Understanding Damaged Mitochondria: Implications and Insights
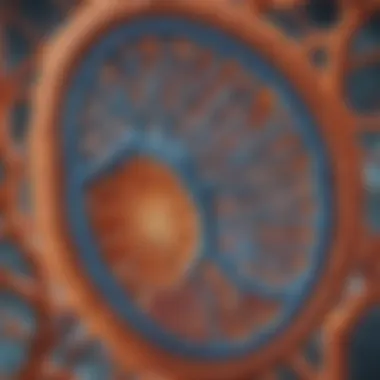
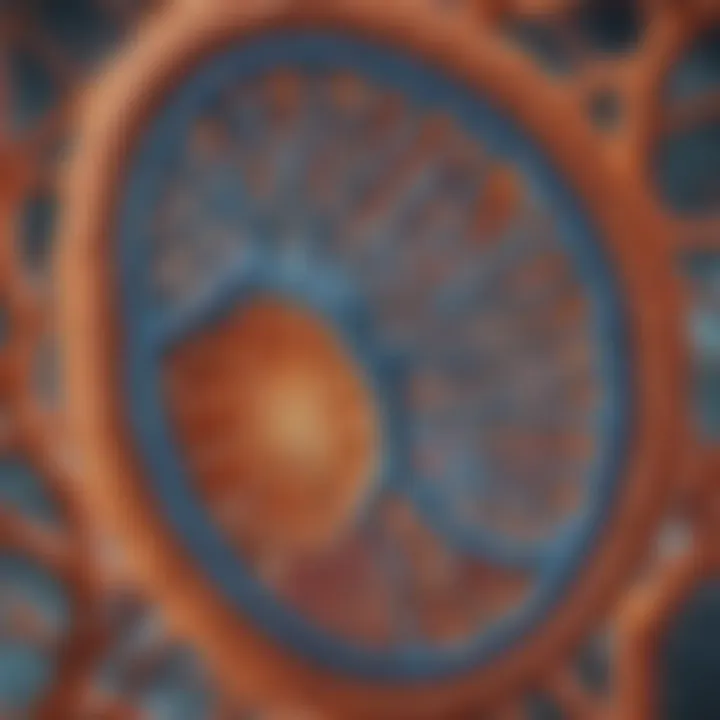
Intro
Mitochondria are essential organelles found within eukaryotic cells. Often referred to as the powerhouses of the cell, they are responsible for generating adenosine triphosphate (ATP), the primary energy currency of cellular functions. Beyond energy metabolism, mitochondria also play significant roles in regulating apoptosis and the generation of reactive oxygen species (ROS). However, mitochondrial dysfunction can lead to numerous health issues and is closely linked to aging.
Damage to mitochondria can have dire consequences on cellular health. The intricate balance of energy production and cellular signaling that mitochondria maintain is often disrupted by factors such as oxidative stress, environmental toxins, and genetic mutations. These elements contribute not only to local cellular effects but can also initiate systemic problems affecting whole organ systems. The aim of this article is to delve into these issues, unpack the complexities behind mitochondrial damage, and examine potential therapeutic strategies for restoring mitochondrial functionality.
Methodology
Overview of research methods used
The findings presented in this article are synthesized from a variety of research methods designed to assess mitochondrial health and dysfunction. A blend of experimental studies, clinical trials, and epidemiological studies provides a comprehensive approach to understanding mitochondria's role in health and disease.
Data collection techniques
Data collection techniques utilized in mitochondrial research include:
- Genetic analyses: Sequences of mitochondrial DNA are analyzed to identify mutations linked to dysfunction.
- Biochemical assays: Measurements of mitochondrial respiration and ATP production help assess functional capacity.
- Imaging techniques: Confocal microscopy and electron microscopy provide visual insights into mitochondrial morphology and distribution within cells.
- Patient surveys and clinical assessments: These methods are used in clinical trials to gather real-world data about symptoms and outcomes in individuals with mitochondrial disorders.
Implications of Mitochondrial Damage
Mitochondrial damage is associated with a range of diseases, including neurodegenerative disorders like Parkinson's and Alzheimer's, cardiovascular diseases, and metabolic syndromes. The cascading effects of mitochondrial dysfunction can promote cellular senescence and apoptosis, leading to tissue degeneration and organ failure.
Factors contributing to damage can be extensively categorized, including intrinsic factors such as genetic predispositions and environmental factors, like pollution or diet. Cumulative damage from ROS can specifically impair mitochondrial function, creating a vicious cycle of further oxidative stress and cellular deterioration.
Therapeutic Strategies
Recent research has focused on various therapeutic approaches for mitigating mitochondrial damage. Possible strategies include:
- Antioxidants: Compounds like Coenzyme Q10 target oxidative stress and aim to reduce damage.
- Mitochondrial biogenesis inducers: Nutrients like resveratrol may stimulate the production of new mitochondria.
- Gene therapy: Targeting specific genetic mutations with CRISPR technology may offer potential future benefits.
Future Directions
Upcoming trends in research
The future of mitochondrial research is promising, with ongoing development in biomarker discovery for mitochondrial dysfunction and new approaches for gene therapy. Researchers are increasingly utilizing high-throughput screening methods to identify novel compounds that enhance mitochondrial health.
Areas requiring further investigation
There remain areas that need more exploration, including:
- The role of the gut microbiome in influencing mitochondrial function.
- Long-term effects of novel therapeutic interventions on mitochondrial dynamics.
- How lifestyle factors, such as exercise and diet, can optimize mitochondrial function.
Understanding damaged mitochondria is not merely an academic exercise; it is a critical component of advancing medical knowledge and therapeutic development.
This article aims to provide in-depth insight into these factors and trends, paving the way for a more thorough understanding of mitochondrial biology. Through examining the implications of damaged mitochondria, we can better comprehend their role in health and disease, promoting future research aimed at restoring cellular harmony.
Prelude to Mitochondria
Mitochondria are essential organelles found in nearly all eukaryotic cells. They are often dubbed the powerhouses of the cell due to their critical role in energy production, specifically in the form of adenosine triphosphate (ATP). Understanding mitochondria is more than an academic exercise; it has immense implications for health, disease, and overall cellular function. As the article unfolds, we shall explore the intricacies and importance of these organelles, enlightening readers about their structure, function, and the consequences of their damage.
Definition and Function
Mitochondria are membrane-bound organelles that convert the chemical energy from food into ATP through oxidative phosphorylation. This process is vital because ATP serves as the energy currency of the cell, fueling various biological processes. Beyond energy production, mitochondria are involved in regulating metabolism, calcium homeostasis, and apoptosis, which is programmed cell death. In essence, they are multifaceted organelles critical for maintaining cellular harmony.
Mitochondrial Structure
The structure of mitochondria is complex, encompassing multiple components that each play significant roles. The key parts include:
Outer Membrane
The outer membrane of mitochondria serves several functions. Primarily, it provides a barrier that separates the mitochondrion from the cytosol. A key characteristic of the outer membrane is its permeability, allowing small molecules and ions to pass freely. This makes the outer membrane a popular choice in studies concerning transport mechanisms and membrane dynamics. Interestingly, the outer membrane also contains proteins known as porins, which facilitate the movement of metabolites. However, while its permeability is beneficial for metabolic processes, it also poses challenges in signaling and the regulation of ion concentrations.
Inner Membrane
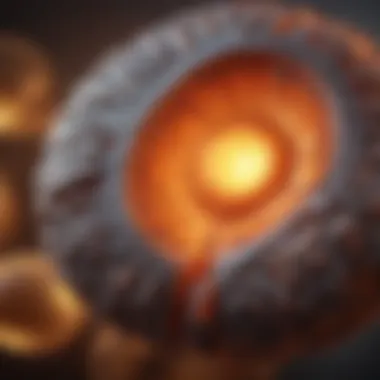
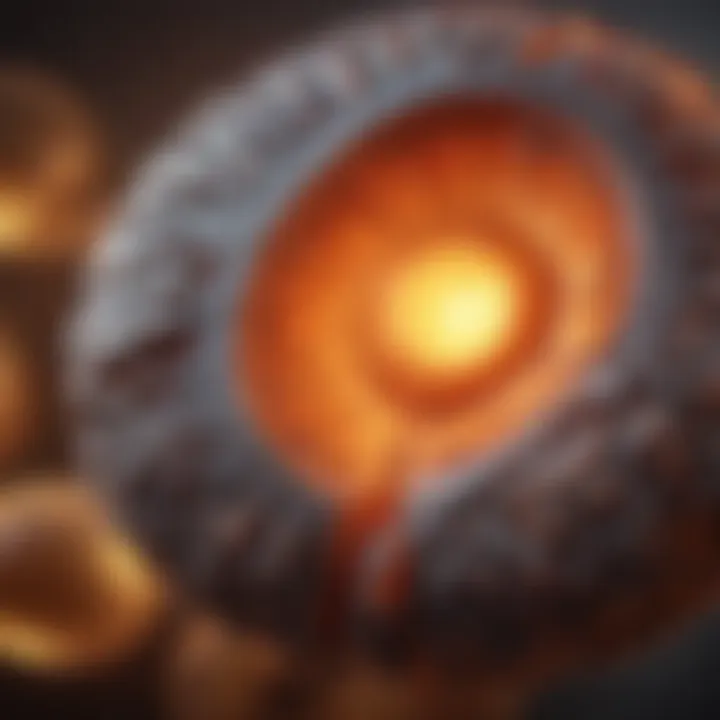
The inner membrane is where the magic of ATP production occurs. It is densely packed with proteins that perform the electron transport chain functions. A key feature of this membrane is its invaginations, known as cristae, which significantly increase its surface area, thus enhancing its capacity for energy production. This structural attribute makes the inner membrane highly beneficial for energy metabolism. However, the inner membrane's selectivity also means that it can restrict certain ions and substances, complicating its interaction with the rest of the cell.
Intermembrane Space
The intermembrane space lies between the outer and inner membranes. It plays a role in maintaining the proton gradient necessary for ATP synthesis. A vital characteristic of this space is its ability to accumulate protons, which are critical for the electron transport chain's efficiency. While this accumulation helps in energy production, any alterations or damage in this space can significantly impact mitochondrial function and overall cellular health.
Matrix
The matrix is the innermost compartment of the mitochondria and houses enzymes involved in the citric acid cycle (Krebs cycle), which is crucial for metabolic processes. One key feature of the matrix is its rich content in mitochondrial DNA, ribosomes, and various enzymes, which enable it to operate somewhat independently from nuclear DNA. This independence is advantageous for producing essential proteins and metabolic regulation. Conversely, mutations in the mitochondrial DNA can lead to dysfunction, emphasizing the importance of the matrix in ensuring overall mitochondrial health.
Causes of Mitochondrial Damage
Understanding the causes of mitochondrial damage is fundamental for grasping how these organelles influence cellular health. Mitochondrial dysfunction leads to various diseases and metabolic syndromes, making it critical to investigate what contributes to this damage. Identifying these factors allows for better preventative strategies and therapeutic interventions, enabling professionals to mitigate the harmful effects on health.
Oxidative Stress
Oxidative stress is a significant factor in mitochondrial damage. It occurs when there is an imbalance between the production of reactive oxygen species (ROS) and the body's ability to detoxify them or to repair the resulting damage. Mitochondria are primary sites for ROS production due to their role in energy metabolism. High levels of ROS can lead to mitochondrial DNA damage, protein oxidation, and lipid peroxidation. Over time, these effects contribute to the decline in mitochondrial function and cellular energy production.
Genetic Mutations
Genetic mutations can profoundly affect mitochondrial function. Mitochondrial DNA (mtDNA) mutations can arise from various sources, including inherited genetic disorders and environmental factors. These mutations can impair the electron transport chain, leading to decreased ATP production and increased ROS generation. This genetic decrease in efficiency can perpetuate a vicious cycle of mitochondrial impairment and cellular dysfunction, leading to the development of several age-related diseases and conditions.
Environmental Factors
Environmental factors play a pivotal role in inducing mitochondrial damage. They encompass a variety of elements, which can invoke stress on cellular systems. Several key environmental factors include:
Toxins
Toxins, including heavy metals like lead and mercury, can disrupt mitochondrial functions. These substances interfere with metabolic processes, leading to decreased ATP synthesis and increased oxidative stress. The key characteristic of toxins is their ability to enter cellular systems and elicit systemic responses that compromise mitochondria. Understanding the unique features of toxins is essential for developing preventative measures in affected populations.
Poor Nutrition
Poor nutrition negatively impacts mitochondrial health by depriving these organelles of essential nutrients. A diet low in antioxidants, vitamins, and essential fatty acids fails to provide the necessary substrates for optimal mitochondrial function. The unique feature of poor nutrition is its long-term influence on mitochondrial integrity, leading to cumulative damage over time. Strategies to improve diet quality can reverse some impacts of poor nutrition on mitochondria, highlighting its importance in overall health management.
Infection
Infections can also contribute to mitochondrial dysfunction, as pathogens often exploit mitochondrial processes to replicate. For instance, during a viral infection, the demand for ATP increases, straining mitochondrial capacity. The key characteristic of infection is that it can elicit both direct and indirect effects on mitochondrial health, exacerbating pre-existing conditions. These interactions pose a challenge for treatment approaches, often requiring a multifaceted therapeutic strategy to tackle the underlying infections while addressing mitochondrial damage.
Biological Implications of Damaged Mitochondria
Mitochondria are central to multiple biological processes in the human body. When these organelles become damaged, the implications can be profound. The interplay between mitochondrial dysfunction and overall cellular health is crucial. Understanding these biological implications reveals how damaged mitochondria affect energy metabolism, apoptosis regulation, and aging. These factors are essential in various diseases, making this topic relevant for students, researchers, and healthcare professionals alike.
Energy Production Decline
The primary role of mitochondria is to produce ATP, the energy currency of the cell. When mitochondria suffer damage, their ability to generate ATP declines significantly. This decline in energy production can affect various organs, the heart and brain in particular. Cells that require high energy, such as neurons and myocytes, are especially vulnerable.
Some of the key consequences of energy production decline include:
- Decreased Cellular Function: Cells may not perform optimally, leading to overall systemic dysfunction.
- Increased Fatigue: Reduced energy levels often result in fatigue, affecting daily life and overall health.
- Compromised Metabolism: Mitochondrial damage can lead to metabolic disorders, as ATP is necessary for various metabolic pathways.
The persistence of low energy levels can set off a cascade of biological issues, ultimately leading to the deterioration of health and increased disease susceptibility.
Apoptosis Dysregulation
Mitochondria play a critical role in the regulation of apoptosis, or programmed cell death. This process is essential for maintaining cellular homeostasis and eliminating damaged cells. Alterations in mitochondrial function disrupt this delicate balance, leading to either excessive cell death or survival of damaged cells. Such dysregulation is implicated in numerous diseases, including cancer, neurodegenerative disorders, and autoimmune diseases.
Key points to consider about apoptosis dysregulation include:
- Cancer Progression: Failure to undergo proper apoptosis can allow mutated cells to proliferate, contributing to tumor growth.
- Neurodegeneration: Excessive apoptosis in neuronal cells can lead to conditions such as Alzheimer’s and Parkinson’s diseases.
- Immune Disorders: Impaired cell death pathways can result in the accumulation of dysfunctional immune cells, exacerbating autoimmune conditions.
Understanding how mitochondrial dysfunction affects apoptosis could open pathways for targeted therapies aimed at restoring normal cell death processes.
Role in Aging
Aging is a complex process, and mitochondrial health is intricately linked to how we age at the cellular level. With age, mitochondria tend to accumulate damage from various sources, including oxidative stress. This accumulation compromises their function and is a critical factor in age-related diseases.
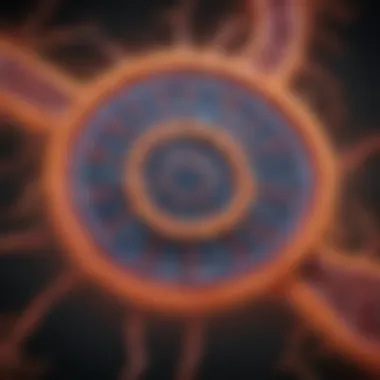
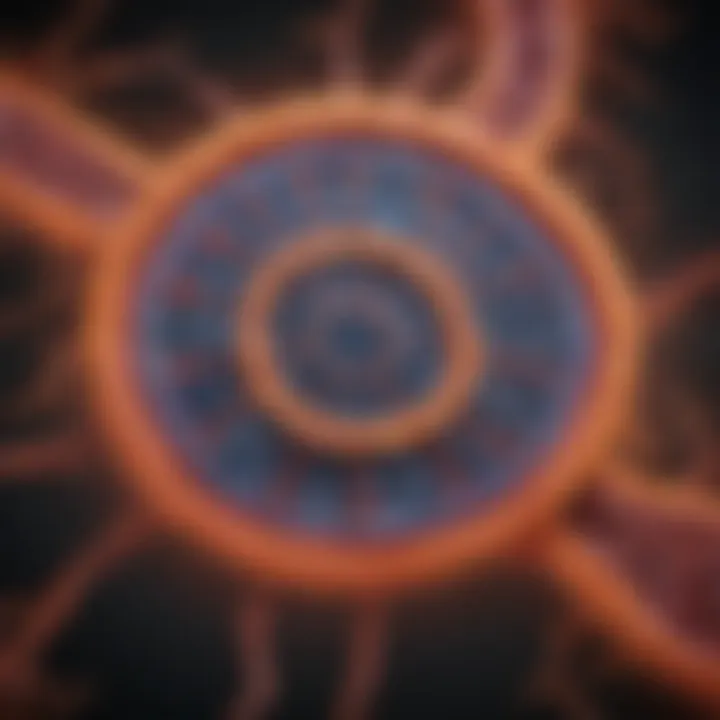
The implications of mitochondrial dysfunction in aging include:
- Increased Oxidative Stress: Aging cells exhibit higher levels of reactive oxygen species, leading to more substantial oxidative damage.
- Cellular Senescence: Damaged mitochondria trigger cell senescence, which contributes to age-related decline in tissue function.
- Chronic Diseases: Mitochondrial dysfunction has been associated with conditions like metabolic syndrome, cardiovascular diseases, and degenerative disorders.
"The study of mitochondria is crucial in elucidating the mechanisms behind various diseases and aging, opening potential avenues for targeted therapeutic strategies."
Further resources on mitochondrial biology can be accessed through Wikipedia and Britannica.
Diseases Associated with Mitochondrial Dysfunction
Mitochondrial dysfunction is a significant area of interest in understanding various diseases. These organelles are involved in a range of essential cellular processes, and their damage can lead to a spectrum of health issues. This section explores how damaged mitochondria correlate with different diseases, highlighting specific conditions that underscore the critical role mitochondria play in cellular health.
Neurodegenerative Disorders
Neurodegenerative disorders present a stark example of the consequences of mitochondrial dysfunction. These diseases often share a common pathological feature, which is the loss of neuron function and cell death.
Alzheimer's Disease
In Alzheimer's disease, mitochondrial dysfunction plays a crucial role in its pathology. One of the specific aspects is that it affects energy metabolism in neurons, leading to cognitive decline. A key characteristic of Alzheimer's disease is the accumulation of amyloid plaques, which disrupt cellular communication and metabolic processes. This makes it a relevant choice for deeper analysis, as understanding the mitochondrial dysfunction might open new avenues for intervention. Notably, a unique feature of Alzheimer's includes the presence of oxidative stress, which further compromises mitochondrial function. Addressing this could provide potential benefits to alleviating symptoms and slowing progression.
Parkinson’s Disease
Parkinson’s disease also demonstrates the critical interplay between mitochondrial health and neurodegeneration. The specific aspect here involves the degradation of dopaminergic neurons, which affects movement and coordination. A hallmark characteristic of Parkinson’s is the development of Lewy bodies, which are protein aggregates that can be impacted by mitochondrial impairment. This condition is a beneficial subject within this article as it emphasizes how mitochondrial damage contributes to the loss of neuronal integrity and function. The unique feature of Parkinson’s is its association with hereditary factors that can enhance mitochondrial dysfunction, posing both challenges and opportunities for research.
Metabolic Syndromes
Metabolic syndromes illustrate another dimension of mitochondrial dysfunction. They include conditions like diabetes and obesity, which have far-reaching implications for public health.
Diabetes
Diabetes is characterized by the body's inability to regulate blood sugar levels effectively, often linked to insulin resistance. Mitochondrial dysfunction in adipose and muscle tissues contributes to metabolic imbalances, which makes it a critical area for study. A prominent aspect of diabetes is the altered energy metabolism, which signifies why it is chosen for this article. The unique feature of diabetes lies in its widespread impact on vascular health, raising the risk for further complications if left unaddressed. Understanding the connection between diabetes and mitochondrial health can lead to more effective treatment strategies.
Obesity
Obesity, similarly, is connected to mitochondrial dysregulation. This condition is characterized by excessive fat accumulation and is directly linked to metabolic disease risk. A pivotal aspect of obesity is its connection to inflammation, which further exacerbates mitochondrial dysfunction. The choice of obesity is pertinent, given its growing prevalence globally. It presents unique considerations, as many of the strategies to combat obesity also target mitochondrial health, showing a direct avenue for potential interventions.
Cardiovascular Diseases
Finally, cardiovascular diseases are yet another sphere affected by mitochondrial dysfunction. These diseases often result in compromised cardiac function and increased mortality risk.
Mitochondrial damage can lead to impaired ATP production, critical for maintaining heart muscle integrity. A significant aspect to note here is how ischemia, the reduction of blood flow, can exacerbate mitochondrial damage, contributing to further cardiovascular complications. The key characteristics of cardiovascular diseases include hypertension and atherosclerosis, both of which are influenced by mitochondrial health. Understanding these links can provide insights into preventive measures and treatment options, demonstrating the systemic importance of mitochondrial integrity in overall health.
Detecting Mitochondrial Damage
Detecting mitochondrial damage is essential in understanding its impact on cellular health and overall physiological function. Mitochondrial dysfunction can contribute to various diseases and conditions, making early detection crucial for effective intervention. Identifying mitochondrial impairment enables researchers to develop targeted therapeutic strategies, ultimately improving patient outcomes.
Furthermore, recognizing the signs of damaged mitochondria can facilitate advancements in diagnostic techniques, allowing healthcare professionals to tailor treatment plans effectively. This section elaborates on the biomarkers associated with mitochondrial dysfunction and the diagnostic tools used to identify these issues.
Biomarkers of Mitochondrial Dysfunction
Biomarkers play a significant role in assessing mitochondrial health. These indicators help in diagnosing mitochondrial-related disorders by providing measurable evidence of dysfunction. Among the most prominent biomarkers are:
- Lactate: Elevated levels of lactate can signal a shift towards anaerobic metabolism, often linked to mitochondrial dysfunction.
- Reactive Oxygen Species (ROS): High ROS levels may indicate oxidative stress, often a byproduct of mitochondrial damage.
- ATP Production: Decreased ATP levels reflect impaired energy production capacity, serving as a telltale sign of damaged mitochondria.
- Mitochondrial DNA (mtDNA) Mutations: Specific mutations in mtDNA can either indicate damage or result from mitochondrial stress.
Identifying these biomarkers can assist in the early detection of mitochondrial dysfunction, providing valuable insights for researchers and clinicians.
Diagnostic Techniques
Biopsy
Biopsy is a crucial method for assessing mitochondrial damage. This technique involves extracting tissue samples to examine the pathological features of mitochondria. One key characteristic of biopsy is its ability to provide direct evidence of mitochondrial abnormalities. It is often considered a beneficial choice due to its specificity.
A unique feature of biopsy is the capability to analyze mitochondrial morphology under a microscope. This can uncover structural changes indicative of dysfunction. However, biopsies are invasive, which can be a disadvantage for patients needing repeated assessments.
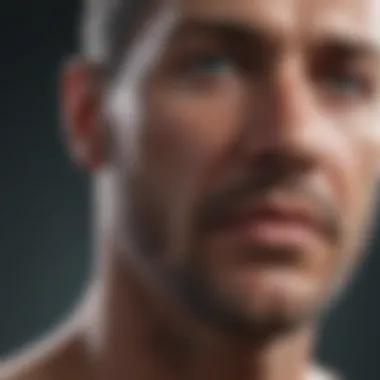
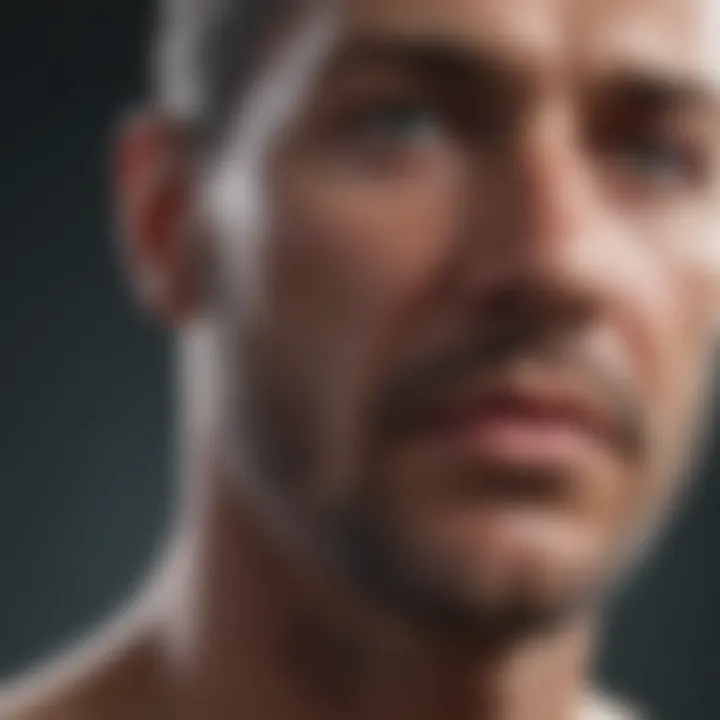
Imaging
Imaging techniques allow for non-invasive visualization of mitochondrial function. This includes methods such as MRI and PET scans. The key characteristic of imaging is its ability to monitor mitochondrial activity in real-time without the need for invasive procedures.
A prominent feature of imaging is the use of specific tracers to highlight areas of metabolic activity. Although beneficial for observing mitochondrial function, imaging methods may have limitations in resolution and sensitivity to detect subtle mitochondrial changes.
Genetic Testing
Genetic testing plays a pivotal role in diagnosing mitochondrial dysfunction by identifying mutations associated with these disorders. Its key characteristic lies in the ability to analyze DNA for specific mitochondrial genes. This method significantly benefits the early diagnosis of inherited mitochondrial diseases.
The unique feature of genetic testing is its precision in identifying genetic anomalies that contribute to mitochondrial impairment. On the downside, it may not provide immediate information regarding the functional state of mitochondria. Thus, it is often used in conjunction with other diagnostic techniques.
The integration of these diagnostic techniques will provide a comprehensive approach to detecting mitochondrial damage, leading to better understanding and treatment.
Therapeutic Strategies for Mitochondrial Restoration
The discussion on mitochondrial health cannot be exhaustive without focusing on therapeutic strategies for restoration. Mitochondria serve critical functions beyond mere energy production. Damage to these organelles influences systemic health and can lead to numerous diseases. Thus, exploring targeted therapies is essential. Restoration strategies aim to improve mitochondrial function and, consequently, overall cellular health. This section examines several approaches, emphasizing their significance in therapeutic discourse.
Antioxidants and Their Role
Antioxidants play a fundamental role in protecting mitochondria from oxidative stress. Reactive oxygen species accumulate as byproducts of mitochondrial metabolism. Excess amounts can lead to further damage. Antioxidants, such as vitamin E and coenzyme Q10, neutralize these harmful molecules. This action helps to maintain mitochondrial integrity. In supplement form, these antioxidants can be beneficial in mitigating oxidative damage. However, effectiveness may vary based on dosage, individual biochemistry, and other factors.
Mitochondrial Biogenesis
Increasing mitochondrial biogenesis is another essential therapeutic approach. This process enhances the number of mitochondria in cells, potentially improving energy metabolism. Key proteins, like PGC-1α, activate genes involved in mitochondrial growth. Exercise has been shown to induce mitochondrial biogenesis, signifying its relevance in health promotion. Various substances, such as resveratrol, also stimulate this process. However, one must monitor responses, as overstimulation may lead to other complications.
Pharmacological Advances
Advancements in pharmacological interventions offer promising avenues for restoring mitochondrial function. Treatments are focused on various pathways, targeting mitochondrial health.
Mitochondrial-targeted Therapies
Mitochondrial-targeted therapies are gaining interest due to their capacity to deliver drugs directly to the mitochondria. These therapies often involve using lipophilic cationic compounds, which accumulate in mitochondria. A notable example is the use of MitoQ, a modified form of coenzyme Q10. It is advantageous as it provides a concentrated dose directly where it is needed. However, caution is necessary as some treatments may produce unintended effects on other cellular components.
Gene Therapy
Gene therapy represents a cutting-edge approach. It aims to correct genetic defects that impair mitochondrial function. This therapeutic strategy can potentially restore normal function by delivering genetic material that promotes healthy mitochondrial activity. A critical advantage is its targeted nature, reducing systemic side effects associated with systemic therapies. However, this strategy can be complex and costly, requiring meticulous delivery methods and oversight.
"The future of mitochondrial medicine may heavily rely on resarch and the incorporation of various therapeutic strategies in clinical settings."
Future Directions in Mitochondrial Research
Research on mitochondria has evolved significantly in recent years. The future of mitochondrial research is crucial not just for understanding cellular metabolism, but also for addressing myriad diseases linked to mitochondrial dysfunction. Several aspects, including innovative approaches and potential therapeutic applications, shape this emerging field. Increased focus on mitochondrial health can lead to breakthroughs in preventative measures and treatments for a variety of conditions.
Innovative Research Approaches
Cutting-edge techniques are reshaping our understanding of mitochondrial dynamics. Researchers are now using advanced imaging technologies, such as super-resolution microscopy, to visualize mitochondria in real-time within living cells. This provides unparalleled insights into mitochondrial function and behavior under various physiological conditions.
Another promising area is the use of CRISPR-Cas9 gene editing to explore genetic mutations that affect mitochondrial function. This precision allows scientists to create models that mimic human diseases, thus unveiling the mechanistic underpinnings of mitochondrial dysfunction. Furthermore, metabolomics and proteomics are helping to profile mitochondrial metabolites and proteins, contributing to a more comprehensive understanding of their role in health and disease.
Collectively, these innovative approaches lead to a better understanding of how mitochondria adapt to stress and their role in different cellular pathways. This knowledge can guide future therapies aimed at improving mitochondrial function.
Potential Clinical Applications
As research unfolds, the clinical implications of mitochondrial studies become apparent. Potential applications may include targeted therapies for diseases such as Alzheimer’s, Parkinson’s, and various metabolic syndromes. Restoration of mitochondrial function could be a game-changer for addressing neurodegeneration.
For example, the identification of specific biomarkers for mitochondrial dysfunction might enhance early diagnostics. This could lead to earlier interventions and improved patient outcomes. Additionally, therapies developed from mitochondrial research, such as gene therapies or mitochondrial-targeted antioxidants, could hold promise in treating conditions rooted in mitochondrial damage.
Understanding the minute details of mitochondrial function can unlock significant advances in medicine, impacting a range of diseases from neurodegeneration to obesity.
As we look ahead, focusing on the relationship between mitochondria and disease will likely drive therapeutic innovation, emphasizing a holistic approach to mitochondrial health.
End
The topic discussed in this article highlights the complex role of mitochondria in cellular health and the implications of their damage. Understanding damaged mitochondria is essential, as these organelles are critical for energy production and metabolic regulation. When mitochondrial function declines, it can lead to a range of disorders, influencing both individual health and broader public health concerns.
Several specific elements emerge from the discussion. First, the identification of causes for mitochondrial damage is key. Factors like oxidative stress, genetic mutations, and environmental challenges contribute to dysfunction. Recognizing these influences can guide prevention and treatment strategies.
Secondly, the biological implications of damaged mitochondria cannot be overlooked. The decline in energy production affects not just individual cells but entire systems. Likewise, the dysregulation of cellular processes, such as apoptosis, plays a significant role in age-related diseases.
Furthermore, the diseases linked to mitochondrial dysfunction, such as neurodegenerative disorders and metabolic syndromes, stress the urgent need to understand this area better. As researchers explore innovative therapeutic strategies, including pharmacological advancements and mitochondrial biogenesis, the potential clinical applications look promising.