Exploring HPLC Techniques: Principles and Applications
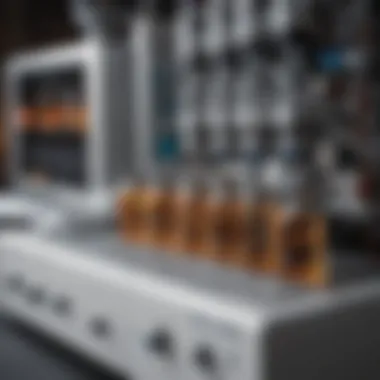
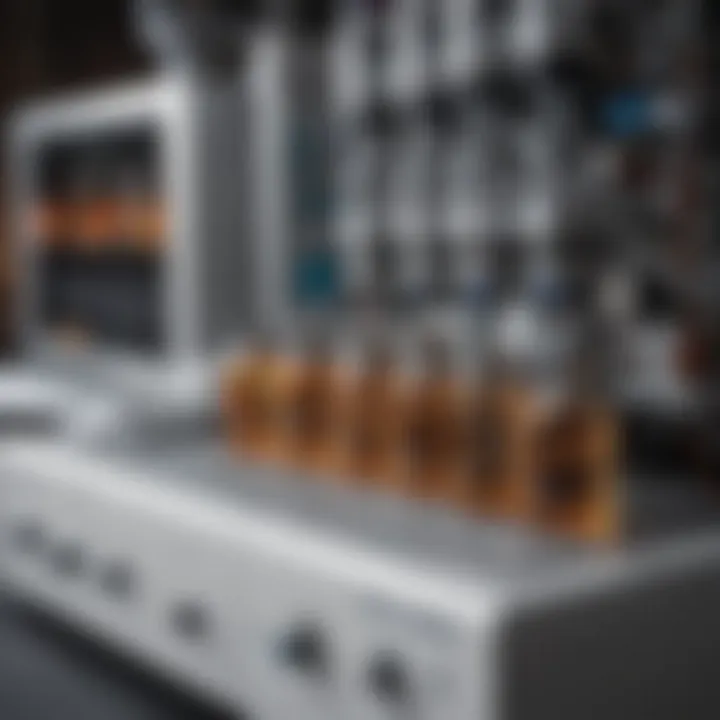
Intro
High-Performance Liquid Chromatography, commonly referred to as HPLC, serves as a cornerstone technique in analytical chemistry. Its significance cannot be overstated; HPLC is vital for separating, identifying, and quantifying components in various mixtures. This technique finds applications across a multitude of sectors, including pharmaceuticals, environmental testing, and food safety.
The article aims to provide a comprehensive examination of HPLC techniques, articulating the underlying principles, essential components, and method development processes. By dissecting the complexities associated with HPLC, readers will gain a deeper understanding tailored to their field of expertise. Prospective readers, whether students, researchers, or professionals, will find value in both the theoretical foundations and practical considerations that HPLC entails.
As this narrative unfolds, an exploration of the principles will set the stage for understanding the equipment involved and how it is utilized for effective results. The subsequent sections will thoroughly discuss the methodical approaches involved in optimizing HPLC results and interpreting the outcomes successfully.
Foreword to HPLC
High-Performance Liquid Chromatography (HPLC) has become an essential analytical tool in numerous fields such as chemistry, biochemistry, and pharmaceuticals. This introduction elucidates the significance of HPLC while outlining core concepts that will be explored in the article. HPLC’s ability to separate, identify, and quantify compounds lends itself well to rigorous scientific inquiry and application.
The sections to come will delve into its foundational principles, integral components, methodical development, widespread applications, and the interpretation of results. Each segment is tailored to furnish the reader with an understanding of HPLC’s intricate mechanics. In essence, this section serves as a launching pad for the detailed exploration that follows.
Definition and Overview
HPLC is a sophisticated technique used for separating mixtures of compounds in a liquid phase. By applying high pressure, it moves solvent through a column packed with solid material, allowing distinct components of the sample to exit at various times, known as retention times. Each component in the sample interacts differently with the stationary phase of the column, leading to separation.
HPLC showcases versatility in its applications; it can be utilized for analyzing small molecules, proteins, and nucleic acids. This adaptability reinforces its relevance across various industries. The core benefit lies in its ability to provide precise, reproducible results, which is particularly critical in the pharmaceutical domain where the safety and efficacy of products must be assured.
Historical Background
The roots of HPLC date back to the mid-20th century. The technique evolved from traditional liquid chromatography, which relied on gravity for solvent movement. The transition to high pressure began in the 1960s, leading to significant enhancements in resolution and speed. Pioneers such as Walter Hawley and his colleagues played pivotal roles in refining the methodology and expanding its application scope.
During the 1970s, the first commercial HPLC systems became available, rapidly advancing research methodologies. The 1980s saw a surge in the development of sophisticated detectors, such as UV-visible and fluorescence detectors, further enhancing analysis capabilities. Today, HPLC stands as a cornerstone technique in analytical labs, continuously improving with innovations in technology and methodology.
Principles of HPLC
High-Performance Liquid Chromatography (HPLC) is a sophisticated technique that serves as the backbone of analytical chemistry. Understanding the principles of HPLC is essential for anyone looking to delve into method development or analysis in various scientific fields. The effectiveness of HPLC lies in its ability to separate complex mixtures with precision and accuracy. Familiarizing oneself with these principles allows researchers to optimize conditions for specific applications and ensure reliable results.
Basic Concepts
At the core of HPLC are several basic concepts that many practitioners must grasp. These include:
- Phases of Separation: HPLC operates on the basis of a mobile phase and a stationary phase. The mobile phase is typically a liquid solvent that transports the sample through the stationary phase, which can be a solid or a liquid-coated solid.
- Retention Time: Each substance in the mixture interacts differently with the stationary phase, causing them to migrate at different rates. This differential migration is measured in retention time, a vital parameter for identifying compounds.
- Pressure and Flow Rate: HPLC systems operate under high pressure, enabling the use of smaller particle sizes in columns, which leads to better separation efficiency. The flow rate of the mobile phase can also influence the separation and detection of analytes.
- Homogeneity of Samples: A well-mixed sample is crucial for achieving accurate results. Incomplete mixing can lead to poor separation and inaccuracies in quantification.
Mastering these basic concepts is the first step toward effective HPLC analysis. Each concept impacts the overall performance and output of HPLC techniques, necessitating careful consideration during method development.
Separation Mechanisms
Separation mechanisms in HPLC are primarily based on the interactions between the stationary and mobile phases. The interplay of these interactions defines the efficiency and selectivity of the chromatography process. Here are the main types of separation mechanisms:
- Adsorption Chromatography: This mechanism relies on the adsorption of the analyte onto the stationary phase. Polar compounds tend to adsorb more strongly, migrating slower through the column. This principle is useful for separating organic compounds from mixtures.
- Partition Chromatography: In partition chromatography, analytes distribute between the mobile phase and a stationary liquid film on the solid. This method is effective for separating compounds based on their solubility in varying solvents.
- Ion-Exchange Chromatography: This mechanism involves the interaction of charged analytes with oppositely charged sites on the stationary phase. It is particularly useful for separating ionic compounds or adjusting the pH of the mobile phase to improve separation performance.
- Size-Exclusion Chromatography: Size-exclusion or gel permeation chromatography separates molecules based on their size. Larger molecules pass through the stationary phase more quickly, whereas smaller molecules take longer as they can enter the pores of the stationary material.
Understanding these mechanisms enhances the ability to choose the appropriate method for specific applications. HPLC's versatility allows scientists to tailor their approaches based on the characteristics of the analytes being studied.
"The principles of HPLC not only define its function but also open pathways for innovative analytical solutions across various research disciplines."
By mastering the principles of HPLC, researchers lay the groundwork for successful application in fields such as pharmaceuticals, environmental science, and food analysis. This knowledge serves as the foundation from which the intricacies of the method can be further explored.
Components of HPLC Systems
High-Performance Liquid Chromatography (HPLC) relies heavily on its core components to function correctly and deliver accurate results. Each component has a specific role that contributes to the overall operation of the HPLC system. Understanding these components is key for students, researchers, and professionals aiming to optimize their analytical processes.
Pump Mechanism
The pump in an HPLC system is fundamental for mobile phase delivery. It creates a precise flow of the solvent through the system and maintains consistent pressure. The capacity to control the flow rate and pressure is critical. Variations can greatly affect separation efficiency and retention times. Today, many advanced pumps provide features like gradient elution, where the composition of the mobile phase changes during the run. This flexibility allows for better separation of complex mixtures. So, it is important to consider both mechanical efficiency and versatility when selecting a pump mechanism.
Injection Systems
Injection systems are essential for introducing samples into the HPLC system. They ensure that the sample is injected consistently and at the right volume. One common type of injection system is the autosampler, allowing for high-throughput analysis by automatically injecting samples from vials. There are several considerations in this area:
- Precision: A precise injection system minimizes the variability between injections.
- Sample volume: The system must accommodate the required volume for analysis without causing issues with resolution or sensitivity.
- Contamination: Maintaining cleanliness to avoid contaminants in samples is critical.
Selecting the right injection system influences the reliability and accuracy of the results in analytical applications.
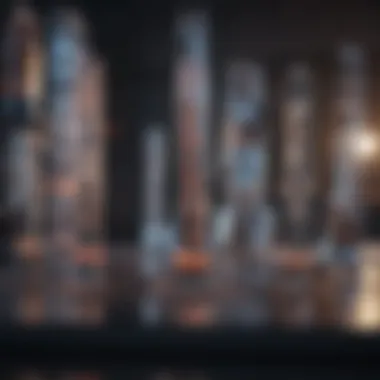
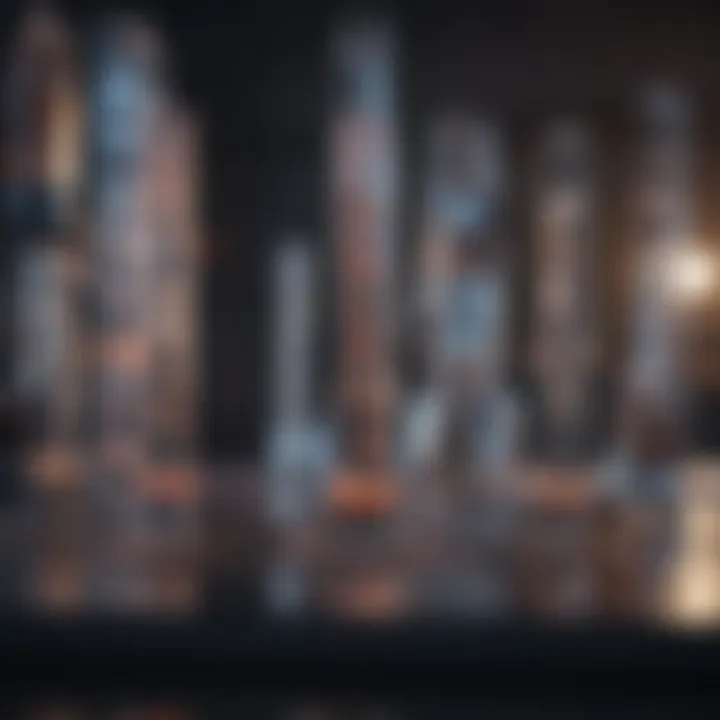
Columns
Columns in HPLC are where the actual separation occurs. They contain stationary phases that interact selectively with the components in the sample. Different column types provide unique separation characteristics. Key considerations include:
- Column length and diameter: These factors influence the resolution and analysis time. Longer columns can offer better separation but may increase analysis time.
- Stationary phase chemistry: The choice of stationary phase material affects the retention and separation of analytes.
- Column temperature: Controlling temperature during analysis can improve separation efficiency, especially for thermally sensitive compounds.
Understanding the intricacies of column selection allows users to fine-tune their HPLC methods to achieve optimal results.
Detectors
Detectors are pivotal for signal generation in HPLC, capturing data on the separated components as they emerge from the column. Various types of detectors exist, each suitable for specific applications. Most commonly used detectors include:
- UV-Vis Detectors: Sensitive to compounds that can absorb ultraviolet or visible light, widely used for analyzing components at specific wavelengths.
- Fluorescence Detectors: Highly sensitive to compounds that fluoresce, used for trace analysis.
- Mass Spectrometers: When integrated with HPLC, mass spectrometers provide molecular weight and structure information, enhancing identification capabilities.
When selecting detectors, factors such as sensitivity, specificity, and the type of analytes are essential. Choosing the right detector can significantly impact the analysis quality.
"Each component of an HPLC system plays a crucial role in achieving accurate and reproducible results in analytical chemistry. Understanding these components helps in method development and troubleshooting."
Optimizing the HPLC system's components is not merely a technical task; it is a necessity for achieving robust outcomes in various scientific disciplines. Understanding the roles and interactions of the pump, injection system, columns, and detectors is fundamental for anyone involved in HPLC analysis.
Method Development in HPLC
The process of method development in HPLC is crucial for achieving reliable and reproducible results. It entails tailoring the analytical method to suit specific parameters required for different analyses. A well-developed method can significantly impact the quality of data obtained, which is essential for meeting regulatory standards and scientific validity. In the context of this article, understanding method development is indispensable for anyone aiming to enhance their analytical capabilities.
Optimizing Conditions
Optimizing conditions involves fine-tuning various factors to achieve the best results. Key factors include flow rate, temperature, and mobile phase composition. Altering the flow rate can affect both analysis time and resolution. A higher flow rate often decreases the time taken for the analysis, but may lead to peak broadening. Temperature adjustments help optimize interaction with the stationary phase. It is beneficial to determine the optimal conditions for different analytes to ensure that the resolution and sensitivity requirements are met.
- Flow Rate: Varies analysis time and resolution.
- Temperature: Affects viscosity and analyte interactions.
- Mobile Phase Composition: Crucial for separation effectiveness.
By assessing these parameters, analysts ensure maximum performance from the HPLC system. Overall, methods must be optimized systematically to balance speed and accuracy, enhancing overall method reliability.
Solvent Selection
Selecting an appropriate solvent is fundamental in method development. Solvents influence solubility, separation efficiency, and the interaction with the stationary phase. A mismatch in solvent properties can lead to poor separations or ineffective analyte elution. A few points to consider when choosing solvents include:
- Solubility of Analytes: Ensure the chosen solvent can dissolve the analytes adequately.
- Polarity: Match the solvent polarity with that of the stationary phase to optimize retention.
- Viscosity: Affects flow rates and gradient development.
Common choices for solvents include acetonitrile, methanol, and water. Each one brings its unique properties to the table, influencing the method outcomes distinctly. Therefore, the solvent selection phase demands careful consideration, often requiring trial and error to identify the optimal conditions for specific applications.
Validation of Methodologies
Validation is an essential part of method development. It ensures that the developed method is reliable, reproducible, and fit for the intended use. Validation includes various aspects such as specificity, sensitivity, accuracy, precision, and robustness. Each element plays a critical role in determining the overall credibility of the method.
Key aspects of validation include:
- Specificity: Ability to measure the analyte distinctively amidst other components.
- Sensitivity: Determining the lowest concentration of an analyte that can be detected reliably.
- Accuracy: How close the experimental results are to the true value.
- Precision: The consistency of results when the method is repeatedly applied.
Validating methodologies should conform to established standards, such as those laid out by the International Conference on Harmonisation (ICH). Comprehensive validation not only bolsters confidence in the analytic method but is also necessary for regulatory compliance.
"Rigorous validation processes ensure that the HPLC methods are dependable and can withstand scrutiny across various analytical scenarios."
Applications of HPLC
High-Performance Liquid Chromatography is integral in diverse fields. Understanding the applications of HPLC enhances its value in various chemical analyses. This section delves into crucial applications in pharmaceutical analysis, environmental testing, and food and beverage quality control. Each of these domains showcases its unique benefits, driven by technology's advancements in HPLC.
Pharmaceutical Analysis
Pharmaceutical analysis is a primary application of HPLC. It ensures the efficacy and safety of drugs. HPLC assists in the quantification of active pharmaceutical ingredients (APIs) and also checks for impurities. The regulatory requirements demand strict adherence to quality control measures. HPLC plays a critical role here.
The method's precision allows for reliable analysis. For example, monitoring drug stability over time requires accurate measurements, usually done by HPLC. Moreover, the fast separation can speed up drug formulation processes, enabling quicker time-to-market for new medications.
Environmental Testing
Environmental testing is another significant area where HPLC’s capabilities shine. This technique is applied to monitor pollutants in water and soil samples. HPLC can detect trace levels of contaminants. This sensitivity is crucial for environmental protection efforts.
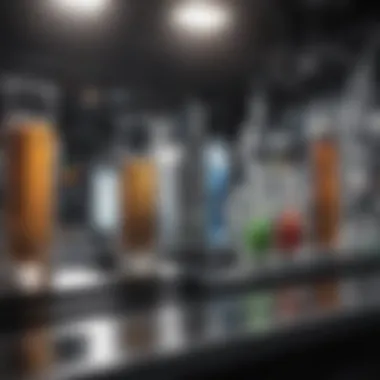
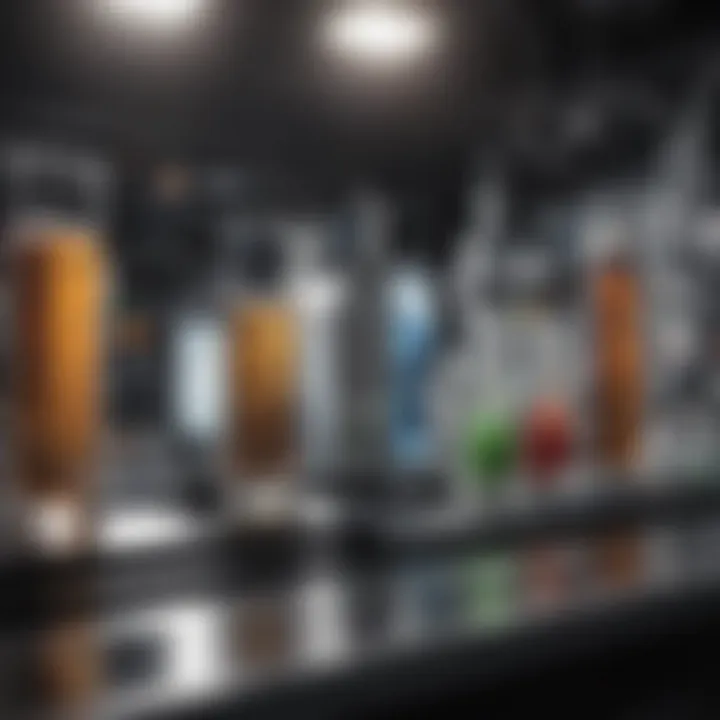
Common applications include the assessment of pesticide residues in agricultural soils or water sources. Detecting these residues helps in evaluating ecological risks. Importantly, HPLC can identify both organic and inorganic pollutants, making it versatile. As regulations on environmental safety evolve, the role of HPLC in this field becomes increasingly vital.
Food and Beverage Quality Control
In the food and beverage industry, HPLC ensures quality and safety standards are met. It is used to analyze food ingredients, additives, and contaminants. For instance, it helps in verifying the authenticity of natural flavors or assessing the levels of preservatives in packaged foods.
This method also evaluates nutritional content, such as vitamins and amino acids. It contributes to label accuracy and compliance, which is essential for consumer trust. Additionally, the method's ability to work with various matrices makes it valuable for analyzing complex food matrices.
HPLC is essential for maintaining the integrity of products in pharmaceuticals, environment, and food sectors.
Interpreting HPLC Results
Interpreting HPLC results is crucial for any researcher aiming to extract valuable insights from their chromatographic analyses. HPLC generates complex data in the form of chromatograms, which, when correctly interpreted, can reveal critical information about the purity, concentration, and identity of compounds in a sample. This section explores two primary elements: understanding chromatograms and quantification techniques. Both areas are essential for drawing accurate conclusions in various research contexts.
Understanding Chromatograms
Chromatograms serve as graphical representations of the separation process. They illustrate the signal intensity of the detector against time. Each peak corresponds to a different compound within the mixture. The retention time of each peak is a unique marker, allowing scientists to identify substances based on prior knowledge or by using calibration standards.
Key considerations when interpreting chromatograms include:
- Peak Area and Height: The area under the peak often correlates with the concentration of the analyte. Understanding how to measure this accurately is essential for quantification.
- Baseline Resolution: An ideal chromatogram should display a well-defined baseline. Issues like noise and drift can complicate interpretation.
- Retention Time Consistency: Each compound should ideally produce a consistent retention time across analyses, which aids in its identification and characterization.
Accurate interpretation of chromatograms can lead to thoughtful conclusions about product quality, purity, and composition.
Quantification Techniques
Quantification is a process used to determine the amount of a specific analyte in the sample. There are several techniques used in HPLC quantification, each with its strengths and weaknesses. Understanding these methods is necessary for producing reliable results.
- External Calibration: This involves preparing standard solutions at known concentrations. By plotting the peak area against concentration, a calibration curve can be generated. This method is straightforward and widely used in practice.
- Internal Standards: In this approach, a known quantity of a substance is added to the sample before analysis. The peak area ratio of the analyte to that of the internal standard is used for quantification, providing a more reliable analysis by compensating for variations in injection volume and detector response.
- Response Factor Method: It uses the response of the detector to the analyte and a standard, allowing for the conversion of peak area into concentration using a response factor.
Each technique may be suitable for different applications, and the choice often depends on the sample matrix and required accuracy. The adequacy of the selected method should be verified through validation studies, ensuring that results are not only accurate but also reproducible.
"The ability to interpret HPLC results accurately is vital for decision-making in both research and industry settings."
In summary, interpreting HPLC results demands a solid understanding of chromatograms and quantification techniques. A comprehensive grasp of these areas contributes significantly to the reliability and validity of analytical outcomes.
Common Problems and Solutions
Understanding the common problems associated with High-Performance Liquid Chromatography (HPLC) is essential for any serious practitioner in the chemistry field. Issues such as peak broadening and baseline noise can significantly impact the accuracy and reliability of your results. Addressing these challenges not only improves method performance but also enhances the overall quality of analysis. By identifying and rectifying these problems, one can ensure that the HPLC system functions efficiently and produces reproducible results.
Peak Broadening Issues
Peak broadening is a common challenge that affects the separation quality in HPLC. It leads to a loss in resolution, which directly impacts the ability to distinguish between closely eluting compounds. Several factors contribute to peak broadening, including:
- Column overload: When too much sample is injected, it can exceed the column's capacity, leading to distorted peaks.
- Inadequate particle size: Larger stationary phase particle sizes can cause increased diffusion and elongation of the peak.
- Temperature fluctuations: Changes in temperature can alter viscosity and affect mass transfer, resulting in broader peaks.
To mitigate peak broadening, it's important to optimize injection volumes, select appropriate particle sizes, and maintain stable temperature conditions during the analysis. Regular column maintenance and monitoring of the HPLC system can also aid in identifying early signs of issues. By addressing these factors, analysts can significantly improve peak shapes and enhance resolution, allowing for more accurate quantification of analytes.
Baseline Noise and Drift
Baseline noise and drift present major obstacles in reliable HPLC analysis. They can obscure the true signals from analytes, complicating quantification and interpretation of results. Baseline noise is typically caused by:
- Electromagnetic interference: External electrical sources may introduce noise into the system.
- Inconsistent solvent quality: Impurities in solvents can lead to erratic baseline readings.
- Improper detector settings: Incorrectly set parameters can amplify disturbances in the signal.
To combat baseline issues, careful selection of high-purity solvents and thorough system flushing are recommended. It's also advisable to optimize detector settings and eliminate sources of electromagnetic interference. Continuous monitoring of the baseline during runs can help in quickly detecting variations that could affect outcome reliability. By minimizing noise and drift, the clarity and accuracy of chromatographic results are markedly improved, thereby ensuring trustworthy data collection.
Ensuring optimal performance in HPLC requires vigilance and attention to detail in addressing peak broadening and baseline problems. Regular maintenance is key to reliable results.
Emerging Trends in HPLC Techniques
Emerging trends in High-Performance Liquid Chromatography (HPLC) signify the continuous evolution of this analytical method. As science progresses, the demand for more efficient and precise techniques becomes essential. This section discusses notable trends like miniaturization, automation, and integration with other technologies. Each trend shapes the future landscape in analytical chemistry, offering enhanced performance, reduced costs, and improved accuracy.
Miniaturization and Automation
Miniaturization in HPLC refers to the design and use of smaller systems without sacrificing performance. This trend is critical as it supports reduced reagent consumption, less waste, and lowers operational costs. Miniaturized systems often enable faster analysis times while maintaining high resolution and sensitivity.
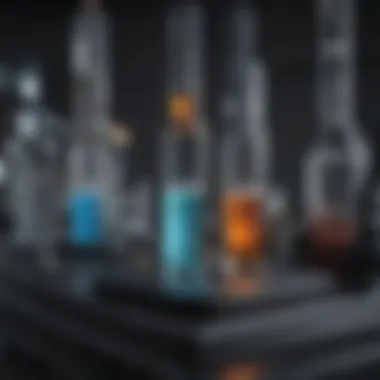
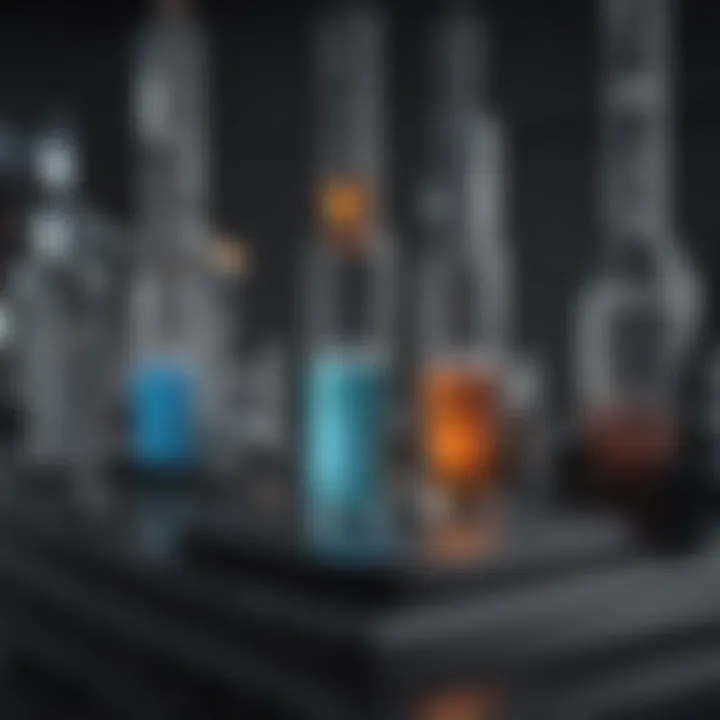
Automation is closely linked with miniaturization. With advanced robotic systems and software integration, procedures that once required extensive manual intervention can now be performed automatically. This shift minimizes human error and increases throughput in laboratories. Automated systems not only enhance efficiency but also allow for consistent reproducibility across multiple analyses.
Benefits of miniaturization and automation include:
- Reduced sample and solvent consumption
- Increased speed for high-throughput screening
- Streamlined workflows with minimal hands-on time
The ongoing development in microfluidics further enhances miniaturization in HPLC. This approach enables precise control of fluid transport at the microscale, making it possible to conduct complex analyses with just minute sample volumes. Micro HPLC systems are increasingly popular in various fields, from pharmaceutical development to environmental monitoring.
Integration with Mass Spectrometry
Integrating HPLC with mass spectrometry (MS) represents a significant advancement in analytical chemistry. This combination leverages the strengths of both techniques. HPLC efficiently separates components in a mixture, while mass spectrometry provides detailed analysis of the separated compounds. By linking these two methods, scientists can achieve enhanced sensitivity and specificity in identifying substances.
Mass spectrometry's capability to quantify compounds accurately allows researchers to perform an array of experiments with complex mixtures. This integration is often termed HPLC-MS. It is particularly useful in characterizing biological samples, environmental samples, and drug formulations.
Key considerations for HPLC-MS integration include:
- Sensitivity: Mass spectrometry is highly sensitive, enabling detection of trace levels of analytes.
- Versatility: This setup is applicable across multiple fields, including proteomics, metabolomics, and environmental science.
- Data richness: The combination generates rich datasets, contributing to a deeper understanding of sample composition.
The increasing adoption of HPLC-MS is evident as institutions seek to push the boundaries of analytical methods. The result is a more robust approach to addressing modern challenges in research.
"The integration of HPLC with mass spectrometry has transformed how we analyze complex samples, providing both separation and identification in one powerful technique."
Future Directions in HPLC Research
Future directions in High-Performance Liquid Chromatography (HPLC) are essential as they pave the path for innovation and improvements in analytical methodologies. The evolution of HPLC is driven by the need for more efficient, accurate, and adaptable systems. As industries rely heavily on precise analytical techniques, exploring future trajectories in HPLC holds significant relevance in enhancing research and application.
These advancements are not mere upgrades; they are critical for meeting the ever-increasing demands of various fields, including pharmaceuticals, environmental science, and food safety. Key elements in this evaluation include:
- Technological Integration: As HPLC systems become more complex, integrating advanced technologies will enhance performance.
- Cost-Efficiency: New methods aim to reduce operational costs without sacrificing quality.
- Sustainability: Researching greener solvents and energy-efficient systems is gaining traction.
Understanding these developments is vital for anyone engaged in HPLC research, as they will directly influence analytical strategies employed in diverse applications.
Advancements in Column Chemistry
The composition and design of columns in HPLC are central to improving separation efficiency and resolution. Recent advancements focus on new materials and innovative column designs.
New stationary phases developed from novel polymers enhance selectivity and stability, allowing for a broader range of analytes. These improvements lead to:
- Higher Resolution: Advanced materials facilitate better separation of closely related compounds, crucial in pharmaceuticals.
- Longevity: Innovations extend the lifetime of columns, offering a better return on investment.
- Temperature Stability: Columns that withstand varying temperatures improve method robustness.
These advancements not only enhance method performance but also support environmentally friendly practices by needing less solvent.
Hybrid Techniques
Hybrid techniques in HPLC integrate various analytical methods to achieve better results and broaden the scope of analyses. For instance, coupling HPLC with Mass Spectrometry (HPLC-MS) provides detailed molecular information, which is invaluable for complex samples.
The synergy between techniques introduces notable benefits:
- Improved Sensitivity: Combined methodologies increase the detection of trace compounds.
- Comprehensive Analysis: Users gain valuable insight into both qualitative and quantitative aspects of samples.
- Versatility: Hybrid methods adapt to a variety of applications, from drug development to environmental monitoring.
As hybridization continues to evolve, researchers will likely uncover new potential applications that further enrich the field of analytical chemistry. This holistic approach is paramount in addressing analytical challenges across various scientific domains.
Ending
The conclusion serves as a critical element in this article, weaving together various insights gathered from the previous sections. HPLC, as a sophisticated analytical technique, leaves a distinct mark on the fields of chemistry, biology, and even environmental science. This technique is not only significant in its application but also provides an essential understanding of underlying principles that govern it.
The importance of conclusively summarizing the content cannot be overstated. It helps the reader regain their focus after exploring detailed methodologies and complex terminologies. Furthermore, it emphasizes the core findings, allowing for reflection on how these can be applied in real-world scenarios.
A well-rounded conclusion also highlights the future relevance of HPLC in ongoing research, promoting continued engagement and exploration in this area.
Summary of Key Insights
In summation, High-Performance Liquid Chromatography stands as a foundational technique in various scientific disciplines. Here are the key points discussed throughout the article:
- Fundamental Principles: The principles of HPLC are centered around the efficient separation of components in a mixture, employing various mechanisms that depend on interactions within the column and the mobile phase.
- Critical Components: Understanding the system's components—such as pumps, injection systems, columns, and detectors—is essential for effective application and troubleshooting of the technique.
- Method Development: The nuances of optimizing methodologies and solvent selection are crucial for achieving reliable results. Validating these methods ensures reproducibility and accuracy in analytical work.
- Wide Applications: HPLC plays a pivotal role in pharmaceutical analysis, food safety, and environmental testing, showcasing its versatility in different fields.
- Interpreting Results: A thorough comprehension of chromatograms and quantification techniques facilitates accurate data interpretation.
Significance of HPLC in Modern Research
High-Performance Liquid Chromatography has emerged as a cornerstone in modern research landscapes. Its significance lies in its ability to provide precise analytical data in a time-efficient manner. Researchers continue to rely on HPLC for its ability to identify and quantify analytes in complex samples with robustness and relevance.
As industries evolve, the demand for more sophisticated analytical techniques grows. HPLC, with its ongoing improvements in technology and method development, remains at the forefront. Its integration with other methods like mass spectrometry enhances its capability, making it indispensable in pharmaceutical and biomedical research.
To conclude, HPLC not only serves current analytical needs but also paves the way for future advancements. Its importance cannot be overlooked, reaffirming its status as an essential technique in scientific inquiry.