M3 Technologies in Molecular Devices: Innovations and Impact
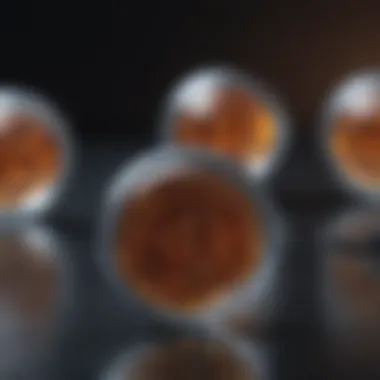
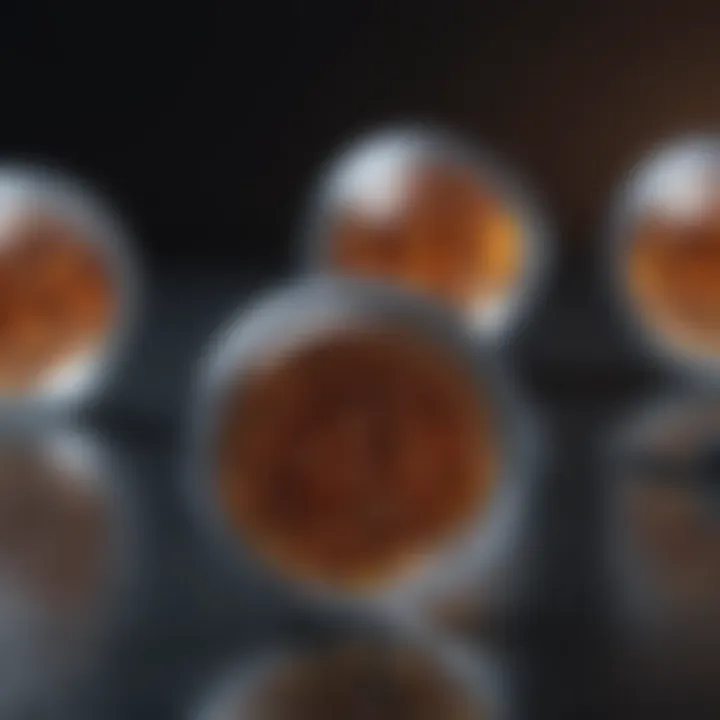
Intro
The landscape of molecular devices is undergoing a revolution, with M3 technologies emerging at the forefront. The significance of these advancements cannot be overstated; they are reshaping fields from medicine to materials science. Whether it’s through novel fabrication techniques or the diverse functions enabled by such innovations, these molecular devices are poised to drive scientific inquiry into new territories.
Exploring these devices invites us to think beyond conventional methodologies. It requires an understanding not just of what these technologies can achieve but also how they intertwine with varied disciplines. The implications of M3 technologies extend across scientific boundaries, propelling collaboration among researchers from multiple specialties.
Key Points to Consider:
- The shift towards molecular electronics and biocompatible materials.
- The pivotal role of interdisciplinary efforts in advancing research.
- Upcoming trends that signal the future direction of molecular devices.
In our journey through this intricate field, we will tackle aspects of methodology, future directions, and how M3 technologies act as a bridge linking theoretical research to practical applications.
Prolusion to Molecular Devices
The realm of molecular devices has become a cornerstone of modern scientific innovation, catalyzing advances across disciplines ranging from nanotechnology to biomedicine. The significance of understanding molecular devices lies not only in their theoretical framework but also in their practical applications. These devices operate on the fundamental principles of molecular interactions, making them pivotal in the development of more efficient technologies. An in-depth exploration into this topic reveals both the astonishing capabilities and the challenges faced in their design and implementation.
Defining Molecular Devices
Molecular devices can be characterized as systems characterized by molecular components that perform particular functions or facilitate specific processes at the molecular level. They range from simple sensors that can detect environmental changes to complex circuits capable of computing at unprecedented scales. The unique aspect of these devices is their reliance on the intricate behavior of molecules, allowing for functionalities that are not typically achievable through conventional technologies. For instance, molecular switches can serve as on/off controls at the most fundamental levels.
These devices include a multitude of applications:
- Molecular Sensors: Designed to monitor chemical changes, allowing for real-time data collection.
- Nanomaterials: Employed in energy storage or conversion, harnessing efficiencies that far surpass traditional methods.
- Bioelectronics: Bridging the gap between biological systems and electronic devices, which can revolutionize medical diagnostics.
Their ability to operate at a nanoscale is a double-edged sword; while it opens the door to innovation, it also introduces complexity in both their functioning and real-world applications. This complexity makes defining molecular devices under a unified framework particularly challenging yet necessary.
Historical Context and Development
The historical backdrop of molecular devices traces back to the early investigations into molecular structure and behavior. The origins can be linked to foundational chemistry and physics principles, but significant advancements began to emerge in the late 20th century. Researchers gradually realized the potential of manipulating molecular interactions to create devices that could perform specific tasks.
One early example involves the development of molecular wires, which laid the groundwork for understanding how to control electron flow at a molecular level. This was a monumental leap that paved the way for the design of more sophisticated devices. Over the years, many researchers have contributed to the expanding library of molecular devices, usign techniques such as organic synthesis and nanotechnology during this exploration.
As the field matured, collaborations with disciplines such as materials science and engineering led to significant breakthroughs. The realization that molecular devices could mimic biological processes or enhance electronics brought forth a new arena of interdisciplinary research, where the line between biology and technology began to blur.
Today, advancements in characterization techniques and computational modeling further enable scientists to predict and manipulate molecular behaviors, continually pushing the boundaries of what is possible with molecular devices. The journey from conceptual understanding to practical application is an exciting narrative, filled with both trial and error and astoundingly fruitful discoveries.
"Understanding the past is essential when forging paths to the future in molecular technology. The lessons learned from historical advancements guide us in tackling current challenges."
Overall, comprehending the nuances of molecular devices sets the stage for delving into M3 technology and its implications in both scientific and societal contexts. The exploration of this intersection is what ultimately drives continuous innovation in the field.
Overview of M3 Technology
M3 technologies represent a pivotal advancement in the realm of molecular devices, carving out a unique niche that blends intricate science with practical applications. Understanding this framework is crucial for anyone delving into the nuances of molecular devices, as the implications are vast and touch various fields including materials science, nanotechnology, and electronic engineering. This overview not only sketches the significance of M3 technology but also sheds light on the specific categories and core principles that underline their functionality.
Classification of M3 Molecular Devices
M3 molecular devices can be classified into several categories based on their functionality and applications. Below are some prominent types:
- Molecular Sensors: These devices detect specific biological or chemical markers through a molecular recognition process. Examples include glucose sensors for diabetes management.
- Molecular Switches: They operate by altering their state in response to external stimuli, which can be electrical, chemical, or optical. These switches play pivotal roles in data storage and retrieval processes.
- Molecular Actuators: These convert electrical signals into mechanical motion at a molecular level, making them suitable for applications in robotics and micro-manipulation.
Understanding how these classifications interact and what makes each distinct is fundamental to grasping the overall utility of M3 technologies. Not only does this help in distinguishing between device types, but it also informs potential advancements that might arise when integrating features from one category to another.
Core Principles Governing M3 Devices
The operational principles of M3 devices are based on several key aspects that define their effectiveness and usability. These include:
- Molecular Recognition: At the heart of many M3 devices lies the principle of molecular recognition, which facilitates selective interaction with target molecules. This characteristic is vital for sensors looking to detect specific substances.
- Self-Assembly: Several M3 technologies rely on self-assembly for fabrication, where molecules spontaneously form structured arrangements. This principle is not only economical but also enhances the precision of device construction.
- Electronics at the Nanoscale: M3 devices often employ nanoscale phenomena, such as tunneling and capacitive effects, playing a crucial role in their electronic performance. The ability to manipulate electrons at a molecular level can lead to breakthroughs in energy efficiency.
In summary, a thorough examination of M3 technologies’ classifications and core principles lays the groundwork for understanding their deeper implications in practical applications. By familiarizing oneself with these elements, researchers and professionals can better appreciate the transformative potential that these molecular devices hold for future technological advancements.
"The future of technology increasingly lies at the intersection of molecular discovery and engineering excellence.”
As we move forward, it’s essential to consider not only the current implications but also how M3 technologies might evolve and transform industries as we know them.
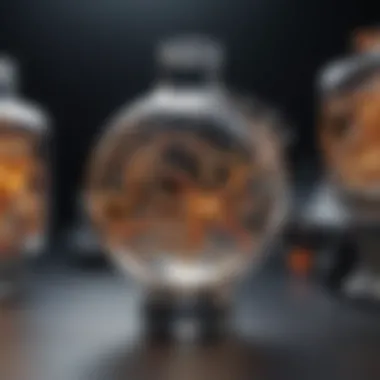
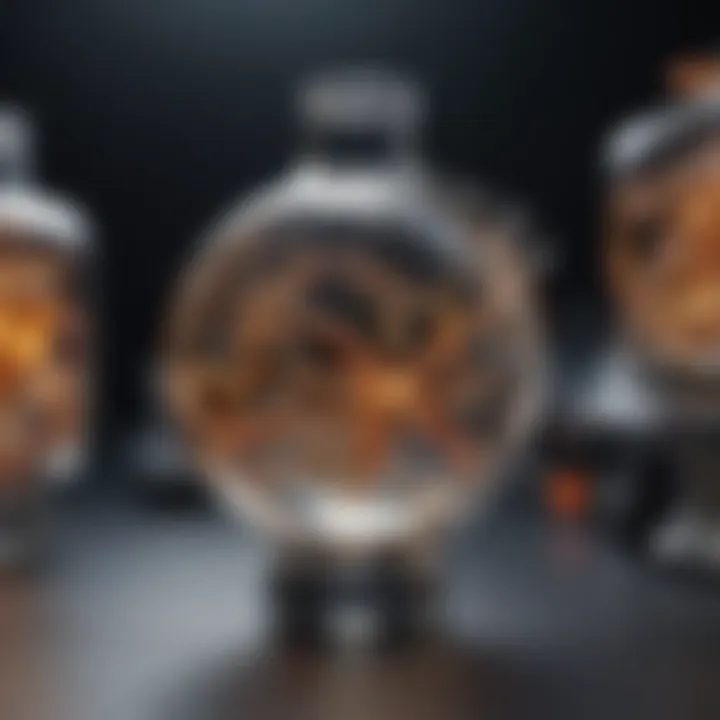
Fabrication Techniques for M3 Devices
The fabrication of M3 molecular devices constitutes a fundamental aspect of their development, as it directly influences their functionality, efficiency, and potential applications. Effective fabrication techniques not only enhance performance but also enable researchers to create devices that can be scaled for commercial uses. Recognizing the impact of various methods can guide innovators in pushing the boundaries of what's achievable in the realm of molecular technologies.
Methods of Synthesis
M3 devices can be synthesized using a variety of methods, and each one comes with its own set of advantages and challenges. Let's delve into a couple of prominent synthesis methods:
- Self-assembly: This approach involves molecules organizing themselves into structured arrangements without external guidance. For example, DNA origami takes advantage of the natural affinity of nucleic acids for each other. Self-assembled structures can be remarkably complex, yet they offer a straightforward pathway to fabricate devices at the nanoscale.
- Chemical vapor deposition (CVD): CVD is a widely used technique for creating thin films of materials on substrates. It's particularly valuable in producing high-quality semiconductors and nanostructures. This method's ability to produce uniform layers makes it an ideal choice for precision applications.
These methods represent just the tip of the iceberg when it comes to synthetic approaches. As the field evolves, novel techniques are continuously emerging, each promising to enhance our capabilities further.
Scalability and Industrial Applications
The transition from laboratory-scale devices to industrial production is a critical juncture for M3 technologies. Scalability remains a prominent concern, as methodologies need to be practical for widespread manufacturing while retaining quality and performance. A few considerations include:
- Cost management: To make devices commercially viable, synthesis methods must be cost-effective. Scaling up can often lead to increased costs, so balancing quality with expense is vital.
- Materials compatibility: Creating devices that can effectively integrate with existing materials in industrial settings adds another layer of complexity. Addressing compatibility issues can pave the way for innovative applications ranging from consumer electronics to sustainable energy solutions.
- Automation potential: Mechanizing synthesis processes not only can improve efficiency but also ensures consistency. Advances in robotic systems and AI-driven optimization may well redefine how we approach fabrication.
Research institutions have taken strides to establish frameworks conducive for scaling up, which plays a key role in ensuring these technologies have a lasting impact on the market.
"The effective synthesis and scaling of M3 devices can propel innovations across various sectors, enhancing performance and sustainability."
In summary, understanding fabrication methods in M3 device development is essential, as it influences their viability for future applications. Researchers must tread carefully, balancing innovation with practical aspects to expand the potential of molecular devices.
Functional Diversity in M3 Devices
The functional diversity present in M3 devices is a substantial factor that amplifies their value in various applications. As scientists and engineers explore the potential of these molecular technologies, understanding their varying functions leads to significant advancements across disciplines such as nanotechnology, medicine, and energy solutions. M3 devices are not crafted from one-size-fits-all principles; they're designed to adapt to a multitude of tasks. This flexibility is a game-changer for industries looking to innovate.
A few specific elements contributing to their functional diversity include:
- Tailored Chemical Properties: Each M3 device can have unique chemical properties which enable them to engage in specific Reactions. From catalysis to molecular sensing, these characteristics make them remarkably versatile.
- Varied Electrical Conductivity: The electrical behavior of M3 devices is essential for applications in computing and sensors. Devices can be fine-tuned to exhibit either high conductivity or semiconducting properties, which gives them a broader role in electronic circuits.
- Optoelectronic Characteristics: Many M3 devices exhibit significant optoelectronic features, making them suitable for use in light-emitting devices and solar cells. These properties enable them to absorb and emit light efficiently, which enhances their applicability in renewable energy.
"Functional diversity in M3 devices is a key pillar for innovation, opening doors to new possibilities across various scientific fields."
Chemical Properties and Reactions
Diving into the molecular level, the chemical properties of M3 devices dictate how they interact with other chemical species. This interaction can lead to novel reactions that are only possible under specific conditions. For instance, some M3 devices have been seen to act as highly efficient catalysts in chemical reactions, pushing reaction kinetics to new heights. They can enhance reaction rates while minimizing energy consumption, presenting substantial advantages in industrial processes.
In the realm of drug delivery, certain M3 devices have the capability to respond to biochemical stimuli, allowing for targeted delivery of pharmaceuticals. Their ability to change form or function based on the pH environment or presence of specific molecules provides a tailored approach to medical treatment, holding great promise for personalized medicine.
Electrical and Thermal Conductivity
When we turn our attention to electrical and thermal conductivity, it’s clear these two characteristics are interlinked with the functional capabilities of M3 devices. Electrical conductivity is imperative for integrated circuits and devices like sensors. The unique molecular structure of M3 materials allows for fine-tuning, enabling them to achieve desired conductivity properties; some can even function as superconductors under certain conditions.
Thermal conductivity is likewise crucial—especially in applications where heat dissipation is vital, like in battery technologies. By optimizing thermal properties, M3 devices can ensure efficient heat management, prolonging the lifespan of systems in which they're included. Knowing how to leverage these conductive properties effectively can lead to optimized device performance.
Optoelectronic Features
Finally, the optoelectronic features of M3 devices cannot be understated. These characteristics play a vital role in applications involving light and electronic interactions. For example, innovations in photovoltaic cells greatly benefit from this functional diversity, where efficiency in light absorption translates directly to energy generation. The materials' ability to both emit and detect light paves the way for advances in photonics and telecommunication technologies.
Furthermore, the integration of optoelectronic M3 devices with other technologies has resulted in compact and highly efficient systems. These advancements herald a future where smart devices and sensors are even more effective, adaptive, and interconnected.
Applications of M3 Molecular Devices
The topic of Applications of M3 Molecular Devices serves as a crucial pivot point in understanding the broader implications of this innovative technology. Their relevance spans several domains, highlighting the versatility and transformative potential inherent in M3 devices. As we dive deeper into their applications, it is important to recognize the benefits they yield, as well as certain considerations that come along for the ride.
Role in Nanotechnology
M3 molecular devices play a pivotal role in the fields of nanotechnology, bridging the gap between molecular science and practical applications. They can be fine-tuned at such a small scale that they open up an array of possibilities. For instance, researchers are developing nanoscale sensors that can detect environmental pollutants at trace levels. The significance of M3 devices here is underscored by their ability to improve the sensitivity and specificity of these sensors, ultimately leading to more effective monitoring of ecological health.
Moreover, in drug delivery systems, M3 devices enable targeted therapies. By utilizing molecular recognition, medications can be delivered precisely where they are needed, reducing side effects and improving treatment efficacy. This precision results from the unique interactions at the molecular level, showcasing how M3 devices are not just more efficient; they are also more ethical in their operation.
Design considerations for these applications include the stability of molecular structures under operational conditions.
"M3 technologies walk a fine line. They need to be potent enough to provide results yet stable enough to perform under varied conditions."
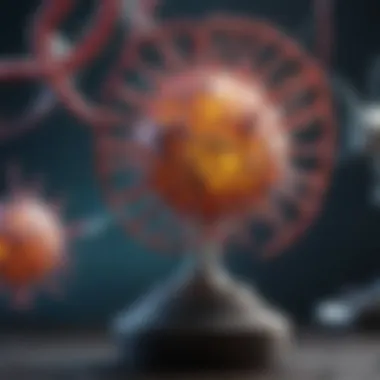
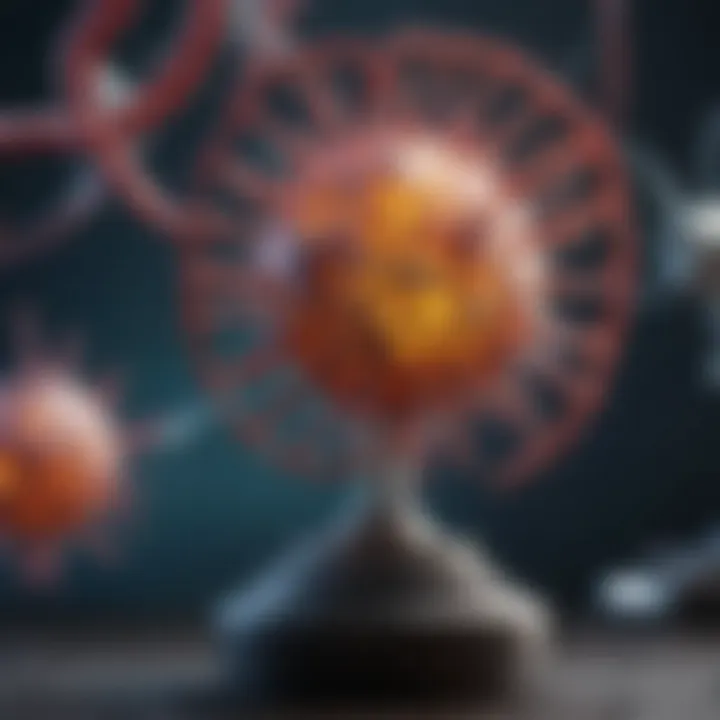
Impacts on Biological Systems
The interface between M3 devices and biological systems presents an exciting array of possibilities that raise both hopes and ethical concerns. Their application in biomedicine, particularly in diagnostics and therapeutics, is transformative. M3 molecular devices can assist in real-time monitoring of biological processes, paving the way for personalized medicine. Imagine a scenario where wearable devices continuously monitor glucose levels in diabetic patients, alerting them when intervention is necessary—all thanks to the functions of M3 technology.
However, the intricacies also underline the importance of assessing long-term impacts. Questions arise regarding biocompatibility and potential toxicity. It’s not just about what these devices can do; it’s about how they do it and the subsequent effects on human health. The ongoing research in this field ensures an understanding of these interactions becomes part of the design process from the get-go.
M3 Devices in Energy Storage Solutions
In the realm of energy storage, M3 molecular devices emerge as game-changers. They're paving the way for more efficient batteries and supercapacitors by allowing for molecular-level efficiencies in charge storage and transfer. For instance, innovations in lithium-sulfur battery technology leverage M3 devices to improve overall energy density while mitigating the common drawbacks found in traditional battery types.
Benefits also extend to renewable energy. M3 devices can be engineered to optimize energy conversion processes in solar cells, enhancing their overall effectiveness.
However, the economic feasibility of scaling up these technologies poses a pressing challenge. The balance between high-performance output and cost-effectiveness remains a serious discussion point among researchers and manufacturers. The goal is to create solutions that are not only cutting-edge but also accessible to a wider audience, thereby encouraging adoption in varied markets.
Through a comprehensive understanding of these applications, one begins to build a mental framework around M3 technologies. Their embedded potential crosses paths with critical issues of sustainability and ethics, demanding that stakeholders include multidisciplinary insights for responsible and innovative developments.
Challenges in the Implementation of M3 Technologies
The journey towards incorporating M3 technologies into wider applications is rife with hurdles that can trip even the most industrious minds. As cutting-edge as these molecular devices may be, the challenges in their implementation can seldom be overlooked. Understanding these hurdles is critical as they not only highlight the current limitations but also point toward opportunities for innovation and growth in this fascinating field.
Technical Limitations
When we dive into the technical limitations of M3 technologies, it becomes clear that the marvelous potential these devices hold is somewhat shackled by several pressing technical issues. For instance, the intricate nature of molecular devices often leads to complications in consistency and reliability during production. The minute size of these devices can make it challenging to conduct precise manipulations, resulting in variations that might compromise performance.
Furthermore, the integration of M3 devices with existing technologies can present significant compatibility hurdles. As researchers strive to bridge the gap between conventionally used components and novel molecular solutions, device performance may not reach its intended height. It’s like trying to fit a square peg in a round hole; the dimensions and specifications often clash, leading to inefficiencies.
Another concern is the limitation in the scalability of fabrication techniques. While small-scale production may yield impressive results, transitioning to mass production without sacrificing quality is a whole different ballgame. It’s akin to crafting a beautiful painting in a tiny frame; once the frame expands, maintaining the same artistry becomes a challenge.
Cost-Effectiveness and Accessibility
Cost is another beast that demands attention when discussing M3 technologies. Developing these advanced molecular devices often comes with a hefty price tag. The resources required for precise fabrication methods, including specialized materials and equipment, can be steep. As a result, the question of cost-effectiveness looms large in the arena of research and application.
Making these technologies accessible to a broader audience presents another layer of complexity. As costs mount, funding becomes a requisitory challenge. It’s not just about research; it’s about ensuring that promising innovations can reach the market. For students, educators, and professionals alike, narrowing that financial gap is crucial.
Additionally, regulatory hurdles can further drive up prices. Compliance with safety and environmental standards is paramount, and fulfilling these requirements can demand a significant investment of time and money.
The path to innovation is often lined with obstacles; it's how we address these challenges that determines our success.
Identifying these limitations is not merely an academic exercise but a crucial step toward the next wave of breakthroughs in molecular devices, paving the way for an era where advancements are viable and widely implemented.
Future Directions in M3 Research
The landscape of molecular devices, especially M3 technologies, is continuously transforming as researchers push boundaries. Understanding future directions in M3 research is not merely an academic exercise; it holds the promise of significant advancements across various sectors. As we look forward, emphasis is placed on potential innovations and the essence of interdisciplinary collaborations for driving progress.
Potential Innovations and Improvements
A key focus for the future of M3 technologies is the drive towards potential innovations that could overhaul existing paradigms. Research teams are exploring materials that exhibit enhanced performance characteristics. For instance, utilizing nanostructured materials might enhance conductivity and decrease energy loss. Other focal points include:
- Enhanced efficiency in energy conversion and storage, particularly concerning solar cells and batteries.
- Development of biocompatible molecular devices aimed at medical applications—this could revolutionize drug delivery systems, making them quicker and more effective.
- Novel self-assembling techniques to create complex structures at the molecular level, addressing the scalability issues that current manufacturing processes face.
These innovations not only improve device functionalities but also promise environmentally friendly approaches by using sustainable materials and processes.
"The advancement of M3 technology hinges on our ability to innovate and adapt to ever-changing scientific landscapes."
Interdisciplinary Collaborations for Advancements
While individual research can yield compelling results, the true potential of M3 technologies lies in collaborative efforts across various fields. The synthesis of knowledge from chemistry, physics, biology, and engineering is crucial.
- Collaboration between chemists and engineers can facilitate the design of better fabrication methods, while insights from biology can inspire novel applications in healthcare-saving technologies.
- Establishing partnerships with industry leaders enables research to transition from the lab to market, ensuring that these technologies not only exist as concepts but become viable products.
- Academic institutions can lead the way by fostering environments where cross-disciplinary research can flourish. For instance, linking researchers who specialize in nanotechnology with those in renewable energy can generate hybrid solutions that have far-reaching impacts.
Case Studies of Successful M3 Implementations
The realm of molecular devices, particularly with the advances encapsulated by M3 technologies, stands as a testament to the feats that scientific ingenuity can achieve. Case studies of successful implementations not only showcase the potential of M3 devices but also illuminate the paths forged through practical application. By dissecting these instances, we gain insights into the benefits, challenges, and innovations that define this exciting field.
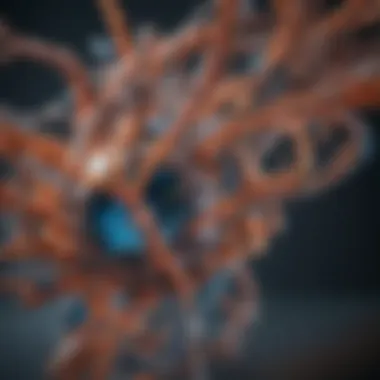
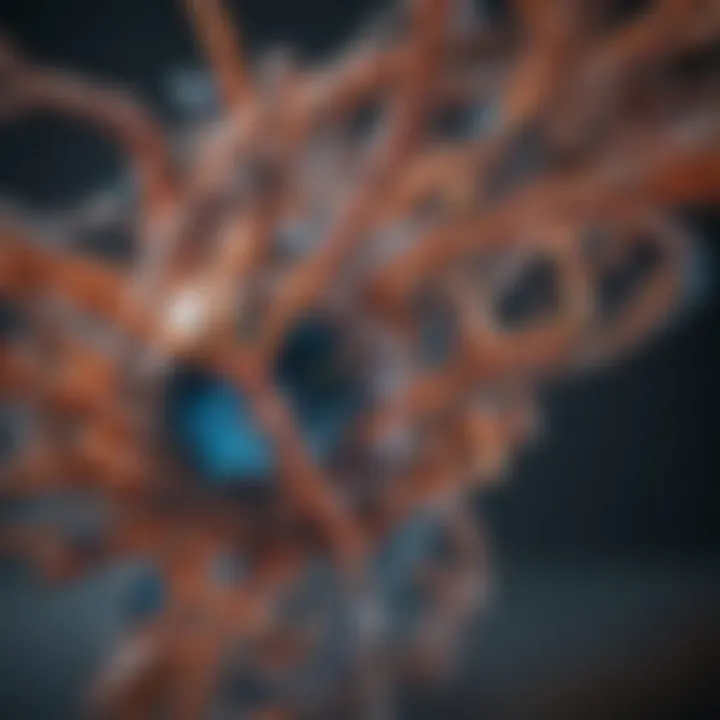
Prominent Research Institutions and Initiatives
Numerous research institutions across the globe have spearheaded initiatives aimed at M3 technology innovations. For instance, the Massachusetts Institute of Technology has launched a paradigm-shifting program focused on molecular electronics. This initiative improves the development of nanoscale devices that offer greater efficiency than traditional counterparts.
Moreover, Stanford University is also at the forefront. Their ongoing project investigates how M3 devices can integrate seamlessly with biological systems. The findings are promising, pushing the boundaries of what molecular devices can achieve not only in technology but also in health sectors.
Key components of successful research initiatives include:
- Collaboration: Various teams bringing together diverse expertise.
- Funding: Securing adequate resources often determines the reach of projects.
- Publication: Sharing results to stimulate further research and public interest.
Impact on Global Research Community
The successful case studies in M3 technology ripple beyond just individual accolades; their implications resonate throughout the global research community. These successes pave the way for new standards, ensuring that methodologies are evaluated and, when needed, redefined. Plus, they encourage an open dialogue between researchers, corporations, and the public.
What’s crucial to understand is how these implementations inspire a collective drive toward progress:
"Innovation thrives on shared knowledge and collaboration."
In a practical sense, the influence of successful M3 studies fosters:
- Increased Investment: As successful cases emerge, funding agencies and venture capitalists become more willing to invest in research that shows tangible benefits.
- Educational Curricula: Universities and colleges are adapting their programs, ensuring that students become adept in the latest technologies and theories.
- Interdisciplinary Guidelines: As M3 technology straddles multiple fields—biochemistry, materials science, and electrical engineering—adopting interdisciplinary frameworks is becoming essential.
Through these paths, major breakthroughs become communal victories. As the global community evaluates and absorbs the insights yielded from M3 implementations, the collective journey becomes richer, accentuating the power of combined efforts in scientific progress.
Ethical Considerations in Molecular Device Development
The development of molecular devices, while brimming with potential, also comes with a host of ethical considerations that cannot be overlooked. As the M3 technologies march forward, understanding these considerations is vital. This section focuses on the environmental impact, sustainability, and safety concerns surrounding the applications of these cutting-edge devices. By unpacking these components, we can grasp the broader implications of M3 technologies in our society.
Environmental Impact and Sustainability
Molecular devices can significantly impact our environment, both positively and negatively. On the one hand, these innovations offer solutions to pressing ecological challenges, such as energy efficiency and pollution reduction. For instance, certain molecular devices can be integrated into renewable energy systems, enhancing their efficiency and decreasing dependency on fossil fuels.
However, we must also consider the ramifications of their production and disposal. Many materials used in molecular devices can be harmful to the environment if not properly managed. For example, the synthesis of these devices often involves chemicals that require careful handling and disposal processes. Neglect in this area could lead to soil or water contamination, not to mention the health hazards posed to workers in manufacturing settings.
Here are key points to think about when considering the environmental impact of molecular devices:
- Resource Extraction: The journey begins with the sourcing of raw materials. Some materials are rare, leading to habitat destruction or unethical mining practices.
- Energy Consumption: The energy needed in the fabrication process can contribute to carbon footprints unless sustainable practices are implemented.
- End-of-Life Issues: Proper disposal and recycling frameworks must be in place to mitigate potential environmental hazards.
By prioritizing sustainability from the onset, researchers and developers can ensure that the benefits of M3 technologies do not come at an unacceptably high cost to our planet.
Safety Concerns in Applications
The application of molecular devices extends into numerous fields, including medicine, nanotechnology, and electronics. While their potential to revolutionize these sectors is clear, safety concerns must take precedence. For any new technology, a careful risk-benefit analysis is crucial. Risks could stem from unintended toxicity or malfunction in the devices, which may have serious repercussions based on their applications.
Applications in areas such as drug delivery or health diagnostics pose particular challenges. For example, if a molecular device interacts negatively with biological tissues or causes adverse reactions, the consequences could be dire. Thus, rigorous testing protocols and ethical guidelines must inform the development process.
Considerations around safety include:
- Toxicological Assessment: Ensuring that materials are safe for both humans and the environment is paramount.
- Regulatory Compliance: Adhering to existing regulations and standards lays the groundwork for safe product deployment.
- Transparency: Clear communication regarding potential risks and benefits to stakeholders helps build trust and prepares for informed decision-making.
In closing, the ethical landscape in the realm of molecular devices is complex yet critical. Integrating environmental responsibility and safety considerations into M3 technology development shapes not only public perception but also the long-term viability of these innovations. Managing these ethical dimensions as the technology evolves will be essential in fostering a responsible path forward.
Epilogue: The Road Ahead for M3 Technologies
As we round off our exploration into M3 technologies, it becomes crucial to acknowledge the broader trajectory of molecular devices. This conclusion serves as a reminder of the transformative potential inherent in these innovations. M3 devices aren't merely the latest trend; they represent a paradigm shift poised to redefine the landscape of science and technology. The implications of these advancements cut across multiple disciplines, fostering not just incremental improvements but also revolutionary breakthroughs.
Summary of Key Insights
Throughout the discourse, several pivotal themes have emerged:
- Multifunctionality: M3 molecular devices exemplify versatility, functioning in fields ranging from healthcare to energy. This adaptability extends their applicability significantly.
- Innovative Fabrication: Various synthesis methods have been uncovered. These methods not only streamline production but could lower costs and enhance scalability, making the technology more accessible.
- Interdisciplinary Collaboration: The fusion of chemistry, physics, and engineering is essential. Current challenges require insights from multiple fields, paving the way for new methodologies and applications.
In summary, M3 technologies exhibit unique qualities that position them for profound impact. Their ability to interface with a range of scientific inquiries allows them to serve as critical tools in tackling pressing contemporary issues.
Final Thoughts on Interdisciplinary Research
Looking ahead, the road for M3 technologies is dotted with both promises and challenges. Researchers and professionals must gird themselves for cooperative engagement across disciplines. No single field holds the answers; it’s about pooling knowledge and sharing expertise. For example, chemists collaborating with material scientists can lead to new insights in device functionality that neither could achieve alone.
Moreover, the dialogue between academia and industry is more crucial than ever. Such partnerships could catalyze the practical application of M3 technologies, pushing them from theoretical frameworks to tangible impacts in society.
"The future of M3 technologies hinges not just on scientific discovery but on our ability to connect the dots between different disciplines."