Exploring RNA Stabilization Solutions and Their Impact
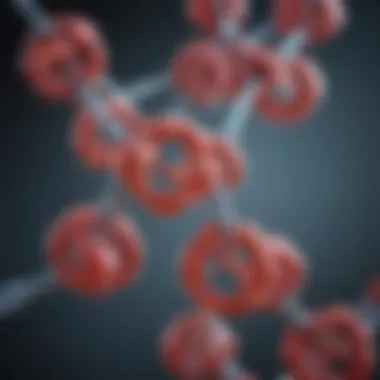
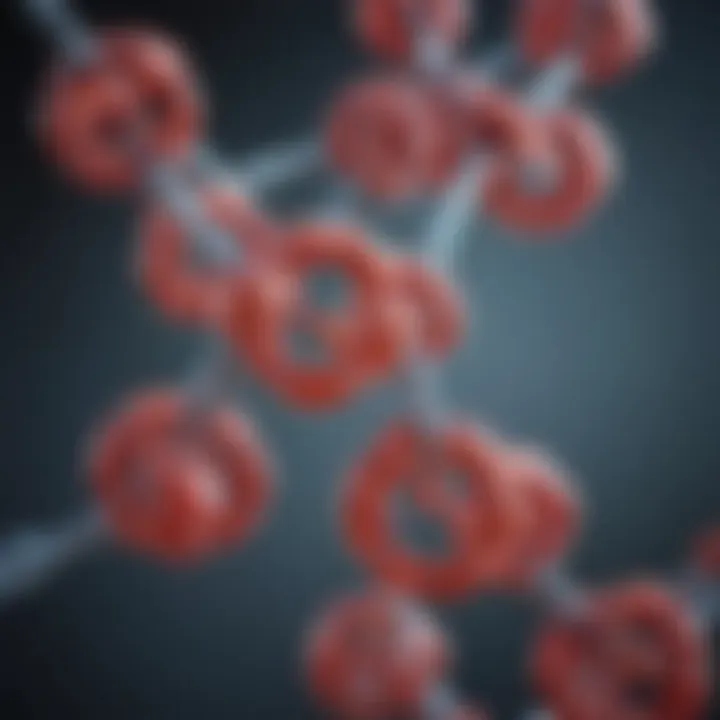
Intro
The significance of RNA in modern biology can’t be overstated. It serves as a critical player in gene expression and regulation, making it pivotal for the understanding of countless biological processes. However, just like a man trying to hold sand, RNA is incredibly fragile and susceptible to degradation. That being the case, the demand for effective RNA stabilization solutions has surged over the years. We’re diving into the depths of the mechanisms, applications, and future implications of these solutions, ensuring clarity in an often convoluted topic.
Methodology
Overview of research methods used
To provide a thorough analysis of RNA stabilization solutions, a multifaceted research approach was adopted. This involved:
- Literature review: A deep dive into existing scholarly articles and reviews discussing the various stabilization techniques and their mechanisms.
- Case studies: Examining specific instances where stabilization solutions have been applied effectively in both laboratory and clinical settings.
- Expert interviews: Engaging with scientists and researchers actively working in the field to gather firsthand insights and real-world applications.
Data collection techniques
Data collection was conducted using an assortment of strategies to ensure a comprehensive understanding of the topic. These included:
- Surveys: Targeted surveys were distributed to researchers, capturing their experiences and preferences regarding RNA stabilization.
- Online databases: Utilizing databases like PubMed to collect quantitative and qualitative data on recent advancements in RNA stabilization.
- Field observations: Direct observation during laboratory studies provided practical insights that mere theoretical research could not achieve.
Mechanisms of RNA Stabilization
RNA stabilization solutions operate through several mechanisms, each uniquely tailored to protect RNA from enzymatic degradation. Common mechanisms include:
- Chemical modifications: Alterations like methylation and phosphorothioation can make RNA less susceptible to nucleases.
- Use of stabilizing agents: Compounds like polyethylene glycol or certain salts may foster a protective environment for RNA.
- Encapsulation technologies: Innovative approaches include the use of liposomes or nanoparticles to encapsulate RNA, shielding it from degradation.
Applications in Research
The applications of RNA stabilization are diverse, transcending basic research and spilling into various domains such as:
- Clinical diagnostics: Reliable RNA preservation is essential for accurate testing.
- Therapeutics development: RNA-based medications require stability to ensure efficacy.
- Molecular biology: Experiments often rely on intact RNA samples to derive meaningful conclusions.
One case study highlighted a preliminary trial using an innovative encapsulation method. Researchers reported a % improvement in RNA integrity over standard methods, underscoring the potential for future exploration in this realm.
Future Directions
With the ever-evolving landscape of molecular biology, several trends and areas of further investigation are burgeoning:
- Advancements in technology: Developing new materials that enhance RNA stability, such as biocompatible polymers or next-gen nanoparticles.
- Personalized medicine: Exploring stabilization solutions tailored to specific patient needs, which could significantly enhance therapeutic efficacy.
- Environmentally friendly solutions: Investigating the use of biopolymers or natural compounds for RNA stabilization that align with green chemistry principles.
"Emerging RNA technologies hold great promise, yet their implementation hinges on achieving optimal stability for real-world applications."
Ending
Prelude to RNA Stabilization
In the vast field of molecular biology, the integrity of ribonucleic acid, or RNA, takes center stage. RNA plays numerous roles in the cell, functioning primarily as a messenger between DNA and proteins. However, RNA is inherently unstable; it can degrade quickly due to environmental factors or enzymatic actions. This vulnerability presents challenges in both research and clinical settings. Therefore, understanding RNA stabilization solutions is imperative for ensuring the reliability of RNA studies and applications.
The Importance of RNA Stability
Why is RNA stability so crucial? In simplest terms, the reliability of any experiment relying on RNA rests on how well that RNA can resist degradation. When RNA degrades, it compromises the validity of the data, leading researchers down a rabbit hole of complications. To put it bluntly, if you can't trust your RNA, you can't trust your results.
Key benefits of RNA stabilization include:
- Enhanced reproducibility of experimental results. This is vital for peer review and building upon existing research.
- Increased accuracy in diagnostics related to RNA, such as in detecting viral infections or genetic disorders.
- Improved therapeutic outcomes in RNA-based treatments, ensuring that the RNA components remain functional.
A failure to address RNA stability can mean the difference between a groundbreaking discovery and a failed experiment. If researchers overlook this aspect, they might as well be navigating a dark tunnel with no light in sight.
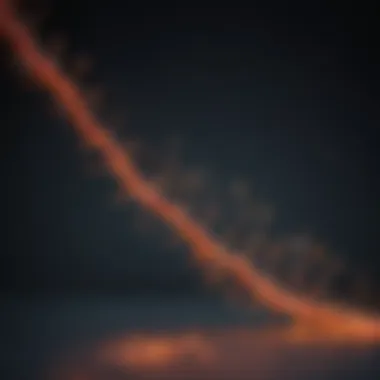
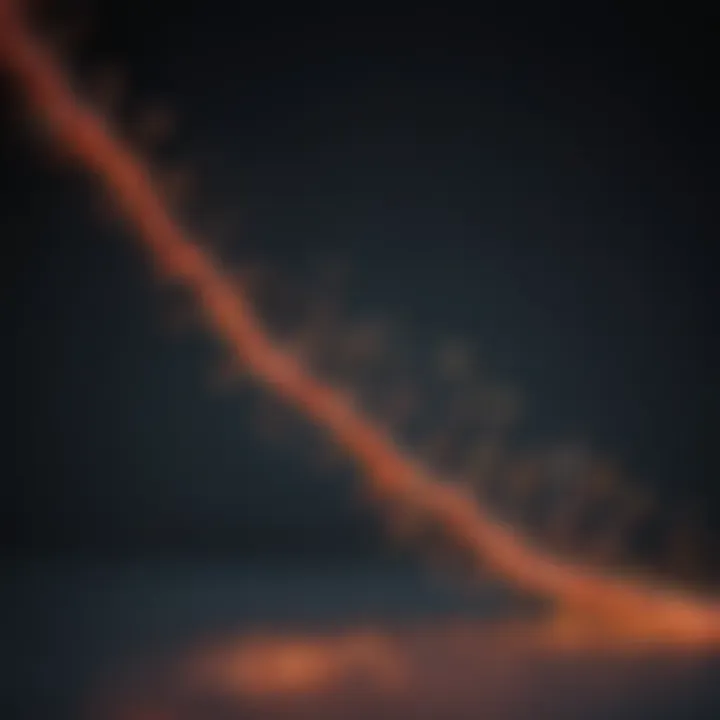
Overview of RNA Types and Functions
Understanding the various types of RNA is crucial in the context of stabilization solutions. Each type serves distinct purposes within the cell:
- Messenger RNA (mRNA): Acts as the intermediary, conveying genetic information from DNA to the ribosome, where proteins are synthesized. It’s essential for the proper expression of genes.
- Ribosomal RNA (rRNA): A building block of the ribosome, rRNA is vital for protein synthesis. Its stability is significant for maintaining the overall structure and function of ribosomes.
- Transfer RNA (tRNA): Functions as the adaptor molecule that flips amino acids into the ribosome so they can be assembled into proteins. Stability ensures that tRNA effectively delivers its cargo.
These types highlight not only the diversity of RNA but also its central role in cellular functions. RNA is like a finely tuned machine; if one component falters, the entire operation can stall. Getting a grip on their specific requirements for stabilization is therefore pivotal in any RNA-related endeavor.
Chemical Composition of RNA Stabilization Solutions
Understanding the chemical composition of RNA stabilization solutions is paramount to maximizing their efficacy. The delicate nature of RNA presents unique challenges that these solutions are designed to overcome. By comprehending the constituents of these formulations, researchers can better assess their applicability in various scenarios. Unpacking the complexities of each component reveals how they work synergistically to preserve RNA integrity.
In this intricate biological landscape, it's vital to consider factors such as stability, environmental conditions, and specific application requirements. Effective RNA stabilization solutions not only prolong the lifespan of RNA but also guard against degradation caused by enzymes and external factors. As researchers delve into the inner workings of these solutions, they uncover insights that can significantly influence experimental outcomes.
Key Components and Their Roles
The formulation of RNA stabilization solutions often includes several key components, each playing a crucial role in maintaining RNA integrity:
- Buffering Agents: These are instrumental in maintaining a stable pH level, which is critical for RNA. Without proper pH control, RNA can degrade rapidly. For instance, Tris and phosphate buffers are commonly utilized due to their effectiveness in maintaining an optimal environment.
- Chelating Agents: These substances, such as EDTA, bind to divalent metal ions that could potentially catalyze RNA degradation through oxidative processes. This is a protective measure against any unwanted enzymatic activity that could compromise RNA samples.
- Stabilizers: Compounds like glycerol or polyethylene glycol are often added to enhance the overall stability of RNA. They can assist in preventing freezing damage or degradation during storage, thus preserving the material for future use.
- Antioxidants: Certain formulations incorporate antioxidants to counteract oxidative stress, which can severely impact RNA's stability. This is particularly important for samples exposed to air or light.
Each component contributes uniquely to the solution’s overall efficacy. Understanding their specific roles helps researchers choose the most suitable formulation for their specific needs.
Comparative Analysis of Different Formulations
Not all RNA stabilization solutions are created equal. A comparative analysis sheds light on the variations among these formulations and their suitability for different applications. For instance, some solutions are specifically tailored for short-term storage, while others may be designed for extended use under varying conditions.
When examining the pros and cons of various formulations, researchers might consider:
- Performance: Some solutions may outperform others in preserving RNA integrity under specific conditions. For example, a solution that is exceptionally effective in high-temperature environments might not be as effective in maintaining stability at lower temps.
- Compatibility: Certain additives might influence downstream applications like reverse transcription or PCR, making compatibility a crucial consideration in choosing a formulation.
- Cost-effectiveness: Budgetary constraints are often an unspoken factor. Evaluating the costs associated with different options can guide researchers toward making informed decisions without sacrificing quality.
An interesting aspect to examine is the evolving nature of these solutions. Newer formulations may incorporate cutting-edge materials or techniques, leading to improved stabilization performance.
"The choice of RNA stabilization solution can make or break an experiment, often determining the reliability of results."
Mechanisms of RNA Stabilization
Understanding the mechanisms through which RNA stabilization occurs is crucial for researchers and practitioners in the field of molecular biology. With RNA's inherent instability, due to its single-stranded structure and susceptibility to enzymatic degradation, it's vital to explore how stabilization solutions can mitigate these challenges. The significance of these mechanisms extends beyond mere preservation; they enhance experimental reliability, improve the quality of RNA for therapeutic applications, and increase reproducibility in research settings.
Chemical Interactions That Enhance Stability
At the heart of RNA stabilization lies a variety of chemical interactions that contribute significantly to its integrity. These interactions include hydrogen bonding, ionic interactions, and hydrophobic interactions. Understanding how these forces interplay can lead to smarter formulations designed for specific applications.
- Hydrogen Bonding: This occurs between the nucleobases of RNA and surrounding solvent molecules or stabilizing agents. Such bonding can prevent the strands from unwinding, thereby maintaining RNA’s secondary structures.
- Ionic Interactions: The presence of ionic compounds can shield negative charges on the RNA backbone. This is essential in preventing degradation by ribonucleases, enzymes known to cleave RNA.
- Hydrophobic Interactions: The chemical environment—particularly the presence of hydrophobic agents—can promote stability by limiting water exposure and, in turn, reducing the likelihood of hydrolysis reactions that could compromise RNA integrity.
To sum up, grasping these interactions informs the design of more effective RNA stabilization solutions, ultimately leading to improved outcomes in various RNA-based applications.
Role of Environment in RNA Integrity
The environmental conditions surrounding RNA play a pivotal role in its stability. Factors such as temperature, pH, and ionic strength can dramatically affect RNA's integrity. Each of these factors must be carefully controlled during experiments to ensure accurate results.
- Temperature: RNA is notorious for being sensitive to thermal fluctuations. High temperatures can lead to denaturation, while low temperatures may slow the activity of protecting agents. Therefore, maintaining an optimal temperature is crucial for RNA preservation.
- pH Levels: The acidity or basicity of the environment can affect the ionization of nucleotides and their interactions with stabilizing agents. Ideally, pH should be kept within a range that favors RNA stability, often around neutrality.
- Ionic Strength: The concentration of salts in the solution can impact the electrostatic interactions of RNA. A balanced ionic strength can enhance or inhibit enzymatic activity, thus influencing RNA preservation.
In light of these environmental considerations, strategies for RNA stabilization must be multifaceted. Not only should the composition of the stabilization solution be optimized, but also the conditions under which RNA is handled and stored. This comprehensive approach can extend the lifespan and efficacy of RNA for various downstream applications.
"The very essence of RNA stability hinges not merely on chemical composition but intricately intertwines with the environmental context. Ignoring one aspect might doom the entire project to failure."
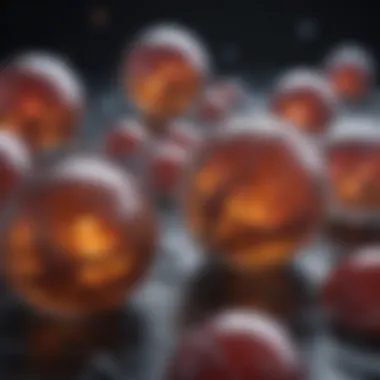
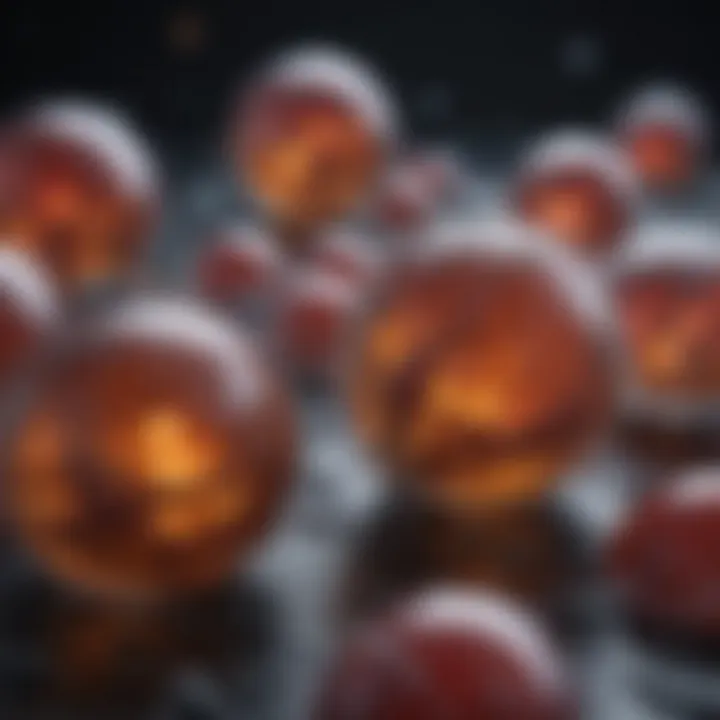
By delving into both the chemical and environmental mechanisms of RNA stabilization, we can better appreciate the nuanced factors contributing to successful RNA preservation. This knowledge equips researchers with the tools they need to craft effective stabilization strategies tailored to their specific needs and applications.
Applications of RNA Stabilization Solutions
The application of RNA stabilization solutions holds a significant position in modern biological sciences. These solutions are crafted to protect RNA molecules from degradation that can derail research outcomes or clinical diagnostics. In an era where precision and reliability are paramount, understanding how these solutions function in various contexts becomes crucial. Their role extends beyond mere preservation; they're instrumental in influencing experimental designs, facilitating advances in research, and bridging gaps between laboratory findings and clinical applications.
Research Applications in Molecular Biology
In molecular biology, the quest for understanding RNA dynamics has led to extensive incorporation of stabilization solutions. When conducting experiments involving RNA, researchers often face the peril of degradation, which can manifest in poor yield and unreliable data. Utilizing stabilization solutions can enhance the quality of RNA samples significantly.
- In Situ Hybridization: These solutions aid in maintaining RNA integrity during in situ hybridization experiments, allowing accurate localization studies within tissues.
- Transcriptomics: In the field of transcriptomics, the need for reliable RNA extraction methods cannot be overstated. Solutions like RNAlater can preserve RNA for later analysis, thus preventing sample loss over time.
- Gene Expression Analysis: Researchers examining gene expression can benefit from these stabilization techniques, ensuring that fluctuations observed in results are due to biological variance rather than sample degradation.
The incorporation of RNA stabilization solutions allows for a more nuanced understanding of various RNA species, contributing to discoveries that may otherwise remain hidden.
Clinical Relevance in Diagnostics and Therapeutics
In the clinical realm, the implications of RNA stabilization are equally critical. The accuracy of diagnostics heavily relies on the integrity of RNA extracted from biological samples. Whether it’s assessing disease markers or guiding therapeutic decisions, RNA stabilization can enhance the reliability of diagnostic assays.
- Cancer Diagnostics: In oncology, stabilized RNA is essential for identifying genetic mutations through assays like liquid biopsies. The data gained from RNA analyses can inform targeted therapies and improve patient outcomes.
- Infectious Disease Detection: Timely diagnosis of infectious diseases requires pristine RNA samples. Solutions designed to stabilize RNA can ensure robust performance of polymerase chain reaction (PCR) tests, crucial in managing outbreaks.
The use of RNA stabilization solutions positions healthcare professionals to make better-informed decisions that can significantly affect patient management.
Impact on Biotechnological Innovations
RNA stabilization solutions not only influence the current landscape of molecular biology and clinical diagnostics but also pave the way for biotechnological innovations. As researchers explore gene therapies, regenerative medicine, and synthetic biology, the demand for stable RNA preparation becomes more evident.
- Gene Therapy: In gene therapy, the persistence and stability of RNA constructs can make or break the success of therapeutic applications, often aimed at correcting genetic disorders.
- Synthetic Biology: Developers in synthetic biology rely on RNA for creating predictable cellular behaviors. RNA-stabilization methods allow for more efficient programming of cells toward desirable traits.
"The proper handling and stabilization of RNA can markedly improve the success rates in the burgeoning fields of biotechnology and therapeutic development."
Overall, the beneficial applications of RNA stabilization solutions extend across various domains, from research to clinical practices, and are pivotal for fostering biotechnological advancements that continue to emerge.
Critique of Existing RNA Stabilization Solutions
Examining the effectiveness of RNA stabilization solutions is crucial as it reveals not only their utility but also their shortcomings. The applications of these solutions are vast, ranging from academic research to clinical diagnostics, yet a closer look at the existing formulations can lead to insightful discoveries. This section focuses on analyzing the limitations of current products while emphasizing the necessity for advancements in RNA preservation techniques.
Limitations of Current Formulations
Current RNA stabilization solutions, while capable of extending the lifespan of RNA samples, often have inherent limitations that could compromise their overall effectiveness. Here are some primary factors worth considering:
- Stability Under Various Conditions: Many formulations perform variably under different environmental conditions. For instance, what might excel in a controlled lab setting may not fare well in real-world applications where temperature, humidity, and light exposure can fluctuate widely.
- Compatibility Issues: A significant drawback is the compatibility of these solutions with downstream applications. Certain stabilizers may interfere with enzymatic reactions or affect the efficiency of subsequent analytical methods like qPCR or RNA sequencing.
- Cost Efficiency: Price can also be a barrier. Some advanced formulations are prohibitively expensive, limiting their accessibility to smaller labs or research institutions. This can foster an inequitable distribution of resources in research environments, where the wealthy laboratories get access to better stability solutions while others do not.
- Chemical Safety: Another important aspect to consider is the safety of the reagents involved. Some stabilization solutions can involve hazardous chemicals that raise concerns over safety and environmental impact.
The limitations highlight the need for comprehensive assessments in the chemistry of these solutions. Addressing such concerns can help in developing more robust formulations.
Beyond Conventional Solutions: A Need for Enhancement
The rapid advancements in biotechnological research underscore an urgent need for improvements in RNA stabilization practices. As the demand for high-quality RNA samples rises, exploring beyond conventional solutions becomes imperative. Here are some areas where further innovation is vital:
- Nanotechnology Applications: The integration of nanotechnology can lead to enhanced stabilization techniques. Nanoparticles could effectively protect RNA from degradation, thus offering impressive preservation capabilities in varied conditions.
- Biocompatible Polymers: Using specially designed biocompatible polymers may mitigate interaction issues seen with many traditional stabilizers. Such advancements could offer tailored solutions that resonate with specific RNA applications.
- Customized Formulations: The future may lie in the design of customized solutions. Tailoring stabilization solutions to the specific needs of particular RNA types, like mRNA or miRNA, could drastically improve both stability and function.
- Smart Stabilization Techniques: Leveraging smart materials that respond to environmental stimuli may open new pathways for RNA preservation. If RNA could be protected reactively, responding to changes in its environment, it could significantly enhance stability and reliability.
In summary, critically analyzing existing RNA stabilization solutions helps to pinpoint gaps that can be addressed with scientific ingenuity. Broadening our perspective beyond conventional methods will empower scientists to evolve RNA preservation strategies and harness their full potential in research and therapeutic settings.
"The critique of existing solutions is not merely an examination of imperfection but an invitation to innovate and elevate the practices that bind our scientific pursuits."
The assessment and progressive enhancement of RNA stabilization methods not only holds significance for research applications but could ultimately impact personalized medicine and beyond.
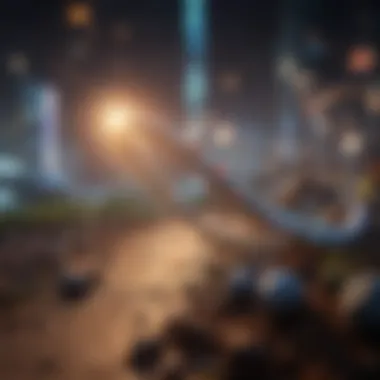
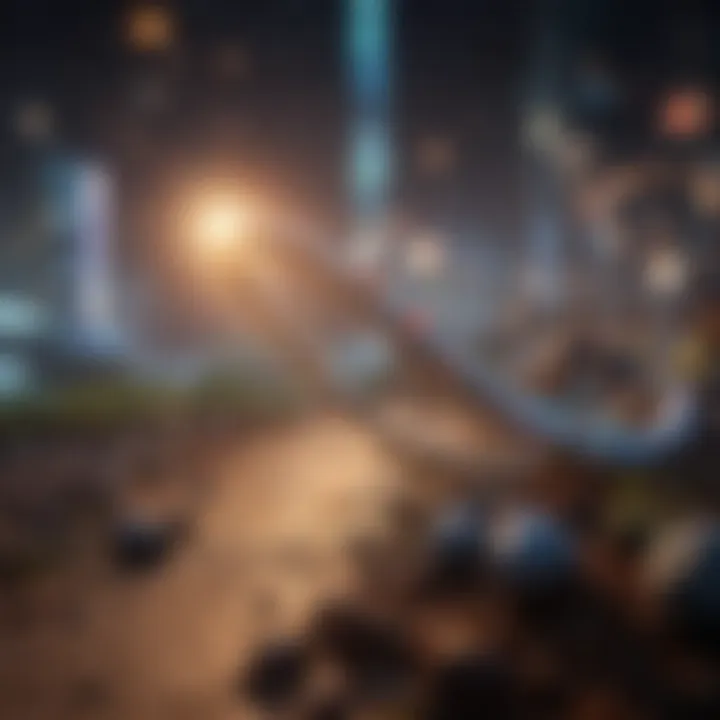
Future Directions in RNA Stabilization
The future of RNA stabilization is a burgeoning area of interest within molecular biology and biotechnology. As researchers increasingly recognize the integral role of RNA stability in both basic science and applied research, innovative solutions and cutting-edge technologies are emerging. These advancements are not merely academic; they have significant implications for research methodologies, therapeutic regimes, and diagnostic accuracy.
Emerging Technologies in RNA Preservation
In recent years, a variety of technologies have surfaced aimed at enhancing RNA preservation. One promising avenue is the use of nanomaterials to stabilize RNA. For instance, carbon nanotubes and gold nanoparticles have demonstrated potential for preventing RNA degradation by acting as scaffolds that protect against ribonucleases. These materials provide a protective matrix, ensuring that RNA molecules remain intact for longer periods.
Another notable approach involves the incorporation of cryoprotectants in RNA storage solutions. Cyroprotectants, such as dimethyl sulfoxide (DMSO), have been shown to mitigate cold-induced damage when samples are stored at low temperatures. This instances an increased longevity, allowing for better reproducibility of results across experiments.
Moreover, researchers are exploring synthetic biomaterials designed to mimic the natural cellular environment. These bio-inspired matrices can interact with RNA through various biochemical forces, helping to maintain its structure and function. This not only improves stability but also enhances the likelihood of successful downstream applications.
In addition to these advancements, the integration of artificial intelligence (AI) in the development of preservation techniques is noteworthy. AI algorithms are capable of predicting the optimal conditions for RNA stabilization, taking into account numerous variables such as temperature, pH, and ionic strength. This intersection of technology and biology could revolutionize how researchers approach RNA handling and storage.
Potential for Personalized Medicine
The implications of RNA stabilization extend into personalized medicine, which tailors treatment based on individual genetic profiles. The capacity to effectively stabilize RNA allows for more reliable diagnostics and therapies that rely on RNA biomarkers. By ensuring that RNA samples remain intact and functional, healthcare providers can make more accurate assessments and create personalized treatment plans.
Furthermore, advancements in RNA stabilization techniques can enhance the efficacy of RNA-based therapeutics, such as mRNA vaccines and gene therapies. In these contexts, maintaining RNA integrity is crucial for ensuring optimal delivery and expression of therapeutic agents within the target cells. Innovations in stabilization methods can potentially lead to more effective treatments with fewer side effects.
The marriage of RNA stabilization with personalized medicine holds promise for more effective disease management strategies. It encourages a shift away from one-size-fits-all approaches, offering a glimpse into tailored treatments that harness the full potential of RNA technology.
Finale
The topic of RNA stabilization is paramount in both research and therapeutic landscapes, rendering this exploration not just useful but essential. As this article elucidates, the mechanisms behind RNA stabilization solutions, along with their diverse applications, underscore the intricate balance between science and technology in the field of molecular biology. The necessity for robust RNA preservation cannot be overstated, especially given the fragile nature of RNA and its susceptibility to degradation under various conditions.
Summary of Key Insights
Throughout the discourse in this article, several key insights emerge, highlighting the significance of RNA stabilization:
- Central Role in Research: RNA stabilization solutions play a critical part in ensuring sample integrity, which ultimately enhances reproducibility in experimental outcomes. For researchers, this translates to more reliable data, paving the way for innovative discoveries that could impact various domains, from genetics to biotechnology.
- Diverse Applications: From diagnostic tests to therapeutic remedies, the utility of RNA stabilization reaches far and wide. It aids in developing RNA-based therapies, improving the stability and effectiveness of mRNA vaccines, and is pivotal in fetal and cancer diagnostics.
- Emerging Technologies: Innovations in preservation technologies are on the rise, including techniques that modify the environmental conditions surrounding RNA molecules, showing promise for enhanced stability.
This insight-filled journey through the mechanisms and applications of RNA stabilization elucidates a layered understanding that extends far beyond chemical composition.
Implications for Future Research
The future directions in RNA stabilization are ripe with possibilities, setting the stage for exciting breakthroughs in the scientific community:
- Continued Optimization: Future research might focus on fine-tuning existing stabilization solutions, making them more effective or versatile across different RNA types and conditions.
- Interdisciplinary Approaches: The integration of RNA stabilization techniques with advancements in fields such as nanotechnology or synthetic biology could yield novel solutions that expand the capabilities of RNA-related applications.
- Personalized Medicine: As precision medicine becomes more mainstream, the implications of RNA stabilization in tailoring therapies to individual genetic expressions cannot be overlooked. Future studies could explore the dynamic relationship between RNA stability and patient-specific responses to treatments.
Importance of Citing Sources
First and foremost, references serve as the backbone of credibility. When discussing complex biological mechanisms or novel applications, citing previous works allows researchers to validate claims and assertions based on established findings. For instance, mentioning primary research articles or review papers demonstrates that the conclusions drawn are grounded in rigorous scientific inquiry. This is especially vital in a fast-evolving field like RNA research, where discoveries emerge at a rapid pace.
Enabling Further Exploration
Moreover, references offer readers the chance to dive deeper into a subject. A well-referenced article encourages the audience to explore sources that they might not have encountered otherwise. This does not just enhance their understanding but also fosters a culture of continuous learning and inquiry. A well-placed citation can lead a curious mind down a rabbit hole, engaging them with studies that could range from foundational work to cutting-edge research.
Practical Applications for Researchers
In practical terms, having a robust reference section can guide researchers when designing their own experiments or studies. By identifying methods and protocols discussed in cited works, scientists can adapt and refine techniques that have been proven successful or insightfully critiqued by peers. This makes research more streamlined and reduces the likelihood of redundancy in efforts, ultimately contributing to more innovative findings.
Considerations for Proper Referencing
However, it’s critical to approach references thoughtfully. Not every cited work carries the same weight; thus, distinguishing between high-impact research articles and lesser-known studies is crucial. Misleading or improperly used references can confuse or misinform the reader, undermining the entire narrative. For instance, relying heavily on outdated studies in a field that thrives on recent developments might hinder the discourse rather than advance it.
"Well-sourced references don't just increase credibility; they can also inspire future research avenues that could lead to breakthrough discoveries."
The End
In summary, the integration of references in discussions surrounding RNA stabilization solutions significantly enriches the content. It not only adds a layer of trustworthiness but also encourages an environment of exploration and ingenuity. Through careful selection of sources, writers can contribute to the ongoing narrative in scientific discourse, bridging gaps in understanding and paving the way for new research horizons.