Exploring Silicon: Properties, Uses, and Innovations
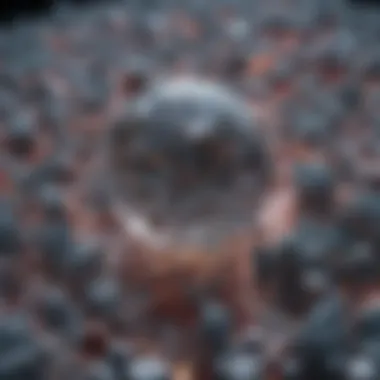
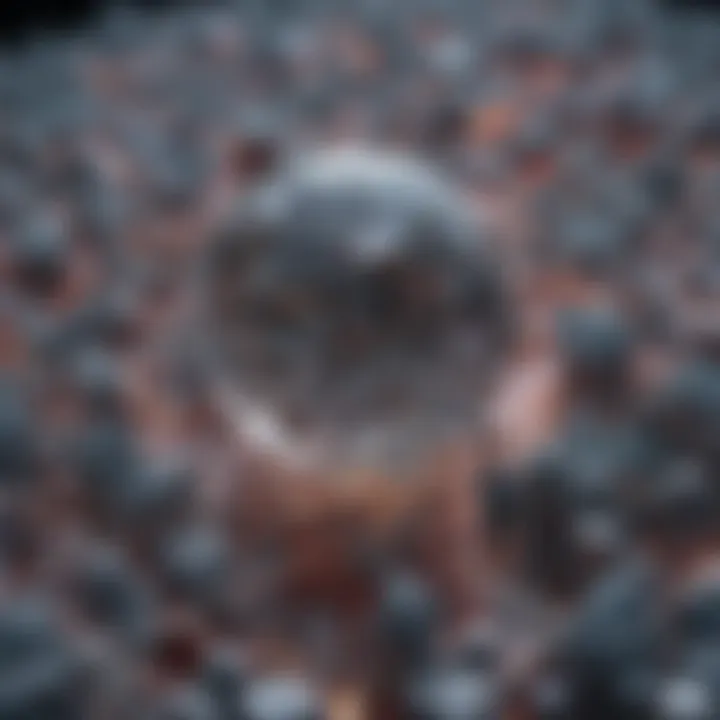
Intro
This article focuses on silicon, a versatile element found in various forms that serve different applications. Silicon has become integral in many scientific fields, including technology, biology, and materials science. By examining its properties and innovations, we aim to illustrate its multifaceted roles. This inquiry highlights silicon's significance in modern research and its future trajectory.
Methodology
Overview of research methods used
The research undertaken in this article involved a comprehensive literature review, analyzing both academic papers and industry reports. The approach ensured a balanced understanding of silicon in various contexts. Emphasis was placed on peer-reviewed articles sourced from reputable journals, as well as insights from recent patents to capture recent advancements.
Data collection techniques
Data was collected through online databases such as Google Scholar and ScienceDirect. Articles were selected based on keywords related to silicon's properties, applications, and innovations. Additionally, insights from platforms like Reddit and industry reports uncoveed contemporary discussions surrounding silicon applications.
Properties of Silicon
Silicon presents unique properties that contribute to its wide-ranging usability. It has a high melting point, makes it ideal for high-temperature applications. Its semiconducting ability allows it to be a backbone of electronics. Furthermore, silicon's chemical stability enhances its durability in various environments. More details on the properties are as follows:
- Thermal Conductivity: Ideal for thermal management in electronic devices.
- Electrical Conductivity: Essential for semiconductors.
- Mechanical Strength: Enables use in construction and manufacturing.
Silicon’s semiconducting properties have made it the foundation of the modern electronics industry.
Applications of Silicon
Silicon's applications are extensive. The element is pivotal in multiple sectors:
- Technology: Utilized in the production of computer chips, solar cells, and LED devices.
- Biology: Emerging roles in drug delivery systems and nutrient identification.
- Materials Science: Used in the development of advanced composites and ceramics.
Innovations continue to emerge in these domains, reflecting the element's adaptability to meet new challenges.
Future Directions
The study of silicon is continuously evolving. Several emerging research areas warrant attention:
- Sustainable Silicon Production: Innovations aiming to reduce environmental impacts associated with silicon mining.
- Advanced Semiconductor Technologies: Research into new alloys and compounds to enhance performance.
- Biocompatible Silicon Applications: Investigating silicon's function in biomedical instruments and therapies.
Further investigation is necessary to unravel silicon's potential in these burgeoning fields, ensuring both scientific and practical advancements.
In summary, silicon’s various forms are not only crucial for existing technologies but also essential for future innovations. Understanding its properties and applications will empower researchers, students, and professionals to harness its full potential.
Prologue to Silicon
The study of silicon is crucial to understanding its many applications in various fields such as technology, material science, and biology. Silicon is not only a fundamental element found in the Earth's crust, but it also plays a vital role in modern society. Its unique properties make it indispensable in the production of electronic devices, solar cells, and even in biological systems. The diverse forms of silicon, each with distinct characteristics, highlight the versatility of this element.
Understanding silicon allows researchers, educators, and professionals to innovate and optimize processes across disciplines. The continuous exploration of silicon’s properties has led to significant advancements, making it necessary for anyone involved in science or technology to be familiar with this element.
Through this article, we will examine the historical context, periodic table placement, and specific forms of silicon, providing a comprehensive overview of its significance in contemporary research and applications.
Historical Context of Silicon
Silicon was first isolated in 1824 by the Swedish chemist Jöns Jacob Berzelius. The understanding of silicon has evolved significantly since its discovery. Originally, silicon was regarded as a mere component in certain minerals. However, with the progression of the industrial revolution and advancements in chemistry, its importance unraveled, particularly with the advent of electronics in the 20th century. At the heart of this revolution, silicon emerged as a key material in semiconductor technology, leading to the development of transistors and integrated circuits.
As the demand for electronic devices surged, so did the research on silicon. Its properties of conductivity and thermal resistance made it the ideal choice for a wide range of applications. Understanding its historical significance, therefore, frames the current landscape of technology that relies heavily on silicon.
Silicon in the Periodic Table
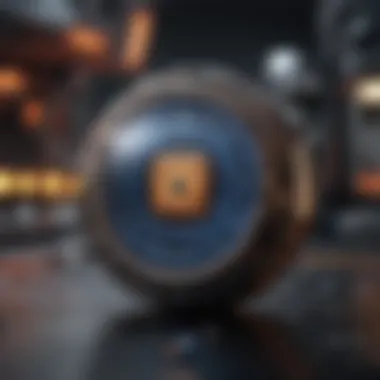
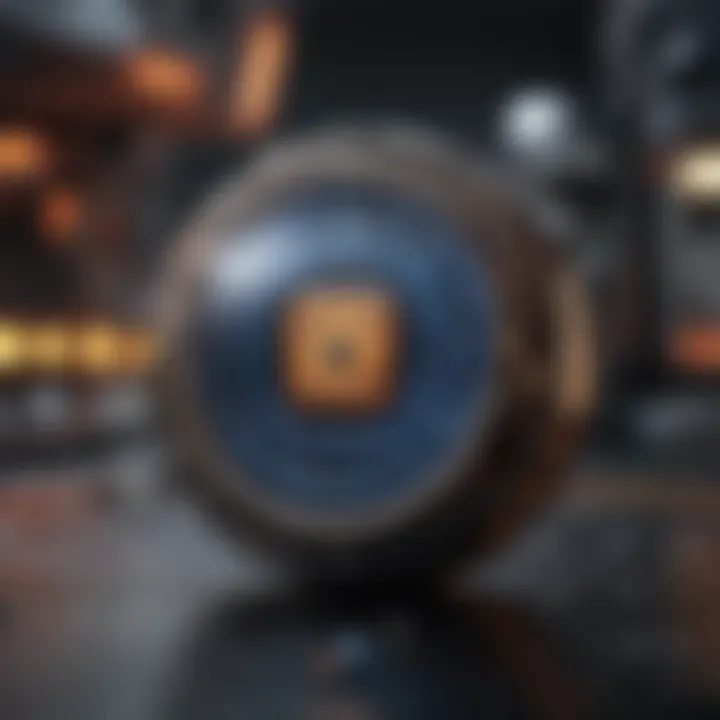
Silicon is located in group 14 of the periodic table and has the atomic number 14. It is a metalloid with properties that are in between metals and non-metals. Silicon typically exhibits a shiny appearance and is solid at room temperature. Its place in the periodic table indicates its capability to form covalent bonds with other elements, making it quite versatile.
The periodic classification is crucial in predicting how silicon will behave when combined with other elements. It can react with a range of non-metals such as oxygen and nitrogen, and these reactions have significant implications in creating various silicon compounds. This classification has direct relevance to the chemical properties and applications of silicon, leading to its use in everything from electronics to pharmaceuticals.
Forms of Silicon
Understanding the forms of silicon is essential as this element plays a crucial role across various fields, including electronics, renewable energy, and materials science. Each form of silicon has unique properties that influence its application and suitability for different technologies. For researchers and professionals, this knowledge informs the choice of materials in projects that demand specific characteristics. Moreover, insights into the advancements in these silicon forms could lead to improved efficiencies and innovations, making this examination particularly relevant in today's fast-paced tech environment.
Crystalline Silicon
Structure and Characteristics
Crystalline silicon has a well-ordered atomic structure, arranged in a repeating lattice pattern. This structured arrangement is a key factor that contributes to its electrical properties. The primary characteristic of crystalline silicon is its high purity, which enhances its effectiveness as a semiconductor. A unique feature of its structure allows for efficient electron movement, making it a popular choice for electronic applications. The advantages of crystalline silicon include its excellent conductive properties and stability, though it can be more expensive to produce compared to other forms of silicon.
Applications in Electronics
The applications of crystalline silicon in electronics are vast. It is the backbone of the semiconductor industry, widely used in the production of transistors and integrated circuits. The key characteristic here is its reliability and performance under various conditions, making it the preferred choice for high-tech devices. A unique feature that supports its dominance is its compatibility with existing manufacturing processes, allowing for mass production. However, the main disadvantage can be the costs involved in production and processing.
Amorphous Silicon
Properties and Uses in Solar Cells
Amorphous silicon lacks the long-range order found in crystalline structures. This characteristic allows for flexibility in applications, particularly in thin-film solar cells. A notable property of amorphous silicon is its ability to absorb sunlight efficiently, which is beneficial for solar energy conversion. The use of amorphous silicon in solar cells has risen due to its lower material costs and the simplicity of manufacturing. The unique feature of being lightweight and adaptable is also a significant advantage, although its lower efficiency compared to crystalline silicon can be seen as a limitation.
Challenges and Limitations
Challenges with amorphous silicon primarily involve its lower efficiency and stability compared to crystalline counterparts. A key limitation is the degradation of its photovoltaic performance over time, which raises concerns about longevity and reliability. This aspect is relevant for developers seeking long-term solutions. The unique feature of the material's high rate of deposition can be seen as an advantage, but when combined with its degradation issue, it may not provide the desired return on investment.
Polycrystalline Silicon
Manufacturing Processes
Polycrystalline silicon consists of numerous small silicon crystals and is often produced through processes like the Siemens method or the casting method. The key characteristic of these processes is cost-effectiveness, allowing for more scalable manufacturing compared to single-crystal silicon. A unique feature is the ability to produce polycrystalline silicon in large quantities, making it suitable for photovoltaic applications. However, one can argue that the presence of multiple grain boundaries can affect the electronic properties, leading to slightly lower performance in certain applications.
Sustainability Considerations
Sustainability is an important consideration when it comes to polycrystalline silicon. The manufacturing processes can have significant environmental impacts, particularly regarding energy consumption and materials used. The key characteristic that stands out is the potential for recycling silicon, which aligns with increasingly important sustainability practices. Unique features of advanced production methods, such as using less energy or employing renewable energy sources, can be beneficial for minimizing ecological footprints. Nonetheless, the initial environmental costs can be substantial, and ongoing improvements are necessary for broader acceptance in green technology.
Chemical Properties of Silicon
Silicon's chemical properties are fundamental in understanding its broad applications and relevance across technology and materials science. This section highlights the reactive nature of silicon and its behavior when combined with other elements. Such properties are crucial for researchers and engineers who work with silicon in various contexts. Understanding reactivity can lead to enhanced materials and innovate processes in semiconductor technology as well as other industrial applications.
Reactivity with Other Elements
Oxygen and Silicon Compounds
Silicon has a pronounced tendency to react with oxygen, forming silicon dioxide, which is a significant compound in nature and industry. Silicon dioxide is not just a key component of glass. It's also critical in the production of foundry sand and serves as a semi-conductor in electronics. The formation of compounds between oxygen and silicon is noteworthy because they demonstrate unique properties of hardness and thermal stability.
Moreover, silicon dioxide is abundant in the Earth's crust, making it easily accessible for various applications. One of the key characteristics of silicon-oxygen bonds is their strong covalent nature, which contributes to the durability of the resulting compounds. This characteristic makes silicon dioxide a resilient choice for many applications, particularly when high temperatures and harsh environments are involved.
Unique features of silicon compounds also include their insulating properties, making them effective in electronics and telecommunications as insulators in semiconductor devices. However, despite their numerous advantages, silicon-based compounds can sometimes be brittle, which limits their use in certain mechanical applications.
Silicon Alloys
Silicon alloys are another important aspect of silicon's chemical properties. These materials consist of silicon combined with various metals, such as aluminum and copper, to enhance their mechanical and electrical properties. The most significant aspect of silicon alloys is their ability to improve the strength and conductivity of metals, making them incredibly useful in construction and manufacturing sectors.
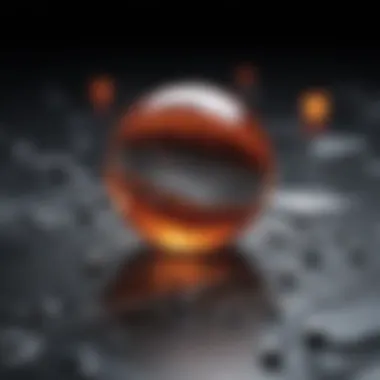
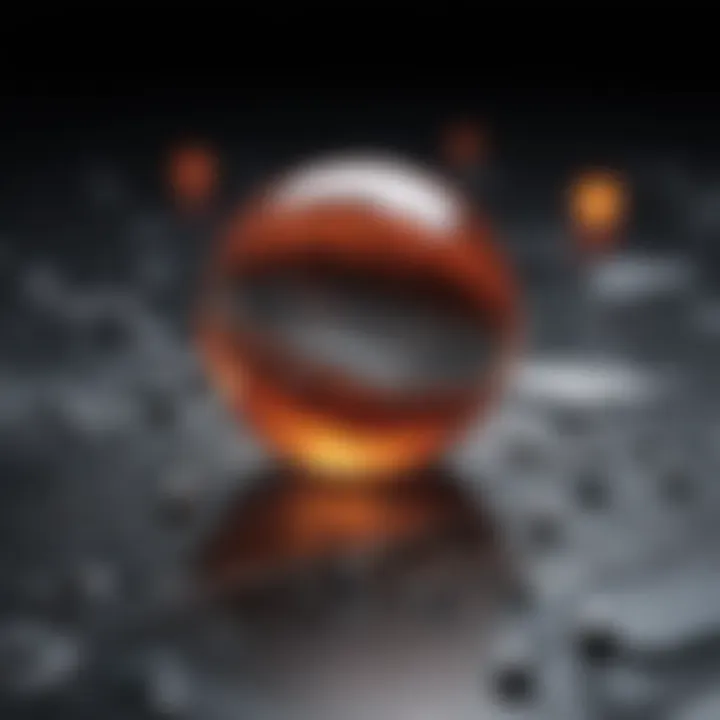
One key characteristic of silicon alloys is their lower melting point compared to pure metals, which allows for easier processing during manufacturing. This significantly benefits industrial applications, particularly within the automotive and aerospace industries where weight reduction and strength are critical.
The unique feature of silicon alloys lies in their versatility. They can be tailored for specific applications, balancing conductivity and strength according to demands. However, one must consider the cost of alloying and the potential complexity of the material properties, which may pose challenges for some applications.
Silicon's Role in Semiconductor Physics
Silicon serves a pivotal role in semiconductor physics due to its intrinsic electrical properties. This section will define how silicon acts as a semiconductor, enabling modern electronics. By controlling its conductivity through doping, engineers manipulate its ability to conduct electricity. This feature is crucial for the creation of transistors and diodes that form the building blocks of circuits in gadgets ranging from simple calculators to complex computers. Understanding silicon's behavior in this context is essential for professionals engaged in semiconductor research and application.
Silicon in Technology
Silicon is fundamental to modern technology, acting as a backbone for various electronic and energy applications. The exploration of silicon in technology allows us to understand its extensive impact on our daily lives. It encompasses advances in electronics, renewable energy systems, and future innovations. Understanding silicon's role in these sectors is crucial for appreciating the progress we have made and the potential for what is next.
Silicon-Based Electronics
Transistors and Integrated Circuits
Transistors are the building blocks of modern electronics. They amplify and switch electronic signals, making possible a range of devices from simple radios to complex computers. Integrated circuits, on the other hand, combine multiple transistors in a single chip to perform various tasks. Their design allows for much smaller and more efficient technology. This compactness has made them a highly beneficial choice in electronics manufacturing. Moreover, the power consumption is significantly reduced compared to earlier models.
A key characteristic of transistors and integrated circuits is their ability to optimize performance while minimizing space. This development has contributed immensely to the advancement of technology. The small form factor allows for portable devices, enabling users to access information and control processes more efficiently. However, challenges such as heat generation and material limitations still exist.
Future Trends in Silicon Technology
Looking ahead, silicon technology continues to evolve rapidly. Innovations like silicon photonics and quantum computing are on the horizon. These advancements allow for faster and more powerful computing capabilities. The integration of silicon photonics in data centers is particularly promising because it enhances data transfer speeds while increasing energy efficiency. Emerging silicon-based technologies have the potential to revolutionize how we compute and communicate.
However, the development of these technologies also poses challenges. There are concerns about fabrication costs, scalability, and the need for new manufacturing processes. Yet, these challenges open more avenues for research and development. Companies are pushing boundaries in silicon applications. The potential reward is significant, leading to smarter electronics and systems.
Silicon in Photovoltaic Cells
Efficiency Improvements
In the field of renewable energy, silicon photovoltaic cells have significantly improved efficiency over the years. The efficiency of these cells measures how effectively they convert sunlight to electricity. Modern advancements have resulted in cells achieving efficiency ratings above 22%. This improvement is crucial in reducing the cost of solar energy and making it a more viable alternative to fossil fuels.
Key characteristics of these efficiency improvements include enhanced material quality and innovative cell design. The use of techniques such as passivation and bifacial technology allows solar cells to capture more sunlight. As a result, solar energy becomes more accessible and widespread, providing a cleaner energy source.
However, there remain challenges in scalability and market adoption. Storage solutions must be developed to complement the increased generation of solar energy. Nevertheless, the gradual shift toward renewable energy systems emphasizes the importance of silicon efficiency improvements.
Emerging Technologies
Emerging technologies in silicon for photovoltaic applications are gaining attention. These include concepts like perovskite solar cells, which promise to offer higher efficiency and lower production costs. Researchers are investigating how to incorporate these new materials with traditional silicon cells to maximize energy output.
A key characteristic of these emerging technologies is their potential to disrupt the existing market. New combinations could lead to breakthroughs in solar efficiency and cost, making solar power more competitive. Testing the reliability and lifespan of these new technologies is still essential as they come into play. However, their potential benefits outweigh the investment risks, making them a popular focus for new research. So, investing in these technologies could lead to a significant impact on energy production in the coming decades.
The advancements in silicon technology and applications open up a new realm of possibilities for future research and innovation, ensuring silicon remains at the forefront of technological development.
Biological Significance of Silicon
Silicon plays a crucial role in biological systems, a facet often overlooked in contrast to its technological applications. The significance of silicon in biological contexts extends from its role in plant health to its implications for human health. Understanding these dimensions provides deeper insights into this element's versatility.
Silicon in Biological Systems
Silicon in Plants
Silicon acts as a beneficial element in plant growth and development. It contributes to the structural integrity of plants, helping to build cell walls. This element enhances mechanical resistance against various stresses, such as pests and diseases. Consequently, plants with access to silicon tend to show increased resilience.
Key characteristics of silicon in plants include its ability to improve nutrient uptake and enhance photosynthesis efficiency. These traits make silicon a popular choice for agricultural practices focused on boosting crop yield and quality. Silicon's unique feature lies in its role in promoting silica deposition, which can help fortify the plant's structure.
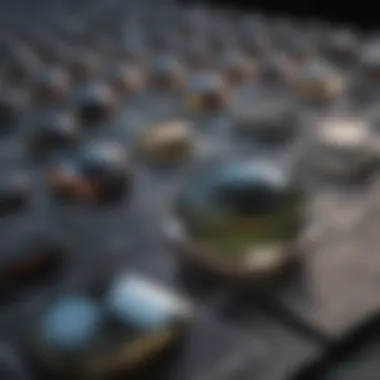
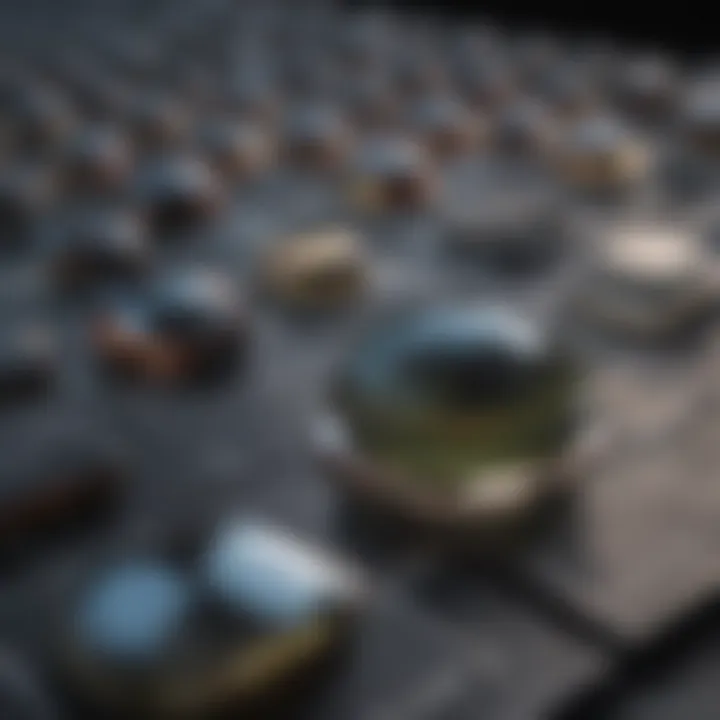
Advantages of silicon application in agriculture include improved plant health and increased resistance to biotic and abiotic stresses. However, there are also challenges. The availability of silicon in soil is dependent on various factors, including soil type and pH. This variability can affect its effectiveness.
Role in Human Health
Silicon's influence in human health is emerging as a vital area of research. It is believed to play a role in bone formation and maintenance, thus contributing to overall skeletal health. Additionally, silicon may aid in the synthesis of collagen, important for skin and connective tissue.
A key characteristic of silicon in human health is its potential to support cardiovascular function by influencing arterial health. This property highlights silicon as a significant nutrient in maintaining bodily functions. Its unique feature includes promoting the mineralization of connective tissues, which offers health benefits but also raises questions about silicon's bioavailability in humans.
Despite promising advantages, the understanding of silicon's role in human health is still developing. Research is ongoing to determine optimal levels and forms of silicon intake for maximum benefits.
Silicon-Based Biomaterials
Applications in Medicine
Silicon-based biomaterials are gaining traction in medical applications, especially in tissue engineering and regenerative medicine. These materials provide a framework for cell attachment and growth, which is essential for developing functional tissue constructs.
Silicon's key characteristic in medicine is its biocompatibility, making it suitable for use in implants and medical devices. This characteristic is beneficial because it minimizes the risk of adverse reactions in the body. Unique features, like the ability to deliver drugs in a controlled manner, highlight silicon's versatility in medical applications. However, there are challenges, notably regarding long-term stability and how the body interacts with silicon-based materials over time.
Future Research Directions
The future of silicon in medical research is promising, with ongoing investigations into new applications and biodegradability. Researchers are exploring how silicon can improve implant performance and integration within biological systems.
A notable characteristic of future research directions in silicon is the focus on developing more efficient biomaterials. Such advancements could open up new possibilities in personalized medicine. The unique features being explored include enhancing functionalization methods to improve compatibility and performance of silicon-based materials. However, potential downsides could arise from unforeseen biological responses, highlighting the need for rigorous testing.
"Silicon-based innovations in medicine stand at the intersection of technology and biological application, presenting opportunities for groundbreaking advancements."
Environmental Impact of Silicon Mining and Production
The environmental impact of silicon mining and production is a critical topic in understanding the broader implications of utilizing this essential element in various applications. Silicon is not only vital for technology and biology but also poses significant challenges to the environment during its extraction and processing phases. Addressing these issues is important for creating sustainable practices that can mitigate negative effects while optimizing silicon's benefits in multiple fields.
Silicon Extraction Processes
Silicon is primarily extracted from quartz, also known as silicon dioxide, through processes that can be quite intensive on energy and resources. The most common method is the carbothermic reduction, where quartz is heated with carbon in an electric arc furnace. This process generates silicon metal, but it also emits considerable amounts of carbon dioxide and requires large amounts of energy, often sourced from non-renewable supplies.
- Environmental Concerns: The extraction process includes the potential for landscape disruption and habitat destruction. The mining of quartz can lead to soil erosion, decreased biodiversity, and contamination of local water sources.
- Energy Consumption: The production of silicon metal is energy-intensive. The reliance on fossil fuels for electricity can exacerbate the carbon footprint associated with silicon production.
- Byproducts: The process often results in waste materials that must be managed properly to prevent environmental hazards.
Sustainability Practices in Silicon Production
Recognizing the environmental ramifications, the silicon industry is exploring various sustainability practices. The aim is to reduce harmful impacts while meeting the growing demand for silicon. Some notable practices include:
- Energy Efficiency: Implementing more efficient technologies in silicon production can significantly reduce energy use. Innovations in furnace designs and heat recovery systems are promising steps.
- Renewable Energy Sources: Using renewable energy, such as solar or wind power, can lessen the carbon impact of silicon production. Many companies are investing in these solutions to power their manufacturing processes.
- Recycling Silicon: Efforts to recycle silicon from end-of-life products, like solar panels and electronic devices, are gaining traction. This practice not only decreases the need for raw materials but also minimizes waste.
- Responsible Mining Practices: Companies are being urged to adopt practices that limit landscape disruption and prioritize ecosystem preservation. This includes adhering to environmental regulations and engaging with local communities.
"To ensure the continued availability of silicon and its applications, it is vital to implement sustainable practices that address environmental impacts while optimizing efficiency."
Through these sustainability measures, the silicon industry can aim to balance the environmental considerations with the demands of modern technology and research. Engaging in these practices not just preserves natural resources but also enhances the viability of silicon in future advancements.
Epilogue
The examination of silicon forms encapsulates a broad scope of applications and innovations that extend well beyond basic electronic components. Silicon's versatility makes it a pivotal element in modern technology, biology, and materials science. As we navigate through these intricate domains, it becomes evident that silicon is not merely a passive participant but rather a driving force behind many advancements.
Future Prospects for Silicon Research
Looking ahead, the possibilities for silicon research seem expansive. New methods of silicon synthesis and purification are being developed. These advancements pave the way for high-purity silicon that meets stringent requirements for solar cells and semiconductor devices. The exploration of silicon's properties in nanotechnology is also gaining momentum. Researchers are studying silicon nanoparticles that offer promising applications in targeted drug delivery and imaging techniques in medicine.
Silicon is fundamental in various fields, including renewable energy, biomedical engineering, and electronics, making its research crucial for future innovations.
Additionally, the integration of silicon with other materials, such as graphene and silicon carbide, is an area ripe for exploration. These combinations could lead to novel devices that outperform traditional silicon-based technologies.
The Role of Silicon in the Next Generation of Technologies
Silicon's influence on the next generation of technologies cannot be overstated. As we advance into an era marked by artificial intelligence and the Internet of Things, silicon remains at the core of these developments. Efforts to enhance silicon's efficiency will take center stage, especially in developing more powerful processors and energy-efficient systems.
Moreover, silicon's role in renewable energy technologies is critical. The push for better-performing photovoltaic cells requires innovations centered on silicon alloys and composite materials. Emerging trends also include smart technology integration into everyday devices, where silicon serves as a key component, enabling seamless connectivity.