The Comprehensive Role of Latices in Science
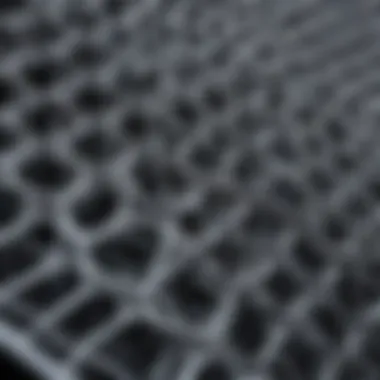
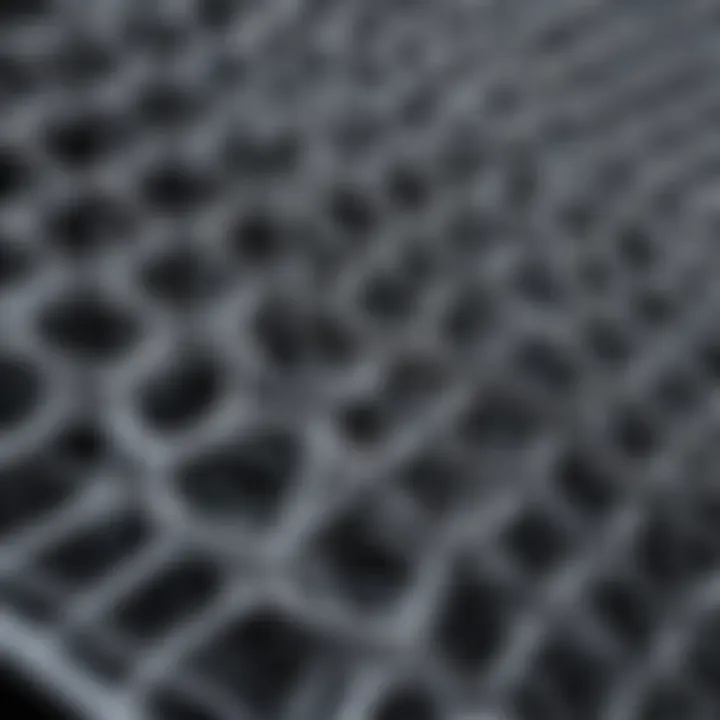
Intro
Latices are a significant area of interest across multiple scientific disciplines. Their potential applications are extensive, ranging from material science to biomedical research. Understanding latices involves studying their structure, composition, and dynamics. As such, this article aims to offer an in-depth exploration of latices, providing clarity on their definitions, various types, and unique properties.
Latices represent colloidal systems where tiny particles, usually between 1 nanometer and 1 micron, are dispersed in a liquid medium. This unique composition allows them to exhibit distinct behaviors in response to environmental changes. The focus on latices in scientific research is not merely academic; it has practical implications that can influence technological and medical advancements.
In this exploration, we will cover recent advancements as well as future directions in the study of latices. Each section will build on the last, creating a cohesive understanding of this complex subject that caters to students, researchers, educators, and professionals in various fields.
Methodology
Exploring the scientific landscape of latices requires a methodical approach. The methodology section will detail various research methods used in the examination of latices.
Overview of Research Methods Used
Research methods in latex studies can broadly be categorized into experimental and computational frameworks. Experimental approaches often involve:
- Synthesis techniques: These are crucial for creating different types of latices, which may involve chemical processes or mechanical dispersion methods.
- Characterization methods: Techniques such as transmission electron microscopy or dynamic light scattering are employed to examine the size and distribution of particles within the latices.
- Dynamic studies: Observing how latices respond under varying conditions adds essential data for understanding their properties.
Computational methods, such as molecular dynamics simulations, also play a critical role. These techniques help researchers predict the behavior of latices under different conditions, forming a bridge between theoretical and practical findings.
Data Collection Techniques
Data on latices can be gathered through a variety of approaches:
- Experimental data: Direct measurement via laboratory equipment helps to gather quantitative results about physical properties.
- Surveys of peer-reviewed literature: Analyzing existing studies provides context and background, helping researchers build on previous findings.
- Collaborative datasets: In interdisciplinary research, sharing data across fields enhances the understanding of how latices might apply in various contexts.
For proper validation, data must be rigorously analyzed and peer-reviewed, ensuring reliability and trustworthiness in the findings.
Future Directions
The field of latex science is ever-evolving, presenting significant potential for future research. Here are two key areas warranting attention:
Upcoming Trends in Research
There is a growing trend toward using latices in drug delivery systems. Researchers are focusing on engineering latices that can effectively transport therapeutic agents to targeted areas in the body. Also, sustainable materials created from latices are becoming increasingly important as industries push towards eco-friendly innovations.
Areas Requiring Further Investigation
Despite the advancements, many questions remain. Areas for further research include:
- Nano-latices: Investigating behaviors at the nanoscale can reveal new applications in electronics and medicine.
- Stability mechanisms: Understanding the factors that affect the stability of latices will lead to more effective applications in various industries.
Understanding Latices: A Scientific Overview
Latices are an essential component in various scientific research areas, offering a fascinating combination of properties that can be explored for multiple applications. As a foundational subject in modern science, understanding latices provides clarity on their roles in disciplines ranging from biology to nanotechnology. This section aims to provide a coherent overview of latices, ensuring that both students and professionals grasp their significance in scientific innovation.
Definition of Latices
Latices refer to a stable dispersion of fine particles in a liquid medium. These particles usually consist of polymers, which can be natural or synthetic in origin. The system appears as a colloid, where the solid components are suspended within the liquid, allowing for unique interactions. The importance of latices lies in their versatility; they can be used in various applications such as paints, adhesives, and medical formulations. Furthermore, latices exhibit properties that can be finely tuned based on their chemical composition and processing conditions.
Historical Context and Development
The exploration of latices has evolved significantly since their discovery. Early research focused primarily on natural latices, like rubber from the rubber tree, which was utilized for various practical applications. As science advanced, the need for more consistent and tailored materials led to the development of synthetic latices in the mid-20th century. Researchers sought to replicate the useful properties of natural latices while addressing limitations such as variability in source materials and processing challenges. The growth of the polymer industry catalyzed this transition, paving the way for innovative applications in coatings, sealants, and biomedical devices.
Classification of Latices
Latices can be primarily classified into two categories: natural latices and synthetic latices.
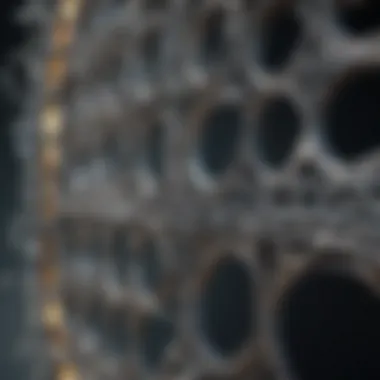
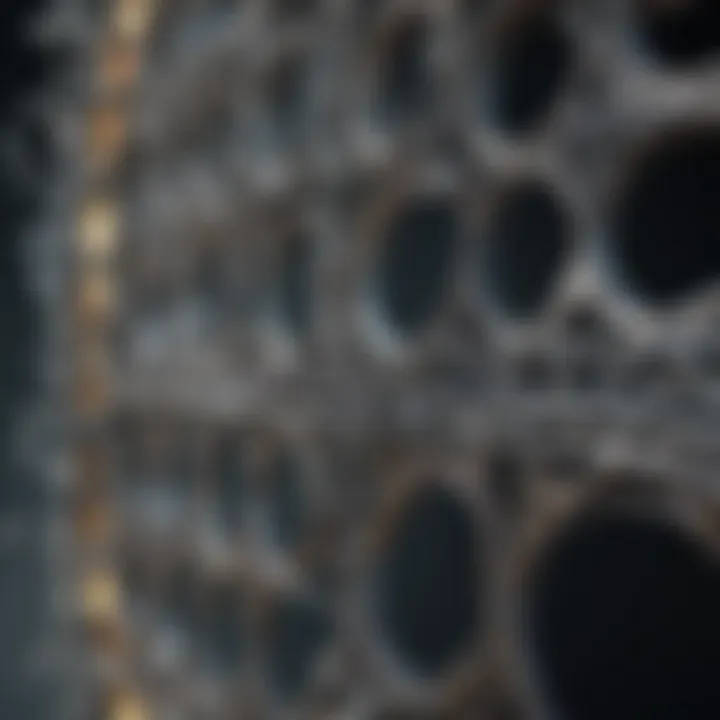
Natural Latices
Natural latices originate from plant sources, such as rubber trees and other flora. A key characteristic of natural latices is their biodegradability, which makes them an environmentally friendly option in several applications. This type of latex is popular in various industries like the automobile and healthcare sectors for its elasticity and durability. The complex composition of natural latices can offer unique mechanical properties. However, variability in composition can also pose challenges in consistency and quality control, making it less predictable for certain applications.
Synthetic Latices
Synthetic latices are produced through polymerization processes and consist of engineered polymers. A significant advantage of synthetic latices is their customizable nature; researchers can modify the chemical structure to achieve desired properties such as increased strength, heat resistance, or specific adhesion characteristics. This flexibility makes them exemplary for industrial applications, including paints and inks. However, their environmental impact can be a concern due to reliance on petrochemical sources and sometimes less favorable degradation profiles compared to natural alternatives.
Understanding the distinctions between natural and synthetic latices can illuminate their respective uses and limitations, effectively guiding research directions and practical applications.
The Chemical and Physical Properties of Latices
Understanding the chemical and physical properties of latices is crucial for numerous applications in scientific research and industrial processes. These properties determine how latices behave under different conditions, influencing their usability in fields like material science, drug delivery, and nanotechnology. A detailed study of these characteristics can reveal insights into how to manipulate latices for specific applications, leading to enhanced outcomes in both laboratory settings and real-world applications.
Rheological Properties
Rheology deals with the flow and deformation of materials. For latices, understanding rheological properties is significant because they affect how these materials can be processed and applied. The viscoelastic behavior of a latex, which encompasses both viscous and elastic responses, informs us about its stability and performance under stress.
Several factors influence the rheological properties of latices:
- Particle Size Distribution: Smaller particles may cause a slurry to behave differently compared to larger ones. This distribution can significantly affect viscosity.
- Solid Content: Increasing the solid content in a latex can lead to changes in flow behavior and stability. High solid content may result in thickening, impacting processing conditions.
- Temperature: Changes in temperature can alter the viscosity of latices, with higher temperatures often leading to decreased viscosity, thereby affecting application processes.
A practical understanding of rheology allows researchers and practitioners to optimize formulations for desired performance, ensuring that latices meet the demanding requirements of various applications.
Thermal Properties
Thermal properties play an important role in determining the stability and performance of latices across different environments. These properties include thermal conductivity, heat capacity, and degradation temperature. Understanding these thermal characteristics enables scientists to predict how latices behave when subjected to heat, which is critical for applications such as coatings and adhesives.
Key considerations include:
- Heat Stability: The ability of a latex to withstand elevated temperatures without degrading is essential, particularly for thermal applications and in environments with fluctuating temperatures.
- Heat Capacity: Knowledge of the specific heat capacity of different latices informs users about their energy requirements for heating and cooling processes in industrial applications.
- Freezing Point and Melting Point: Understanding these points is vital for applications that operate in temperature-extreme conditions. It helps in the design of products stable under various operational settings.
The mastery of thermal properties not only ensures the reliability of latex-based products but also aids in formulating new materials suited for specific thermal environments.
Optical Properties
The optical properties of latices refer to how these materials interact with light. This includes parameters like light scattering, transmission, and reflection. For many scientific and industrial applications, the optical behavior of latices is crucial. It affects everything from the visual quality of coatings to the performance of optical devices.
Several factors influence the optical properties of latices:
- Refractive Index: This is an important characteristic that determines how light bends when it enters or exits a latex material. Altering the refractive index can optimize coatings for specific illumination conditions.
- Scattering Coefficient: This reflects how much light is scattered by the particles within the latex. High scattering can lead to milky appearances in coatings, which may not always be desirable.
- Particle Morphology: The shape and arrangement of the particles within a latex can considerably influence its optical properties.
Understanding optical properties is essential not only for academic research but also for improving commercial products through enhanced visual and functional properties.
Applications of Latices in Various Disciplines
The application of latices spans across many scientific fields, illustrating their versatility and significance. They play crucial roles in biology, chemistry, and physics, enabling innovations and enhancing existing processes. Understanding these applications allows researchers to harness latices effectively, advancing both technology and medical science.
In Biology
Drug Delivery Systems
Drug delivery systems utilizing latices have significantly changed approaches to medicine. The specific aspect of targeted delivery is pivotal; it allows medications to be directed precisely where they are needed in the body. This enhances the effectiveness of treatments while reducing side effects. One key characteristic of these systems is their ability to encapsulate drugs effectively. This encapsulation protects the drug and facilitates a controlled release into the bloodstream.
The unique feature of drug delivery systems based on latices is their biocompatibility. This ensures that they do not induce adverse reactions in the body. However, challenges like the degradation of the carrier material and potential leakage of the drug remain concerning. Nonetheless, the advantages of improved efficacy and specific targeting often outweigh these disadvantages.
Biomedical Applications
In biomedical applications, latices serve as a foundation for various technologies, from diagnostics to tissue engineering. The focus on creating materials that interact well with biological tissues highlights the importance of latices in these fields. One significant characteristic is their tunable properties, which allow for customization based on the specific requirements of each application.
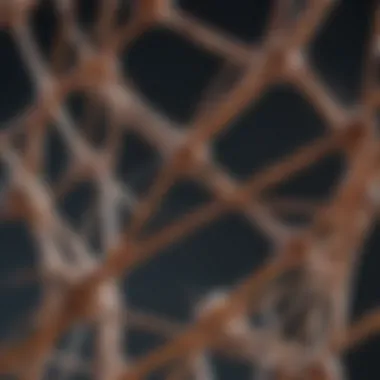
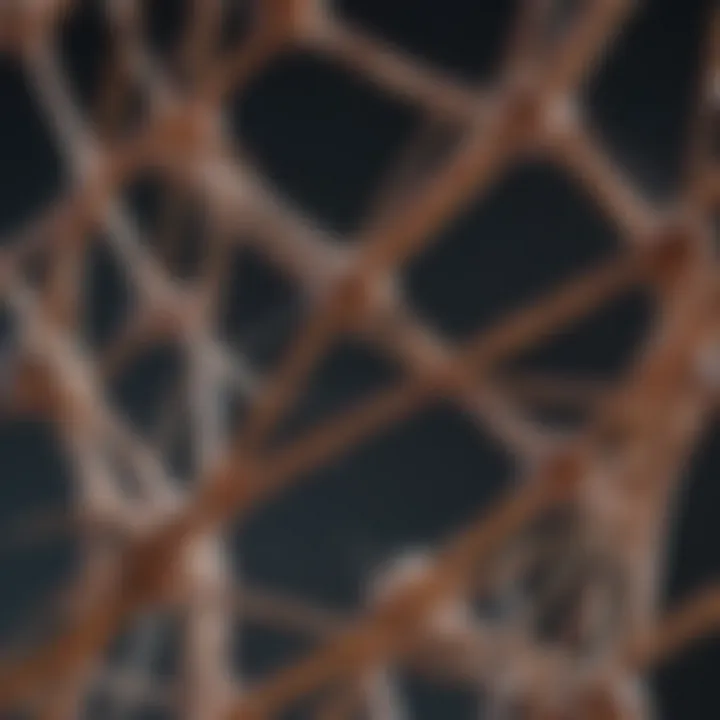
A unique aspect of biomedical applications is their role in the development of biosensors. These sensors can detect diseases at early stages, making them critical in healthcare settings. While the advantages are substantial, challenges such as regulatory approval and long-term stability of the materials exist. Still, the potential for saving lives makes these applications vital in scientific research.
In Chemistry
Polymer Science
In polymer science, latices are essential for innovative material development. They facilitate the production of polymers with unique characteristics. One key characteristic is their capacity to form films and coatings that enhance performance in various applications. This aspect allows for further exploration in developing durable materials.
A unique feature of latices in polymer science is their ability to create highly structured polymers. These structures can lead to materials with enhanced mechanical and chemical properties. Challenges lie in the consistency of production and the complexity of interactions within polymer blends. Nonetheless, their ability to create new materials positions latices as crucial in advancing chemical research.
Material Synthesis
The synthesis of new materials is another area where latices play a significant role. They enable the creation of composites that combine beneficial features from different substances. The key characteristic of these materials is their improved performance in specific applications, such as increased strength or reduced weight. This synthesis approach allows for the development of more efficient materials in various sectors.
A distinct feature of this process is the scalable production of materials, which is vital for commercial applications. However, the complexity involved in scaling up and ensuring uniformity can pose challenges. Despite these drawbacks, the ability to innovate and develop superior materials makes latices invaluable in material synthesis.
In Physics
Colloidal Systems
Colloidal systems involving latices are at the forefront of nanomaterials research. Their ability to manipulate particles at a nano-scale has vast implications. One essential aspect is the self-assembly of particles, allowing for the creation of complex structures. This characteristic is significant in the development of new technologies, including sensors and energy storage devices.
A unique feature of colloidal systems is their dynamic behavior in different environments. This can lead to novel properties that are not present in bulk materials. However, challenges include maintaining stability during processing. Despite such hurdles, the opportunities presented by colloidal systems make them a focal point of research in physics.
Nanotechnology
Nanotechnology benefits extensively from the use of latices. They provide a means to develop nanostructures with precise control over their properties. The key aspect of using latices in nanotechnology is their scalability, enabling the use of laboratory discoveries in commercial products. This characteristic is crucial for real-world applications.
An important feature of nanotechnology applications is their potential for significant advancements in electronics and medicine. Yet, there are concerns regarding safety and environmental impact. Balancing innovation with these concerns is vital for sustainable progress. Overall, latices represent a promising area for future research across various scientific disciplines.
Recent Advances in the Study of Latices
Recent research on latices has revealed significant insights that have implications across multiple scientific fields. The advances in this area can have a profound impact on material science, biology, and engineering applications. Understanding these developments is essential for researchers, as it guides future exploration and application of latices in practical scenarios.
One major area of focus has been on the functionalization of latices. Innovative methods to manipulate the chemical and physical properties of latices can lead to improved performance in various applications. This includes tailoring particle size and surface characteristics. These advancements not only enhance existing compounds but also allow for the creation of entirely new products that can meet specific demands.
Innovative Research Findings
In the realm of innovative findings, recent studies have reported groundbreaking results regarding the stability and behavior of latices under varying conditions. Researchers have observed that certain formulations of synthetic latices maintain their integrity even at elevated temperatures and pressures. This stability is crucial for applications in extreme environments, such as those found in aerospace engineering and deep-sea explorations.
Additionally, new approaches employing machine learning algorithms have expedited the development of latex-based formulations. These algorithms can predict properties of new latices based on existing data, dramatically reducing the time required for research and development. By utilizing big data, scientists can iterate faster and refine their products in a much shorter timeframe.
Technological Innovations
Technological innovations in the study of latices are continually evolving. Recently, methods such as 3D printing with latex materials have emerged, showcasing the versatility and adaptability of these compounds. This technique allows for the use of latices in creating complex structures with precision, suggesting untapped potential in architecture and customized medical devices.
Moreover, advancements in nanotechnology have enabled scientists to design and manipulate nanoparticles within latices. This not only enhances the mechanical and thermal properties of the latices but also opens doors to applications in drug delivery systems. Examples include the encapsulation of pharmaceuticals within latex particles, improving bioavailability and targeted delivery to specific tissues.
"The marriage of traditional latex study with modern computational techniques is reshaping our understanding and application of these materials."
As these advancements continue, they present both challenges and opportunities for researchers. Keeping pace with technological changes requires adaptability and continuous learning, ensuring that the scientific community remains at the forefront of latex research.
Interdisciplinary Research Involving Latices
Interdisciplinary research involving latices plays a crucial role in understanding the complex behaviors and applications of these materials across various scientific fields. By merging insights from chemistry, biology, physics, and engineering, researchers can develop innovative solutions to pressing challenges. Latices serve as a bridge between these disciplines, offering unique perspectives and methodologies that enhance scientific inquiry. This collaboration fosters a comprehensive knowledge base that informs practical applications, ranging from drug delivery systems to advanced materials.
Collaborative Studies
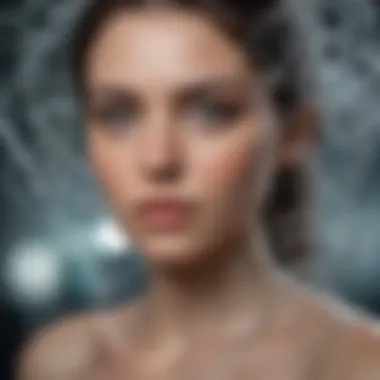
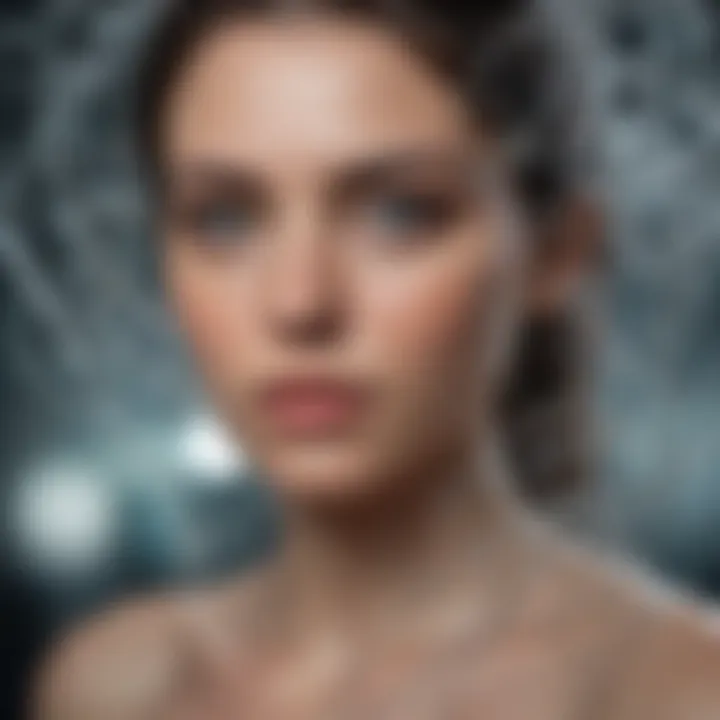
Chemistry and Biology
The intersection of chemistry and biology is critical in exploring the applications of latices, particularly in drug delivery systems. This field benefits significantly from understanding how latices can encapsulate therapeutic agents and facilitate their transport within biological systems. One key characteristic of this collaboration is the emphasis on molecular interactions, which determine the efficiency of these systems in targeting specific cells.
This combination has made it a popular choice for this article due to the increasing demand for precision medicine. The unique feature of this interdisciplinary approach is its ability to leverage biochemical principles alongside materials engineering. However, there are challenges, such as the need for extensive testing to ensure biocompatibility of these latices, which can complicate development processes.
Physics and Engineering
Physics and engineering also contribute significant insights into the study of latices, especially in the realm of colloidal systems and nanotechnology. Understanding the mechanical properties and stability of latices can lead to advancements in material design. The ability to manipulate scale and structure opens new pathways for applications in electronics, coatings, and other areas.
This collaboration is advantageous as it emphasizes empirical testing and application-oriented research, which is often favored in engineering disciplines. A unique feature here is the use of simulations and modeling to predict the behavior of latices under various conditions, improving product development time.
However, the complexity of physical laws governing material behavior can pose challenges, including difficulty in translating theoretical models into practical applications.
Case Studies Highlighting Interdisciplinarity
Case studies that focus on the interdisciplinary nature of latices demonstrate tangible benefits. One example includes the development of biodegradable drug delivery systems that merge chemical formulation with biological efficacy. Another case study may explore the use of latices in creating novel materials that respond dynamically to environmental changes by integrating principles from physics and engineering.
These examples underline how interdisciplinary research not only enriches the academic discourse but also leads to practical innovations. Collaborative efforts drive significant advancements, highlighting the potential of latices in practical contexts.
"Interdisciplinary research enhances creativity and innovation, leading the way to breakthroughs that single disciplines might not achieve alone."
Integrating findings from various fields provides a comprehensive understanding of latices, paving the way for future research initiatives that capitalize on their multifaceted capabilities.
Future Directions in Research on Latices
The exploration of latices marks a significant frontier in various scientific disciplines. It is essential to recognize the future directions in this area due to their potential to influence technology, health, and environmental sustainability. Increased understanding of latices can lead to improved materials, enhanced drug delivery methods, and innovative applications in nanotechnology.
As research progresses, multidisciplinary approaches will likely yield insights that can further optimize the properties and applications of latices. Collaboration among chemists, biologists, physicists, and engineers will become instrumental in realizing the full potential of these substances. By embracing upcoming trends, researchers can tackle challenges that may arise and uncover new opportunities for utilizing latices in impactful ways.
Emerging Trends
Emerging trends in the study of latices encompass several developments. A primary focus is on the synthesis of more sophisticated synthetic latices with tailored properties. This increasing sophistication allows for precise control over characteristics such as particle size and distribution, which can significantly influence the functionality of materials used in applications.
In addition, there is notable attention on the environmental impacts of latices, with a push toward sustainable practices in latex production and disposal. Researchers are investigating biodegradable and non-toxic alternatives, which can mitigate ecological risks associated with conventional synthetic materials.
Moreover, innovations in characterization techniques are evolving. Advanced imaging and analytical technologies can provide insights into the microstructure of latices, aiding researchers in understanding their behavior at the molecular level. This knowledge is crucial for harnessing their full potential in diverse applications.
Challenges and Opportunities
While the future seems promising, several challenges need to be addressed. The scalability of novel latex formulations often proves difficult. Initial laboratory successes may not always translate to industrial-scale production, leading to discrepancies in expected outcomes.
Regulatory issues also present hurdles. As latices find broader application across industries, ensuring compliance with safety standards and regulations will be critical. Adapting formulations to meet these requirements without compromising functionality can be challenging.
However, these challenges also provide unique opportunities for innovation. Addressing regulatory concerns can spur the development of new testing methodologies and standards in the industry. Furthermore, the demand for sustainable materials opens pathways for research focused on eco-friendly alternatives.
Engaging in interdisciplinary collaborations presents another opportunity. By uniting expertise from different fields, researchers can design solutions that are not only effective but also sustainable and safe. Building such connections can foster creativity and open up new avenues in research on latices.
In summary, the future of research on latices holds immense promise, shaped by emerging trends and reinforced by both challenges and opportunities. By navigating these dynamics, the scientific community can pave the way for impactful advancements.
Ending: The Importance of Latices in Scientific Research
Latices play a crucial role in various scientific fields, serving as versatile materials with unique properties. Their significance extends beyond simple applications, influencing advances in technology, medicine, and environmental science. In this section, we discuss several key aspects of why latices are essential to scientific inquiry and innovation.
1. Versatile Applications
Latices are utilized in diverse applications, including paints, adhesives, and drug delivery systems. Their ability to form stable dispersions enables them to improve product performance and efficacy. For example, in biomedical applications, latices facilitate targeted drug delivery, enhancing treatment effectiveness. This versatility is invaluable, as researchers continue to explore new uses in emerging fields like nanotechnology.
2. Interdisciplinary Collaboration
Research involving latices often necessitates collaboration across various scientific disciplines. By integrating knowledge from chemistry, biology, and materials science, researchers can develop innovative solutions to complex challenges. Collaborative studies have led to breakthroughs in fields such as polymer science and self-healing materials, showcasing the interconnectedness of scientific research.
"The dynamic nature of latices fosters collaboration, driving progress across fields and industries."
3. Continued Innovation
The exploration of latices is marked by ongoing advancements. Recent studies highlight innovative techniques for synthesizing novel latices with enhanced properties. These innovations can lead to improved material performance and new applications that were previously unexplored. As researchers push the boundaries of our understanding, latices will likely play an integral part in future scientific breakthroughs.
4. Environmental Considerations
As society becomes increasingly conscious of environmental impact, latices offer potential solutions. For instance, the development of eco-friendly materials based on natural latices can reduce the reliance on harmful chemicals. Research into biodegradable latices demonstrates a commitment to sustainability, making them a valuable focus for future studies.
In summary, latices are vital components in scientific research, offering a unique combination of properties and applications. Their role in interdisciplinary collaboration fosters innovation and addresses critical challenges across various fields. Continuing to explore the significance of latices will undoubtedly open new avenues of study and practical applications, solidifying their importance in the scientific landscape.