal alleles in certain conditions might play a role, but they receive less focus due to their infrequent contribution.
Understanding these exceptions is vital for accurate genetic counseling. Research on paternal contributions to mitochondrial health is still in early stages. However, uncovering these nuances may reshape notions of inheritance. It might also lead to innovative strategies in treating mitochondrial diseases, especially by understanding all parental inputs.
The exploration of mitochondrial inheritance sheds light on genetic counseling and understanding family health histories. This understanding could significantly alter clinical practices.
Evolutionary Significance
The exploration of the mitochondrial genome is crucial for understanding its evolutionary significance. It provides insights into the origins and development of complex life forms. The mitochondrial genome serves as an indicator of evolutionary relationships among species. Its unique inheritance pattern adds an additional layer of complexity to studies in evolutionary biology. By analyzing mitochondrial DNA, researchers can trace lineage and understand how species have diverged over time.
Endosymbiotic Theory
The endosymbiotic theory proposes that mitochondria originated from free-living prokaryotic organisms that entered into a symbiotic relationship with ancestral eukaryotic cells. This theory explains how mitochondria, with their distinct double-membrane structure and circular DNA, closely resemble bacteria. The implications of this theory are significant since it suggests that a major evolutionary leap occurred when these prokaryotes became integral to eukaryotic cells.
Mitochondria are essential for ATP production and energy metabolism. Their presence in nearly all eukaryotic cells indicates a pivotal moment in the evolution of life. Through this endosymbiotic process, cells gained the ability to efficiently convert energy, leading to the development of larger and more complex organisms. The endosymbiotic theory not only provides an understanding of mitochondrial origin but also highlights the importance of inter-organismal cooperation in evolution.
Mitochondrial Redundancy and Diversity
Mitochondrial redundancy and diversity refer to the variations and backup systems present within the mitochondrial genome across different species. This redundancy is important for the survival and adaptability of organisms. For example, some organisms possess multiple mitochondrial DNA copies, which can provide resilience against mutations.
Additionally, diversity among mitochondrial genomes can lead to variations in energy production and metabolic pathways. These differences have evolutionary consequences. Certain variants may confer advantages under specific environmental conditions. Thus, the genetic diversity of mitochondria plays a role in evolutionary fitness and adaptation.
Mitochondrial Dysfunction and Disease
Mitochondrial dysfunction is a critical aspect in the study of cellular and metabolic diseases. This topic encompasses various disorders linked to the malfunctioning of mitochondria, the organelles responsible for producing energy in cells. These dysfunctions can lead to a range of health problems, often affecting multiple systems in the body. Understanding mitochondrial dysfunction illuminates the complexity of energy metabolism and its direct implications on human health. Therefore, it is essential to explore this topic in depth to recognize its significance in both inherited and acquired conditions linked to mitochondrial health.
Inherited Mitochondrial Disorders
Inherited mitochondrial disorders are genetic conditions that arise when there is a mutation in mitochondrial DNA. These disorders can be transmitted from mother to child due to the maternal inheritance pattern of mitochondrial DNA. Such disorders often present a variety of symptoms, which may include muscle weakness, neurological problems, and metabolic complications.
The clinical heterogeneity of these disorders arises from the nature of mitochondrial DNA variations and how they affect different tissues. The severity and range of symptoms can vary greatly, even among individuals sharing the same mutation. This makes early diagnosis and treatment challenging. Several well-known inherited mitochondrial disorders include Leigh syndrome, mitochondrial myopathy, and Leber's hereditary optic neuropathy. By exploring the genetic basis of these conditions, researchers can gain insights into potential therapeutic approaches and management strategies.
Acquired Mitochondrial Dysfunction
Acquired mitochondrial dysfunction refers to the decline in mitochondrial function due to environmental factors or lifestyle choices rather than genetic mutations. Several factors can contribute to this dysfunction, including toxins, oxidative stress, and nutrient deficiencies. The acquired nature of this dysfunction makes it particularly important from a preventive healthcare perspective.
Impact of Environmental Factors
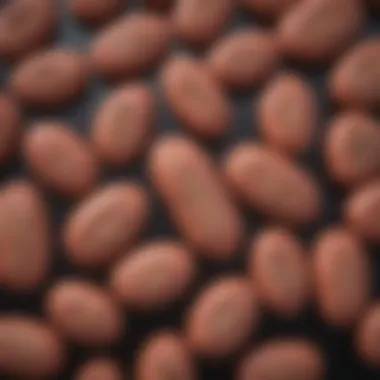
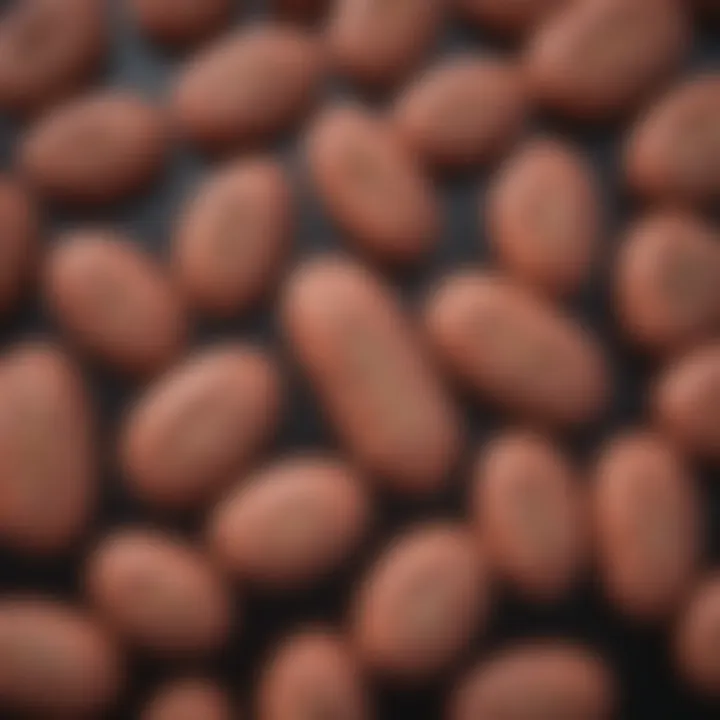
Environmental factors play a significant role in the impairment of mitochondrial function. Exposure to pollutants, heavy metals, and certain chemicals can directly affect mitochondrial activity, leading to oxidative damage. For instance, exposure to pesticides or air pollution can result in increased production of reactive oxygen species. This oxidative stress can compromise mitochondrial integrity and function.
The key characteristic of the impact of environmental factors is its potential for prevention and modification. Unlike genetic factors, addressing environmental contributions could lead to lifestyle changes or interventions to mitigate risks. A unique feature to note is the variability in individual responses to environmental stressors, which can complicate the study of their effects on mitochondrial health. Though preventing exposure to harmful substances poses certain challenges, awareness can encourage healthier environments and communities.
Age-related Decline
Age-related decline in mitochondrial function is another significant concern. As individuals age, mitochondria may become less efficient in energy production. This decline is often associated with a range of age-related diseases, including Alzheimer's disease and cardiovascular issues. The key characteristic of age-related decline is the progressive loss of mitochondrial biogenesis, which reduces the overall number of functional mitochondria in cells.
This aspect is critical for understanding the aging process. The unique feature of age-related decline is that it can be influenced by both genetics and lifestyle. A balanced diet and regular exercise can contribute to maintaining mitochondrial function as one ages. However, the disruptive impacts of an unhealthy lifestyle can lead to accelerated mitochondrial dysfunction.
In summary, both inherited and acquired mitochondrial dysfunction has far-reaching implications for health and wellness. By exploring the interplay between genetic predisposition and environmental factors, researchers can seek innovative solutions to combat mitochondrial-related diseases.
Understanding mitochondrial dysfunction can potentially improve therapeutic strategies targeting critical conditions.
For those interested in learning more about mitochondrial disorders and their implications, resources like Wikipedia provide a wealth of information.
Methods for Studying the Mitochondrial Genome
Understanding the mitochondrial genome has become essential for advances in various fields, including genetics, medicine, and evolutionary biology. The methods used to study this genome improve our knowledge of its structure and function. They also help elucidate the role of mitochondrial DNA in disease and inheritance. As the mitochondrial genome has unique characteristics, specialized research techniques are necessary. In this context, various methods have arisen out of necessity, including sequencing technologies and bioinformatics tools. These methods not only enhance the depth of research but also provide crucial insights that contribute to medical advancements.
Next-Generation Sequencing Techniques
Next-generation sequencing (NGS) techniques have revolutionized how researchers analyze the mitochondrial genome. NGS allows for high-throughput sequencing, meaning a large amount of data can be processed in a short time. Unlike traditional sequencing, which can be time-consuming, NGS provides a comprehensive view of the mitochondrial genome, revealing its complexity and variation among individuals.
These techniques are instrumental in identifying mutations associated with diseases linked to mitochondrial dysfunction. Researchers can obtain complete mitochondrial DNA sequences with high accuracy. This precision is key when studying inherited mitochondrial disorders, allowing for direct correlation between specific genetic alterations and phenotypic expressions.
Some specific NGS methods employed in mitochondrial research include:
- Whole Genome Sequencing: This approach sequences the entire genome, providing insights into both nuclear and mitochondrial DNA.
- Targeted Sequencing: This method focuses on specific regions of the mitochondrial genome, useful for studying known mutations in detail.
Overall, NGS is pivotal in expanding the understanding of mitochondrial genetics, paving the way for future clinical applications.
Bioinformatics Tools
Bioinformatics plays a crucial role in the manipulation and interpretation of data generated from sequencing techniques. The vast amounts of information produced by NGS require sophisticated software and algorithms to analyze it. Bioinformatics tools help in aligning sequences, identifying variants, and predicting their functional impact. These tools also facilitate the integration of mitochondrial genomic data with other omics data, such as transcriptomics and proteomics.
Several popular bioinformatics tools used in mitochondrial genome studies include:
- MitoTool: This tool specializes in the analysis of mitochondrial DNA sequences, aiding researchers in sequence alignment and variant calling.
- MITOS: A tool for annotation of mitochondrial genomes, it identifies genes and provides insights into their functions.
- MAFFT: Used for multiple sequence alignment, crucial for comparing mitochondrial sequences across different species.
Utilizing these bioinformatics tools enables researchers to extract meaningful findings from their data, leading to a deeper understanding of mitochondrial biology and its implications for health.
Bioinformatics is essential in synthesizing genomic data into actionable insights, allowing for advancements in medical research and personalized medicine.
Therapeutic Implications
The exploration of the mitochondrial genome is not limited to understanding its structure and functions. It also opens pathways for therapeutic applications that can improve health outcomes. Understanding how mitochondrial DNA influences cellular metabolism and the manifestation of mitochondrial diseases is key. This section delineates significant therapeutic approaches and their potential in the realm of healthcare, emphasizing both gene therapy and pharmacological interventions.
Gene Therapy Approaches
Gene therapy represents a paradigm shift in treating mitochondrial disorders. This method attempts to correct genetic defects at the molecular level, providing a promising avenue for the treatment of conditions stemming from mitochondrial dysfunction.
One notable approach involves the use of nuclear transfer techniques. Researchers aim to transfer healthy mitochondrial DNA into cells of affected individuals. This can potentially restore normal function and halt disease progression. Another method employs CRISPR-Cas9 technology for precise gene editing. By targeting faulty genes, scientists can reduce or eliminate the expression of deleterious mutations.
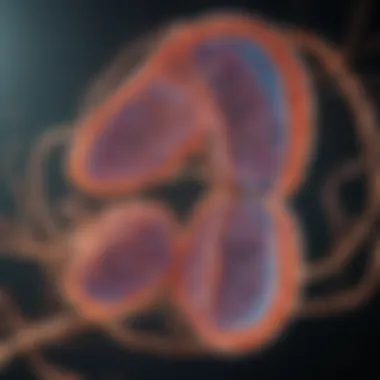
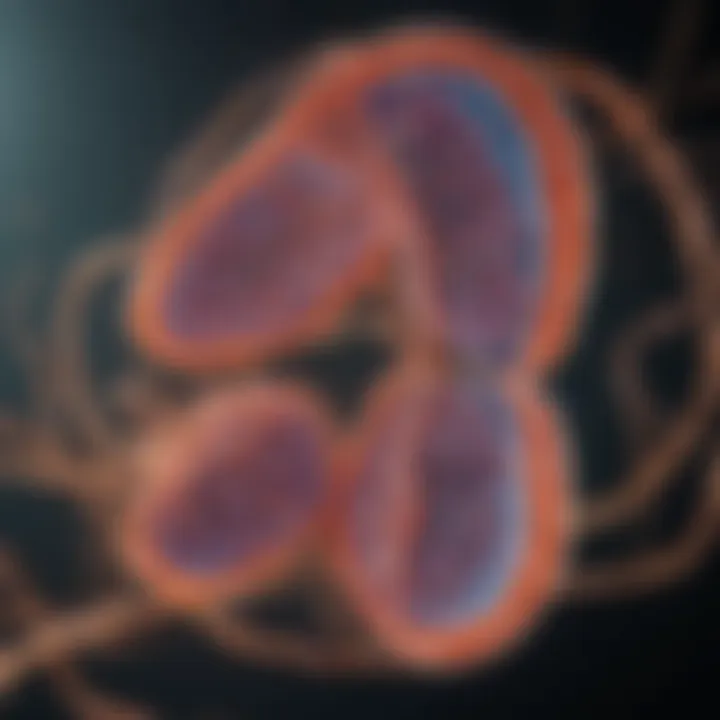
- Benefits of gene therapy include:
- Potential restoration of normal mitochondrial function.
- Reduction in symptoms associated with mitochondrial disease.
- Long-lasting effects as corrected genes can be passed to future generations.
However, there are also significant considerations. The delivery mechanisms for these therapies require careful optimization to ensure that the correct tissues are targeted. Additionally, there are ethical implications regarding the extent of genetic modifications, particularly concerning germline editing.
"Gene therapy not only poses questions about efficacy but also about safety, as unanticipated mutations could arise during the editing process."
Pharmacological Interventions
Pharmacological interventions represent another critical approach toward addressing mitochondrial dysfunction. These interventions can be categorized into symptomatic treatments and disease-modifying therapies.
- Symptomatic Treatments: These are designed to alleviate the symptoms of mitochondrial diseases. They include medications aimed at improving energy production or reducing oxidative stress.
- Disease-Modifying Therapies: Unlike symptomatic treatments, these aim to address the underlying causes of mitochondrial dysfunction, potentially restoring normal cellular function.
Some key classes of drugs in this area include:
- Mitochondrial-targeted antioxidants: These are developed to counteract oxidative damage that can exacerbate mitochondrial dysfunction. Drugs like MitoQ show promise in this domain.
- Nutraceuticals: Compounds such as Coenzyme Q10 and L-carnitine have been studied for their roles in enhancing mitochondrial function and energy production.
While these pharmacological interventions offer hope, their effectiveness may vary among individuals depending on the specific mutation or disorder. Ongoing clinical trials continue to evaluate the efficacy of these treatments, aiming to provide data to guide practitioners in choosing suitable therapeutic options.
In summary, both gene therapy and pharmacological interventions hold significant promise in tackling mitochondrial diseases. As research progresses, these therapeutic implications will likely evolve, providing new possibilities for intervention and improved patient outcomes.
Ethical Considerations in Mitochondrial Research
The examination of ethical considerations in mitochondrial research is paramount due to the profound implications this field holds for human health and genetic integrity. As science progresses, particularly in areas such as gene therapy and mitochondrial replacement therapy, ethical questions arise concerning the manipulation of human life. This article will explore two primary areas of concern: mitochondrial replacement therapy and implications for human modification.
Mitochondrial Replacement Therapy
Mitochondrial replacement therapy is an innovative technique aimed at preventing the transmission of mitochondrial diseases from mother to child. This procedure often involves the transferring of nuclear DNA from a mother with mitochondrial mutations into the donor oocyte that has healthy mitochondria. The result is an embryo with genetic material from three individuals: the mother, the father, and the mitochondrial donor.
This method offers the possibility to eradicate certain genetic disorders, but it raises significant ethical dilemmas. Some of the primary concerns include:
- Consent and Autonomy: Issues regarding informed consent arise when discussing the implications of third-party mitochondrial donation. Future implications for the offspring due to the genetic contributions of three individuals must be comprehensively addressed to respect parental autonomy and decision-making.
- Genetic Modification: While mitochondrial replacement does not alter nuclear DNA directly, it does represent a form of genetic modification. The societal acceptance of such modifications remains under scrutiny as it may lead to unexpected genetic outcomes in future generations.
- Regulation and Oversight: There is a need for clear regulatory frameworks to manage mitochondrial replacement. As this field evolves, it is crucial to balance rapid scientific advancement with ethical oversight to prevent exploitation and misuse.
The potential benefits of mitochondrial replacement therapy are significant, including the prevention of debilitating diseases. However, these benefits must be weighed against moral and ethical considerations, ensuring that future generations are safeguarded.
Implications for Human Modification
The ability to alter mitochondrial DNA through therapies has broader implications for human modification. As the technologies develop, it can potentially lead to choices about physical traits, disease resistance, and other genetic characteristics.
Considerations in this area include:
- Designer Babies: The prospect of creating ‘designer babies’ through selective genetic modifications of mitochondrial and nuclear DNA raises ethical issues regarding equity and access. There is a concern that such practices may only be available to elite groups, creating a genetic divide in society.
- Long-term Consequences: The long-term effects of genetic modifications are still not fully known. Altering mitochondrial DNA could have unforeseen impacts that affect not just the individual but also their descendants.
- Philosophical and Cultural Dimensions: Different cultures hold diverse beliefs about genetic manipulation. Ongoing dialogues within various communities are needed to address these differences and recognize the broader societal impact of these technologies.
Future Directions in Mitochondrial Research
As the field of mitochondrial research evolves, it is essential to explore future directions that may impact our understanding of this complex organelle. Mitochondria are not only crucial for energy production, but they also play vital roles in various cellular processes including apoptosis, calcium homeostasis, and the generation of reactive oxygen species. This section aims to highlight the importance of advancing research methodologies and potential applications that could lead to significant breakthroughs in understanding mitochondrial function and related diseases.
Continuous exploration in this area holds several benefits. For instance, uncovering the intricacies of mitochondrial dynamics can inform therapeutic strategies for mitochondrial disorders and enhance our understanding of age-related diseases. Furthermore, as we shift towards a more integrated approach in biology, the collaboration between multiple fields of research becomes vital to addressing complex biological questions.
Integration of Multi-Omics Approaches
Integrating various omics technologies—such as genomics, transcriptomics, proteomics, and metabolomics—allows us to observe mitochondrial biology from multiple perspectives. This strategy is essential for providing a holistic view of the interactions and mechanisms underpinning mitochondrial function. The integration of these datasets offers promise in identifying novel biomarkers and therapeutic targets for diseases linked to mitochondrial dysfunction.
Researchers are beginning to utilize these multi-omics approaches to assess not only genetic information but also the complex biochemical processes occurring within mitochondria under different physiological and pathological conditions. This comprehensive analysis can help in understanding how various factors contribute to mitochondrial health and disease.
Potential for Mitochondrial Biomarkers
The exploration of mitochondrial biomarkers continues to garner attention. These biomarkers can serve as vital indicators of mitochondrial health and can aid in the early detection of diseases such as diabetes, neurodegeneration, and certain cardiovascular conditions. Moreover, mitochondrial DNA variations can be utilized as specific markers to trace lineage and understand human evolutionary biology.
The development of reliable mitochondrial biomarkers is critical for implementing personalized medicine approaches. By tailoring therapies based on individual mitochondrial profiles, healthcare practitioners can enhance treatment efficacy and minimize side effects.