Exploring Lipid Polarity and Its Biological Impact
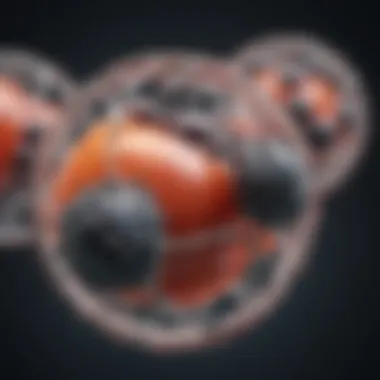
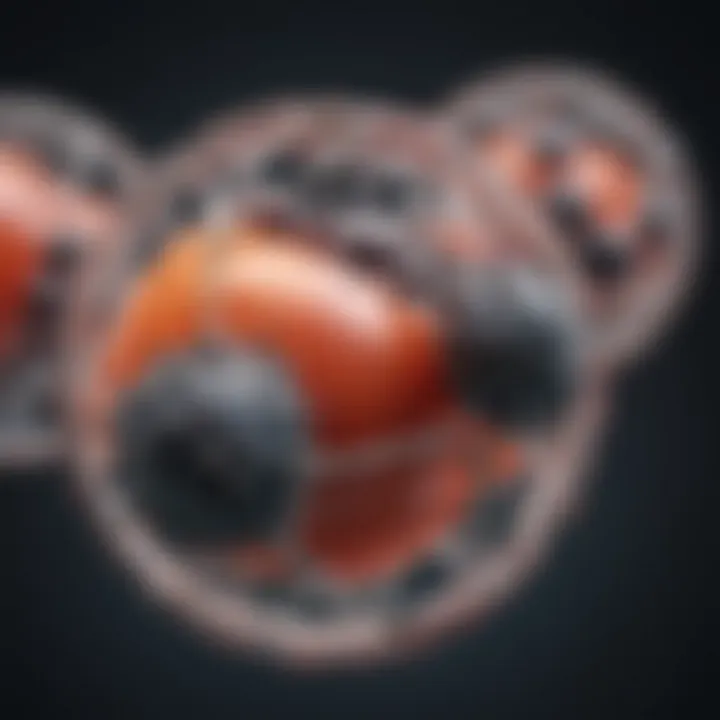
Intro
Lipid polarity is a fundamental aspect that shapes the intricate architecture of biological cells. At its core, polarity in lipids refers to the distribution of electrical charges within the molecules, which heavily influences their behavior within cellular structures and interactions. The concept is not just an abstract scientific principle but is deeply intertwined with the very fabric of life itself and its various biological processes.
In biological membranes, lipids are arguably the most crucial players, acting as barriers and facilitators. They ensure that environments within and outside of cells are distinctly maintained. This article unpacks the layers of lipid polarity by examining its characteristics, interactions in diverse environments, and the implications for health and disease.
Lipid Types and Their Roles
Understanding lipid polarity also involves delving into the different classes of lipids – phospholipids, triglycerides, steroids, and more. Each type carries its unique properties that determine how they interact with water and other biomolecules. For instance, phospholipids, which contain both hydrophobic tails and a hydrophilic head, play a pivotal role in forming cellular membranes, maintaining structural integrity, and facilitating communication between cells.
The health impacts of lipid polarity are increasingly evident in today’s research landscape. Dysregulation of lipid balance can lead to metabolic diseases, cardiovascular disorders, and even neurodegenerative conditions. Thus, grasping the details of lipid polarity extends beyond cellular biology and touches upon public health.
Overall, the complexities of lipid polarity establish a bridge between theoretical science and practical applications. Through this article, we aim to enhance understanding and inspire fresher avenues of research into the dynamic and critical role lipids play in biological systems.
Foreword to Lipid Polarity
Understanding lipid polarity is not just an academic exercise; it forms the bedrock of many biological processes. Lipids are a diverse group of hydrophobic organic molecules that play crucial roles in cellular structure and function. Their polarity, or lack thereof, significantly impacts how they interact with each other and with other cellular components. This section aims to unpack the concept of lipid polarity, highlighting its implications in various biological contexts.
In recent years, lipid polarity has gained traction in scientific discussions because it informs our understanding of membrane dynamics, signaling pathways, and even disease processes. By dissecting lipid polarity, researchers find ways to manipulate lipid interactions and functions, leading to advancements in drug design and therapeutic interventions. Thus, this exploration is far more than theoretical; it can lead to tangible benefits in health and disease management.
Definition of Lipid Polarity
Lipid polarity refers to the distribution of electrical charge within a lipid molecule, influencing its interaction with water and other molecules. Lipids can broadly be divided into polar and non-polar categories. Polar lipids typically possess hydrophilic (water-attracting) heads and hydrophobic (water-repelling) tails, allowing them to form structures like lipid bilayers. This intrinsic property is essential for creating cellular membranes that protect and compartmentalize biological activities.
The balance between hydrophilic and hydrophobic domains dictates a lipid's function. For instance, phospholipids—comprising a hydrophilic phosphate head and two hydrophobic fatty acid tails—are crucial in forming bilayers that constitute cell membranes. Without this polarity, life as we know it wouldn't exist, emphasizing how fundamental lipid polarity is to biology.
Historical Context
The understanding of lipid polarity is rooted in decades of research, beginning in the early 20th century when scientists first began to categorize lipids based on their solubility in organic versus aqueous solvents. This rudimentary classification set the stage for more sophisticated explorations of lipid behavior.
In the latter half of the century, advancements in biochemistry and molecular biology deepened the insights into lipid structures. Pioneering studies established the significance of lipid bilayers, underscoring how lipid polarity was pivotal for membrane formation and function. By the 1990s, researchers were investigating the role of lipid polarity in disease contexts, realizing that alterations in lipid composition could be linked to metabolic disorders, cardiovascular diseases, and even some cancers. This historical journey underscores the evolution of lipid science from simple classification to a sophisticated understanding that now informs clinical applications.
Classification of Lipids
Understanding the classification of lipids is crucial for grasping their diverse roles in biological systems. By categorizing these essential biomolecules, we can better appreciate their structural and functional differences. This section aims to illuminate the significance of lipid classification, emphasizing how each category contributes uniquely to cellular processes. Such a framework allows researchers, educators, and students to form a coherent mental model of lipid behavior in various environments.
Simple Lipids
Simple lipids are often referred to as the foundational building blocks in the lipid family. These lipids primarily comprise triglycerides, which are formed from one glycerol molecule bonded to three fatty acids. They are particularly important for energy storage, given their ability to efficiently store a high amount of energy per gram. When the body requires energy, hormones signal the mobilization of these triglycerides, converting them back into fatty acids and glycerol for oxidation.
Moreover, simple lipids serve a critical function in the insulation and protection of organs. For example, the adipose tissue, which is rich in triglycerides, cushions vital organs against mechanical shock. This characteristic showcases how simple lipids influence overall physiology beyond just energy metabolism.
Complex Lipids
Complex lipids, on the other hand, play multifaceted roles within cell membranes. This category includes phospholipids and glycolipids, both having unique structural arrangements that contribute considerably to membrane dynamics.
Phospholipids have a hydrophilic head and two hydrophobic tails, allowing them to naturally form bilayers in aqueous environments. This arrangement is fundamental to the structural integrity of cellular membranes. Such membranes regulate the movement of substances in and out of the cell and play pivotal roles in signaling pathways.
Glycolipids, which incorporate carbohydrates, not only assist in maintaining membrane stability but also participate in cellular recognition processes. The presence of these molecules on the cell membrane can influence communication between cells, playing a role in immune responses and tissue formation. Overall, complex lipids are indispensable for membrane structure and function.
Derived Lipids
Derived lipids encompass a varied array of compounds, primarily consisting of fatty acids and alcohols. These lipids arise from the modification of simple and complex lipids and can be categorized into two major groups: sterols and fat-soluble vitamins.
Sterols, like cholesterol, are vital structural components of cell membranes. Cholesterol's unique structure allows it to insert itself between phospholipids, affecting membrane fluidity, making it more rigid or fluid depending on the surrounding environmental conditions. This modulation is crucial for maintaining membrane functionality under varying temperatures.
Fat-soluble vitamins, such as vitamins A, D, E, and K, also fit into this category. Their lipid-soluble nature dictates their absorption and transport within the body, contributing numerous biological functions. For instance, vitamin D is critical for calcium metabolism, reflecting the sophisticated interplay of derived lipids in health.
"Lipid classification serves not just as a fundamental concept but as a gateway to the intricate interplay of life at the cellular level."
These classifications allow researchers to delve deeper into the implications of each lipid type, creating a rich tapestry that links lipid behaviors to physiological outcomes.
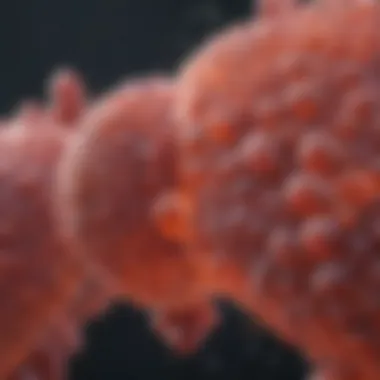
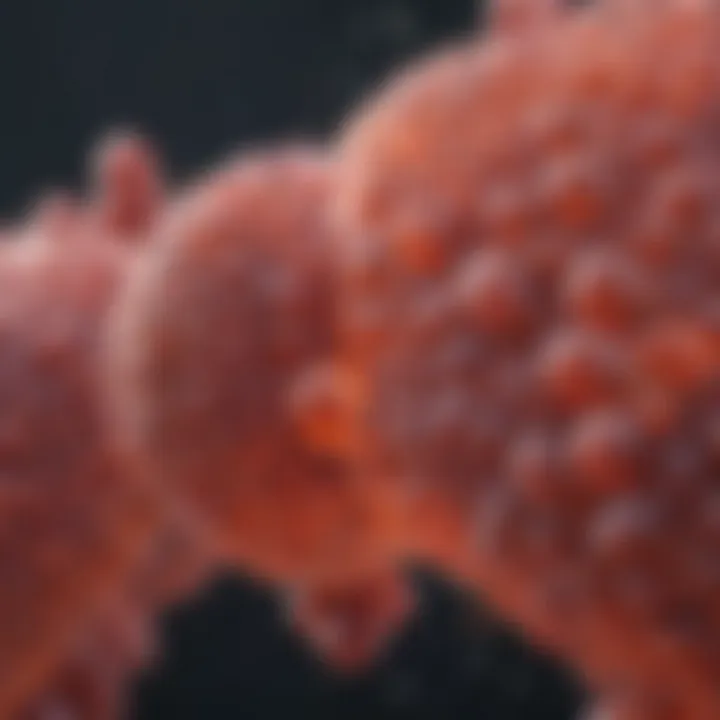
Structural Characteristics of Lipids
Lipid polarity fundamentally shapes the function and behavior of cellular membranes. Understanding the structural characteristics of lipids is key to appreciating their roles in biological systems. These structures not only dictate how lipids interact with water and other molecules but also directly influence physiological processes. By diving into specific elements like tail composition, head structure, and saturation levels, one can unravel the complexities of how lipids contribute to cellular integrity and fluidity.
Hydrophobic Tail vs. Hydrophilic Head
Lipids are unique due to their amphipathic nature, meaning they possess regions that are hydrophobic (water-repelling) and hydrophilic (water-attracting). The hydrophilic head, typically made up of a glycerol or phosphate group, can interact favorably with water molecules.
In contrast, the hydrophobic tail, often comprised of long fatty acid chains, avoids contact with water. This structural variability is crucial; it leads to the formation of lipid bilayers, where hydrophilic heads face the aqueous environment while the hydrophobic tails are sequestered away. This natural arrangement is fundamental for cell membranes, providing both a barrier to unwanted substances and a medium for various biochemical reactions.
"The crucial balance between hydrophobic and hydrophilic properties defines lipid behaviors and dictates the functionality of biological membranes."
Fatty Acid Composition
The specific makeup of fatty acids within lipids is another fundamental aspect influencing membrane characteristics. Fatty acids can vary in length and in the number of double bonds they contain. They can be saturated, meaning every carbon atom is bonded to the maximum number of hydrogen atoms, or unsaturated, which introduces one or more double bonds between carbon atoms.
- Saturated Fatty Acids: Tend to pack tightly together, leading to less fluid membranes.
- Unsaturated Fatty Acids: Reintroducing kinked structures, preventing tight packing and resulting in more fluid membranes.
The ratio of saturated to unsaturated fatty acids profoundly impacts membrane flexibility, with implications for how cells adapt to varying temperatures and metabolic demands.
Saturation Levels and Their Impact
Saturation levels are pivotal in determining lipid functionality. Saturated lipids will maintain a more rigid structure, giving stability and strength to membranes, yet potentially limiting their fluidity. On the other hand, a higher concentration of unsaturated fats can enhance the fluidity of the membrane, allowing for more dynamic interactions and better accommodation for various proteins that reside within or pass through the lipid bilayer.
The membranes’ fluidity is critical because it affects not only permeability but also the efficiency of protein function and the ability of the membrane to facilitate signal transduction. Changes in saturation levels can arise from dietary intake, impacting overall health and the progression of certain diseases. Understanding these relationships opens avenues for potential therapeutic strategies focused on lipid nutrition or alterations in fatty acid composition for health benefits.
Lipid Bilayers and Membrane Formation
The formation of lipid bilayers is pivotal in understanding the cell membrane's architecture and its functional implications. Lipid bilayers create a selectively permeable barrier that regulates what enters and exits a cell, serving not only as a boundary but also as a dynamic environment for various biochemical reactions. The interplay between different lipid types, their structural characteristics, and polarity is crucial in maintaining the integrity and functionality of cellular membranes.
Mechanisms of Bilayer Assembly
Lipid bilayer assembly is a fascinating process driven primarily by the inherent properties of lipids. When lipids are placed in an aqueous environment, they spontaneously arrange into bilayers with hydrophobic tails facing inward and hydrophilic heads pointing towards the aqueous surroundings. This self-assembly mechanism can be broken down into several key steps:
- Hydrophobic Effect: The primary driving force behind bilayer formation is the hydrophobic effect. Lipid tails avoid contact with water, leading them to aggregate and minimize their exposure to the aqueous surroundings.
- Liposome Formation: As the assembly progresses, lipids naturally arrange into spherical structures known as liposomes, which can encapsulate aqueous solutions inside.
- Nucleation Sites: Certain lipids create favorable nucleation sites, which help in the growth of bilayers through lateral diffusion and fusion of lipid molecules.
- Thermodynamic Stability: The final bilayer structure reaches a state of thermodynamic stability, influenced by the types of lipids involved, their chain lengths, and saturation levels. Consequently, this determines the bilayer's thickness and fluidity.
This self-assembly is essential for creating lipid bilayers with particular properties tailored to specific cellular functions. In multicellular organisms, variations in lipid composition can lead to diverse membrane characteristics that cater to different cell types.
Role of Lipid Polarity in Membrane Structure
Lipid polarity directly influences the structure and function of membranes. The polarity of lipid molecules allows them to interact in ways that are critical for cellular activities. Here are some core aspects:
- Bilayer Integrity: Polar lipids, such as phospholipids, contain hydrophobic tails and hydrophilic heads, which create a stable bilayer. This arrangement is vital for maintaining membrane integrity under various environmental conditions.
- Fluidity: The polarity affects lipid packing in the bilayer. A higher proportion of unsaturated fatty acids in lipids leads to increased fluidity. In contrast, saturated acids tend to pack more closely, reducing fluidity.
- Mosaic Model: The fluid mosaic model of membrane structure posits that lipid bilayers accommodate a variety of proteins that float within, anchored by their interactions with polar heads. This interaction is critical for membrane functionality, impacting cell signaling and transport processes.
- Transmembrane Proteins: Proteins in the membrane interface differently based on their own polarity. Integral proteins, which span the membrane, often have hydrophobic regions that interact with the lipid bilayer, while hydrophilic regions extend into the aqueous environment.
"The intricate dance between lipid polarity and membrane structure is an essential aspect of fluid dynamics in biological systems, impacting everything from nutrient transport to cell signaling."
The understanding of lipid bilayers and their assembly mechanisms is pivotal for grasping how cells maintain homeostasis in fluctuating environments, responding effectively to varied stimuli while ensuring vital biochemical functions are preserved.
Functional Implications of Lipid Polarity
Lipid polarity plays a pivotal role in various biological functionalities that are central to cellular operations. It influences interactions within cellular membranes and affects numerous physiological processes. As fundamental components of membranes, lipids ensure these structures maintain their structural integrity while allowing necessary permeability for ions and molecules. Understanding lipid polarity reveals layers of complexity that are critical to both cellular processes and potential therapeutic applications.
Membrane Fluidity and Permeability
Lipid polarity significantly contributes to membrane fluidity and permeability. Fluidity refers to the viscosity of the lipid bilayer, which affects how easily proteins and other components can move within the membrane. Think of a fluid membrane like a well-oiled machine—when everything moves smoothly, it functions optimally.
Factors that influence this fluidity include:
- Lipid composition: The types of lipids present, such as phospholipids or cholesterol, determine how tightly they pack together and their overall fluid nature.
- Temperature: Higher temperatures tend to increase fluidity, making membranes more permeable due to increased kinetic energy.
- Degree of saturation: Saturated lipids pack closely together, reducing fluidity, while unsaturated ones introduce kinks that enhance mobility.
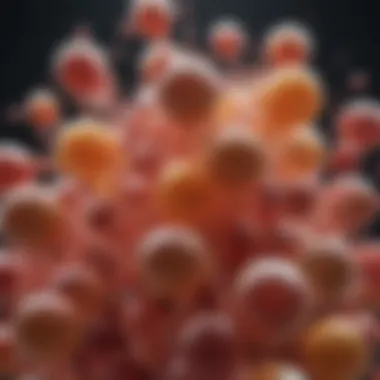
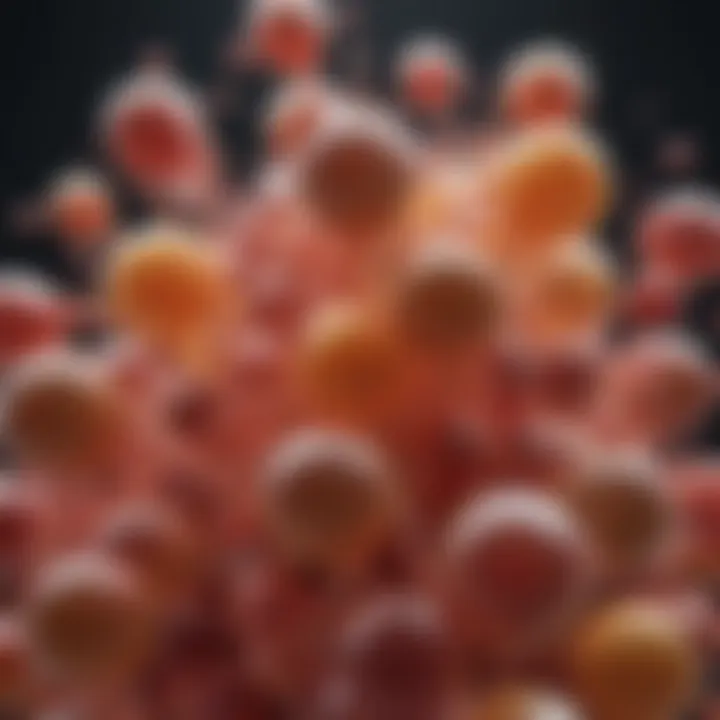
These factors underscore the dual role of lipid polarity—where an increase in unsaturation can enhance membrane permeability, thus permitting the passage of essential nutrients and signaling molecules.
"An optimal balance in lipid composition is crucial for maintaining cellular function. Excessive fluidity can lead to leakiness, while overly rigid membranes can hinder transport processes."
Signal Transduction Pathways
The relationship between lipid polarity and signal transduction pathways is another area of relevance. Lipids are not just structural; they serve as critical signaling molecules that trigger various biological responses. In these pathways, specific lipid molecules can act as secondary messengers that relay signals from membrane receptors to internal cell structures.
Key points regarding this interaction include:
- Phosphatidylinositol: This polar lipid plays a crucial role in signal transduction, particularly in cellular responses to growth factors. Upon receptor activation, it is phosphorylated to form inositol trisphosphate, which acts as a secondary messenger.
- Lipid rafts: These microdomains within membranes are enriched in cholesterol and sphingolipids, providing a unique environment for the clustering of proteins involved in signal transduction.
Lipid polarity is instrumental in determining which molecules can navigate these pathways and how effectively these signals can be transmitted within the cell.
Interaction with Environmental Factors
Lipid polarity does not exist in a vacuum. It interacts dynamically with a variety of environmental factors that can significantly influence its characteristics and behavior. Understanding these interactions is essential for comprehending how lipids function within biological systems and how they respond to changes in their surroundings. Two primary aspects are paramount: the effects of pH and temperature, and the role of solvent polarity. Each of these elements brings its own set of considerations and benefits that can dramatically alter lipid behavior.
Influence of pH and Temperature
The relationship between lipids and environmental factors, particularly pH and temperature, is crucial. Lipids can behave differently depending on the acidity or basicity of their environment. For instance, certain phospholipids may undergo changes in their overall charge under different pH levels, which in turn impacts their interaction with surrounding water molecules and other lipids. This can lead to variations in membrane fluidity and stability.
Temperature, on the other hand, is a critical element that influences lipid state. Increased temperatures can cause lipid bilayers to transition from gel to liquid-crystalline states, making membranes more fluid. Conversely, lower temperatures can cause lipids to pack more tightly, leading to reduced membrane permeability, which can affect the function of membrane proteins and cellular processes.
For a clear understanding, consider these points:
- Lipid membranes become more permeable at higher temperatures due to increased fluidity.
- Acidic environments can protonate lipid head groups, leading to altered interactions with proteins.
- Membrane rigidity at low temperatures can hinder cellular functions crucial for survival.
Effect of Solvent Polarity
In addition to pH and temperature, solvent polarity plays a pivotal role in lipid interactions. The polarity of solvents can modulate lipid solubility, stability, and their ability to form bilayers. For example, lipids are generally more soluble in nonpolar solvents compared to polar solvents, effectively influencing their behavior in various biological processes.
When lipids are exposed to a polar solvent, such as water, they tend to arrange themselves into structures that minimize exposure to the aqueous environment. This behavior is fundamental in forming biological membranes, a sophisticated cellular boundary vital for maintaining homeostasis.
Lipid interactions with solvents can determine their biological function, affecting how they participate in membrane assembly and signaling.
In summary, lipid polarity interacts with environmental conditions extensively. pH levels and temperature impact lipid behavior and functionalities, whereas solvent polarity dictates the solubility and arrangement of lipids. A well-rounded understanding of these factors allows researchers and professionals to appreciate the complexities of lipid behavior in biological contexts.
Lipid Polarity in Health and Disease
Lipid polarity plays a critical role in nearly every aspect of human health and disease. Understanding this can give insight into how disturbances in lipid metabolism can lead to pathological conditions. The polarity of lipids influences cellular membranes, energy storage, and even the efficacy of drug delivery systems.
Lipid Metabolism and Disorders
The metabolism of lipids is a complex series of biochemical processes that are crucial for maintaining cellular function. An imbalance in lipid metabolism can lead to various disorders, such as obesity, cardiovascular diseases, and diabetes. For instance, the accumulation of certain lipid species can foster inflammatory pathways and metabolic dysfunction.
Key considerations include:
- Dyslipidemia: This is characterized by abnormal levels of lipids in the blood. High levels of low-density lipoproteins (LDL) coupled with low levels of high-density lipoproteins (HDL) can contribute to atherosclerosis.
- Fatty Liver Disease: In this condition, excess lipids accumulate in liver cells. It’s often linked to high insulin levels and metabolic syndrome.
The importance of lipid polarity in these contexts can't be overstated. Polar lipids, such as phospholipids, are fundamental in forming membranes that compartmentalize metabolic processes. Disruption of these membranes can lead to cell stress and eventual cell death. Thus, monitoring lipid profiles and maintaining lipid homeostasis could be the keystone in preventing these disorders.
“The balance of lipids in our body is not just a matter of weight; it’s about maintaining cellular integrity and functionality.”
Lipid Polarity and Drug Delivery Systems
Lipid polarity is also paramount in the development of drug delivery systems. Many pharmaceutical compounds rely on lipids to navigate biological barriers and reach their targets effectively.
Here are some methods highlighting this relationship:
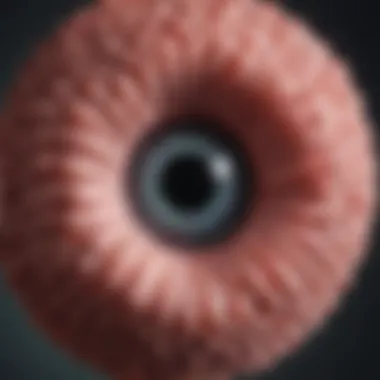
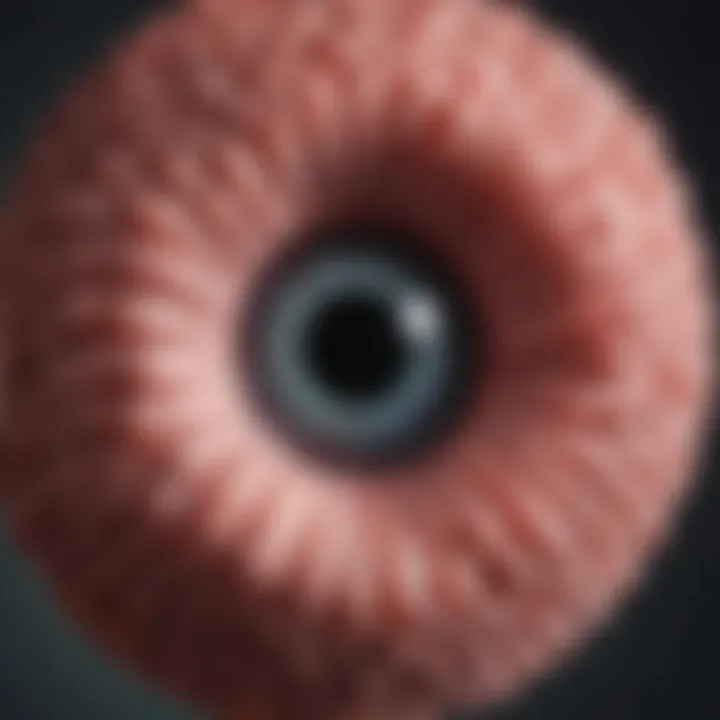
- Lipid Nanoparticles: These are often employed in delivering mRNA vaccines. Their lipid composition can enhance stability and facilitate cellular uptake.
- Micelles and Liposomes: These structures can encapsulate drugs, improving solubility and bioavailability, while protecting sensitive compounds from degradation.
The polarity of these lipid-based carriers can substantially affect their circulation time in the bloodstream, cellular uptake efficiency, and target specificity. Research demonstrates that tuning the polarity of lipid nanoparticles can improve therapeutic outcomes significantly, making lipid polarity a vital factor in drug formulation and delivery. Thus, a nuanced comprehension of lipid polarity can lead to innovative strategies in treating diseases and improving health outcomes.
Research Advances in Lipid Studies
In recent years, research into lipid studies has witnessed remarkable growth, reflecting the increasing recognition of lipids as key players in biological systems. The exploration of lipid polarity opens doors to understanding their diverse roles in cellular functioning and disease. Advances in this field are not just academic; they hold the promise of significant developments in biotechnology, pharmacology, and medical research.
One of the fundamental reasons for the surge in interest is the discovery of novel lipid species and their specific functions. As we peel back the layers surrounding lipid complexity, the implications for metabolic disorders, cancer, and cellular signaling pathways are becoming clearer. Consequently, keeping abreast of these developments helps in identifying potential therapeutic targets and novel strategies to tackle various health conditions.
"The understanding of lipid profiles in diseases can lead to early diagnosis and targeted treatment strategies."
Emerging Techniques in Lipid Analysis
The progress in lipid analysis techniques has been groundbreaking, paving the way for comprehensive lipidomics. Techniques such as mass spectrometry and nuclear magnetic resonance (NMR) spectroscopy have taken center stage in lipid research, allowing scientists to achieve unparalleled resolution in lipid profiling. These methods enable the identification and quantification of lipids in biological samples with remarkable accuracy.
- Mass Spectrometry (MS): This technique enables the analysis of the mass-to-charge ratio of ions. In lipid studies, it helps in identifying lipid classes and structures, making it invaluable for profiling complex lipid mixtures.
- Nuclear Magnetic Resonance (NMR) Spectroscopy: This provides insights into the molecular structure and dynamics of lipids. It reveals how lipid molecules behave in different environments, such as in membranes or under stress.
Additionally, advances in chromatography, particularly liquid chromatography, have improved the separation of lipid species, providing clearer insights into their specific roles and interactions. These emerging techniques help researchers unravel the intricacies of lipid functions, shedding light on their contributions to health and disease.
Insights from Recent Studies
Recent investigations into lipid polarity and its associated biological functions have produced intriguing findings. One promising area is the relationship between lipid composition in membranes and their fluidity, affecting how cells communicate and react to their environments. For instance, studies have shown that alterations in membrane lipid profiles can lead to behavioral changes in cells, influencing processes like cell signaling and apoptosis.
Moreover, lipid metabolism disorders have been linked to a variety of conditions, including heart disease, obesity, and neurodegenerative disorders. By understanding these relationships, researchers are moving closer to developing effective interventions and preventive strategies.
In summary, the ongoing research advances in lipid studies are vital for clarifying the multifaceted roles of lipids within biological systems. As we refine our understanding and improve analytical methods, the potential to uncover novel insights that will guide better healthcare becomes more tangible. The path forward is riddled with opportunities for scientific exploration that promise to shape the future of medicine and health.
Future Directions in Lipid Polarity Research
The exploration of lipid polarity remains a vibrant area of study, ripe with potential for groundbreaking discoveries that could transform our understanding of cellular biology. As research progresses, it becomes increasingly clear that lipid polarity plays a pivotal role not only in fundamental biological processes but also in the development of therapeutic strategies that could address a variety of health concerns. This section focuses on the future directions in lipid polarity research, highlighting the importance of interdisciplinary approaches and the potential for novel therapeutics.
Interdisciplinary Approaches
Collaboration across different scientific disciplines is crucial for advancing our understanding of lipid polarity. By harnessing methodologies from biochemistry, molecular biology, and pharmacology, researchers can create a more comprehensive picture of how lipids function within cells.
- Biophysics: Techniques such as NMR spectroscopy and cryo-electron microscopy allow researchers to visualize lipid structures and dynamics. This visual perspective can unearth how specific lipid arrangements influence membrane behavior.
- Systems Biology: By integrating lipidomics with genomic and transcriptomic data, scientists may uncover intricate networks of lipid interactions that dictate cellular responses. This systems-level approach can be beneficial in elucidating complex signaling pathways that are modulated by lipid composition.
- Computational Modeling: The use of computer simulations can predict how changes in lipid polarity affect membrane properties. Such models can save time and resources in experimentation, providing a theoretical framework to guide empirical studies.
"The future of lipid research lies in the interplay between traditional methods and cutting-edge technology."
Potential for Novel Therapeutics
Another promising avenue lies in the potential for developing novel therapeutics based on insights gleaned from lipid polarity research. As scientists delve deeper into how lipids influence disease pathways, new avenues for drug development emerge.
- Targeted Drug Delivery: Understanding lipid polarity can lead to the design of lipid-based carriers that enhance the solubility and bioavailability of poorly soluble drugs. For instance, nanometer-sized lipid carriers can be engineered to deliver specific compounds directly to target cells, thus minimizing side effects and increasing therapeutic efficacy.
- Therapeutic Lipids: Compounds like omega-3 fatty acids, which possess unique polarity characteristics, are being investigated for their roles in anti-inflammatory responses. Research indicates that these lipids can modulate immune function and might be harnessed to create dietary supplements aimed at managing chronic diseases.
- Regenerative Medicine: The exploitation of lipid polarity in cell membrane formation has implications in stem cell therapy. Insights into lipid interactions may help optimize conditions for stem cell differentiation, leading to advancements in tissue regeneration.
In summary, the synergy between interdisciplinary research efforts and the drive for innovative therapeutics holds significant promise for the future of lipid polarity studies. As the scientific community continues to collaborate and explore, the discoveries made in this field are poised to reshape not only our theoretical understandings but also the practical applications in medicine and biotechnology.
End
Understanding lipid polarity is more than a mere academic exercise; it serves as a cornerstone of molecular biology, impacting everything from cellular integrity to drug design. By diving into the depths of lipid polarity, we uncover how complex lipid interactions contribute to the structural and functional attributes of biological membranes. Furthermore, this area of study sheds light on the implications of lipid behaviors in both health and disease, revealing how shifts in lipid composition can signal metabolic disorders or the efficacy of pharmaceutical interventions.
Summary of Key Insights
In summarizing the key insights gained from this exploration:
- Lipid diversity: Different classes of lipids, including simple, complex, and derived lipids, play unique roles in cellular functions. Each type is characterized by its own physical and chemical properties primarily influenced by its polarity.
- Bilayer dynamics: The organization of lipids into bilayers is fundamental to membrane structure, driven largely by the hydrophilic and hydrophobic nature of the lipid molecules. This arrangement facilitates vital processes such as selective permeability and fluidity, which are essential for cellular homeostasis.
- Biological relevance: The relationship between lipid polarity and various biological phenomena highlights the importance of lipid metabolism and its interplay with health conditions. Abnormal lipid metabolism can lead to serious diseases, underscoring the need for ongoing research in this arena.
This summary encapsulates the multifaceted aspects of lipid behavior and their implications in biological sciences, suggesting a profound interconnection between basic lipid chemistry and complex biological outcomes.
Implications for Future Research
Looking ahead, the implications stemming from our understanding of lipid polarity can pave pathways toward new areas of research. They include but are not limited to:
- Interdisciplinary collaborations: Research in lipid polarity could benefit from the integration of biological sciences, chemistry, and materials science to better understand lipid roles in more complex systems.
- Therapeutic avenues: The study of how lipid polarity affects drug delivery systems opens the door to discoveries in pharmacology. Innovations in lipid nanoparticles for targeted therapies could revolutionize how medications are developed and administered.
- Novel diagnostics: Further exploration of lipid profiles related to specific diseases could lead to breakthroughs in diagnostic methods, ideally allowing for earlier detection of metabolic disorders or other health-related issues.