Components of Solar Cells: An In-Depth Analysis
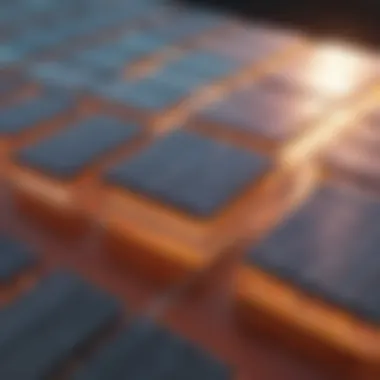
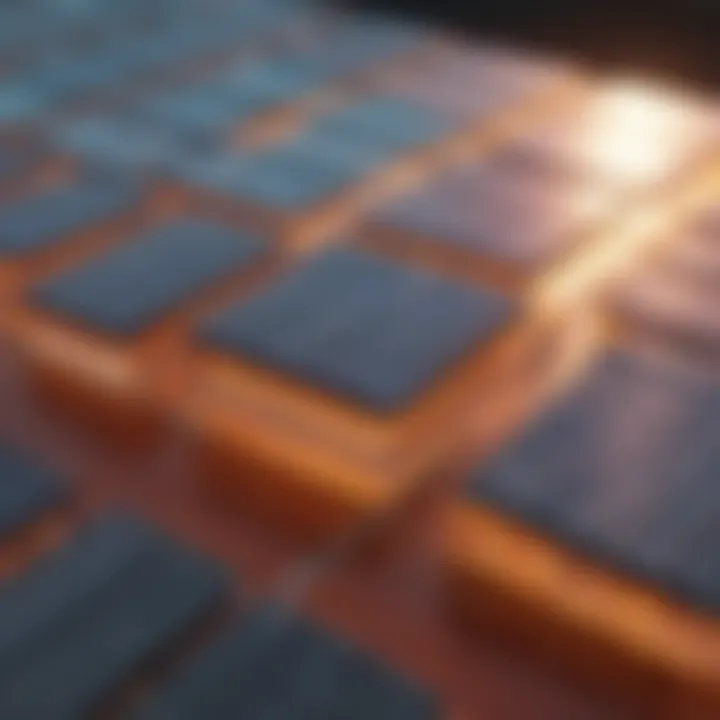
Intro
Solar cells are at the forefront of renewable energy technology. They convert sunlight into electricity, playing a critical role in combating climate change. Understanding solar cells requires delving into their components and how each part works together to enhance performance and efficiency.
Solar cells consist of various materials and structures. Each of these elements has a specific function, contributing to the overall operation of the device. By examining these components, we can appreciate the complexity behind solar technology.
In this analysis, we will explore key components like semiconductor layers, electrical contacts, and protective coatings. This exploration will help clarify their contributions to energy conversion processes. The advancement in materials and technologies will also be discussed, including challenges and future opportunities within the field.
Methodology
Overview of Research Methods Used
The investigation into solar cell components relies on a combination of experimental and theoretical research. Experiments are conducted to test the efficiency of various materials under different conditions. Simulations are also employed to predict how changes in design can affect performance.
Data Collection Techniques
Data is collected through multiple avenues:
- Laboratory experiments provide empirical evidence on efficiency rates.
- Literature review enables a comparison of current findings with existing knowledge.
- Interviews with industry experts offer insights into practical challenges and innovations.
Combining these methods results in a comprehensive understanding of solar cell technology.
Key Components of Solar Cells
Semiconductor Layers
Semiconductors are perhaps the most crucial component of solar cells. Materials like silicon are commonly used because they have the ability to absorb sunlight and generate electric current.
This component's arrangement and thickness directly influence the efficiency of energy conversion. Advances in lab-grown materials, such as perovskites, show promise for higher efficiency rates, but require further study.
Electrical Contacts
Electrical contacts facilitate the extraction of current produced within the semiconductor layers. They connect the semiconductor to the external circuit, enabling energy flow.
Metal contacts used must have low resistance to ensure maximum current extraction. Research into alternative materials, such as silver and aluminum, is ongoing to identify combinations that minimize loss.
Protective Coatings
Protective coatings serve to shield solar cells from environmental factors such as moisture, dust, and UV damage. These coatings are essential for enhancing the longevity of solar panels.
Recent studies focus on developing more durable coatings while maintaining transparency to maximize light absorption.
"The right combination of components is key to improving the performance and durability of solar cells, crucial for their role in sustainable energy."
Future Directions
Upcoming Trends in Research
Research is continuing to evolve. Future studies are likely to focus on new materials that can provide higher efficiency at lower costs. Emerging technologies such as bifacial solar panels and transparent solar cells are gaining attention.
Areas Requiring Further Investigation
Despite advancements, several areas warrant additional exploration:
- Improving energy storage solutions
- Enhancing recycling methods for solar cell materials
- Addressing the scalability of new materials for mass production
A comprehensive approach to these issues may redefine the solar cell industry, making technology more accessible.
Understanding the components that constitute solar cells offers valuable insights into the renewable energy landscape. This knowledge is essential for students, researchers, and professionals alike, providing a foundation for future discoveries and innovations in solar technology.
Intro to Solar Cells
Solar cells are a pivotal technology in the quest for sustainable energy sources. As conventional energy sources deplete, their significance rises. This section lays the groundwork for understanding solar cells by diving into their definition, purpose, and broader implications within renewable energy.
Definition and Purpose
At its core, a solar cell is a device that converts sunlight into electricity through the photovoltaic effect. When light rays hit the cell, they excite electrons, creating a flow of electric current. The primary purpose of solar cells is to harness solar energy—one of the most abundant energy resources available on Earth. Their ability to generate electricity from sunlight provides a clean, renewable alternative to fossil fuels. This technology has applications ranging from residential systems powering homes to large solar farms supplying energy to the grid.
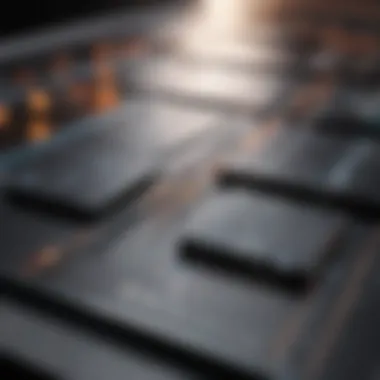
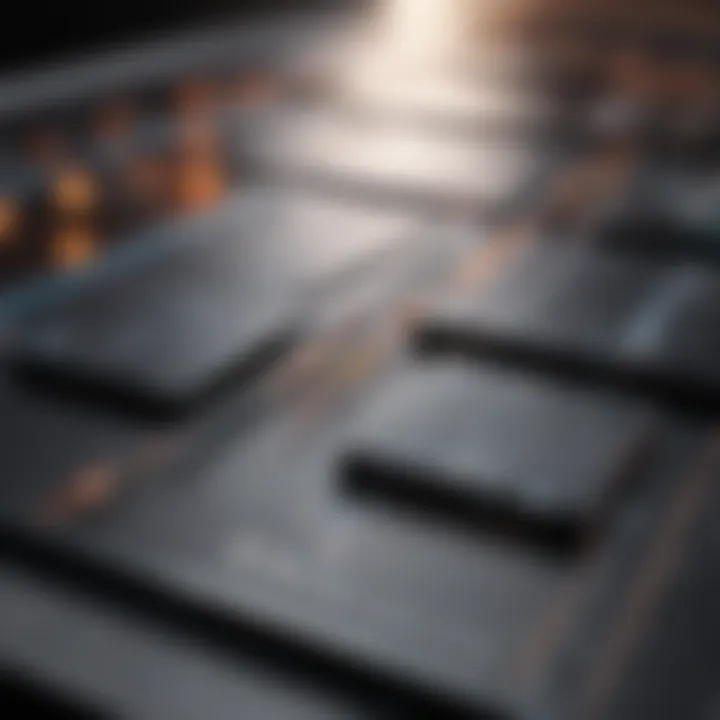
Importance in Renewable Energy
The role of solar cells in renewable energy is vital. As concerns about climate change and energy sustainability increase, solar energy provides a solution. It reduces reliance on fossil fuels, thereby decreasing greenhouse gas emissions. Furthermore, solar installations can be deployed in various settings, from remote areas lacking grid infrastructure to urban environments. This flexibility makes solar energy accessible to a wide audience. In addition, as technology progresses, the efficiency and affordability of solar cells continue to improve, driving further adoption in both developed and developing countries.
"Solar energy is not just the future; it is the present that must be embraced."
As solar technology develops, it highlights the importance of continuous research and development in the field. Solutions to challenges like energy storage and material costs are essential for maximizing the contribution of solar energy to the global energy mix. The ongoing progress in solar cell innovation ensures that this technology remains relevant in achieving global sustainability objectives.
Understanding the fundamentals of solar cells sets the stage for discussing their components, functionality, and future trends. This knowledge not only serves educational purposes but also informs future developments in solar technology.
Fundamental Components of Solar Cells
Understanding the fundamental components of solar cells is essential. These elements are the building blocks that enable solar technology to convert sunlight into usable energy. Each component plays a unique role that affects the overall performance, efficiency, and cost-effectiveness of solar cells. The choice of materials and their arrangement directly influences how well solar panels operate in different conditions.
Photovoltaic Effect
The photovoltaic effect is the foundation of solar energy conversion. It describes how sunlight, when absorbed by a material, generates an electric current. When photons from sunlight collide with semiconductors in the solar cell, energy is transferred to electrons. This energy causes the electrons to move and create an electric flow through the material. This principle is crucial for the operation of any photovoltaic system. Understanding this effect helps in innovating ways to enhance efficiency and improve solar cell design.
Semiconductors
Semiconductors are the heart of solar cells. They are materials that can conduct electricity better than insulators but not as efficiently as conductors. The performance of a solar cell heavily depends on the type of semiconductor used.
Silicon
Silicon is the most common semiconductor used in solar cells. Its key characteristic is its abundance and relatively low cost. Silicon is a widely studied material, and its handling is well understood. A unique feature of silicon is its capability to be easily manipulated to improve efficiency. The advantages of silicon include good efficiency levels and a stable performance over time. However, it can be less effective in low-light conditions compared to other semiconductors.
Cadmium Telluride
Cadmium telluride is another semiconductor used in thin-film solar cells. Its key characteristic is the ability to be deposited in a thin layer, making it cost-effective and lightweight. Cadmium telluride has a higher absorption coefficient, which allows it to absorb sunlight more efficiently even with less material. This unique feature can lead to more flexible solar panel designs. However, it poses environmental concerns due to the toxicity of cadmium.
Gallium Arsenide
Gallium arsenide is known for its high efficiency in converting sunlight to electricity. Its key characteristic is exceptional performance even under high temperatures and low sunlight conditions. Gallium arsenide's unique feature is its capability to be used in multi-junction solar cells, which can capture a broader spectrum of sunlight. The disadvantage lies in its high production cost, which limits its widespread adoption.
Conductive Contacts
Conductive contacts are essential in solar cells, as they allow for the collection and transfer of the electric current generated by the photovoltaic effect. These contacts directly impact the efficiency and performance of a solar cell.
Front Contact
The front contact is responsible for collecting the generated electrical current. Its key characteristic is that it must be transparent to allow sunlight to pass through while still conductive. Additionally, a unique feature of the front contact is that it is often made with fine metal lines to minimize shading on the solar cell surface. This design allows for better efficiency but can pose challenges in maintaining balance between conductivity and transparency.
Back Contact
The back contact serves as the layer where electric current exits the solar cell. Its key characteristic is that it generally consists of a reflective material to maximize light absorption. The unique feature of the back contact is its role in ensuring that electrons flow efficiently out of the cell. However, improper design can lead to resistive losses, affecting the overall efficiency of the solar cell.
Layer Structure of Solar Cells
The layer structure of solar cells is a critical aspect that directly influences their performance and efficiency. Each layer serves a specific purpose, contributing to the overall function of the solar cell in converting sunlight into electricity. The arrangement and materials chosen for these layers affect how well a solar cell can absorb light, convert that light into electrical energy, and manage the flow of that energy. Understanding each layer helps to assess its efficiency and potential in various applications.
Top Layer (Anti-reflective Coating)
The top layer of a solar cell, usually an anti-reflective coating, plays an essential role in enhancing light absorption. This layer reduces reflection off the surface of the solar cell, allowing more sunlight to enter. The commonly used materials for this coating often include silicon nitride or titanium dioxide. By minimizing reflectivity, the anti-reflective coating increases the amount of light that can reach the active layer below. As a result, this layer significantly boosts the overall efficiency of solar cells.
Active Layer
The active layer is where the actual photovoltaic effect occurs, generating charge carriers that ultimately create electricity. This layer typically consists of a semiconductor material, and its structure is crucial for the solar cell's performance.
p-n Junction
A key feature of the active layer is the p-n junction. This structure is formed by joining p-type and n-type semiconductors, creating an electric field. The presence of this field is fundamental to the solar cell’s operation. It facilitates the separation of charge carriers—electrons and holes—generated when sunlight is absorbed. The p-n junction is a popular choice due to its effective charge separation capabilities, which in turn enhances the electrical output of the solar cell. The unique feature of the p-n junction is its ability to maintain an electric field without external power. This efficiency makes it an attractive choice in solar technology. However, it can have disadvantages, such as susceptibility to certain types of degradation under continuous exposure to sunlight.
Charge Carrier Mobilization
Charge carrier mobilization is pivotal in determining how well the generated charge carriers can move through the semiconductor material to the conducting contacts. High mobility of these carriers is crucial for optimizing energy conversion efficiency. The materials used in the active layer directly impact this mobilization. For instance, silicon generally offers a good balance between cost and efficiency. However, emerging materials like perovskites are showing promising characteristics in charge carrier dynamics. A potential downside of these materials could be stability issues, which require further research. Therefore, understanding charge carrier mobilization contributes significantly to improving solar cell technologies.
Back Layer
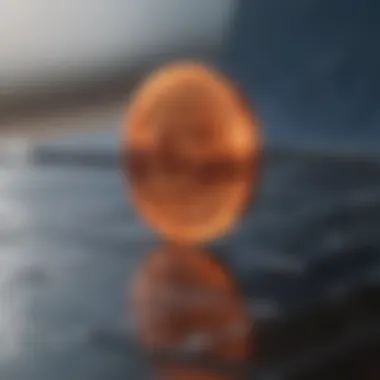
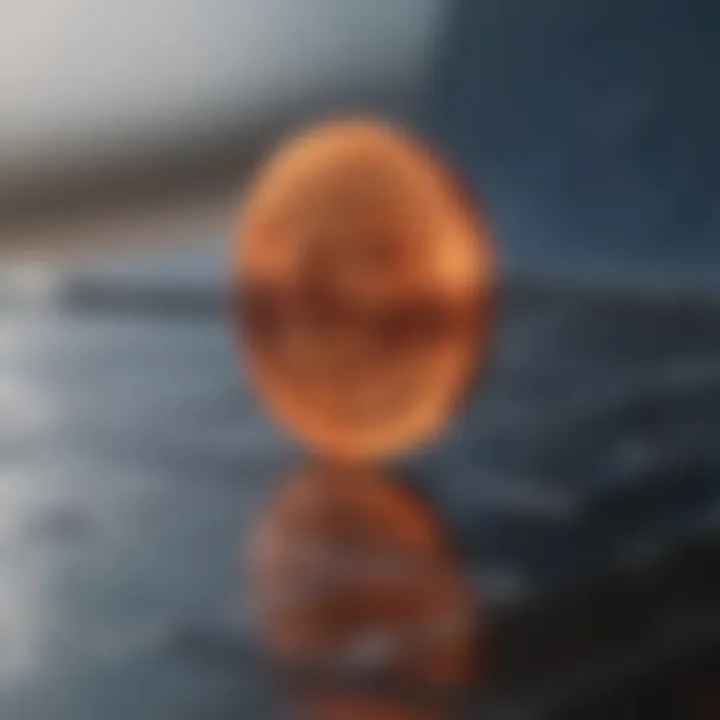
The back layer of a solar cell serves multiple purposes, primarily acting as a reflector for unused light and providing physical support to the structure. While this layer may not directly contribute to the photovoltaic effect, it is essential for overall energy efficiency. Typically, materials such as aluminum are used to reflect light back into the active layer for a second chance at absorption. Additionally, a robust back layer can enhance the mechanical stability of the solar cell, extending its lifespan.
In summary, the layer structure of solar cells plays a vital role in maximizing efficiency and performance. Each layer functions interdependently, contributing to the conversion of solar energy into usable electrical power.
Performance Metrics of Solar Cells
Understanding the performance metrics of solar cells is crucial as it allows us to assess and compare their effectiveness. Metrics such as efficiency, fill factor, and temperature coefficient inform researchers and manufacturers about how well solar cells convert sunlight into energy. Adopting the right performance metrics can lead to advancements in solar technologies and encourage investment in renewable energy solutions. The metrics not only help in evaluating the current technologies but also guide future research and product development.
Efficiency
The efficiency of solar cells signifies the percentage of sunlight converted into usable electrical energy. For instance, a solar cell with an efficiency of 20% transforms one-fifth of the sunlight it receives into energy. This metric is a top priority for researchers and manufacturers as it directly impacts the power output of solar panels.
Performance is influenced by several factors including:
- Quality of materials: Higher-quality semiconductors lead to better efficiency.
- Design of the cell: Innovations in photovoltaic materials and structures can enhance efficiency.
- Environmental conditions: Factors such as temperature and light intensity play a role in real-world performance.
Overall, efficiency is a vital metric as it determines how much energy can be harvested from a given area, making it crucial for applications where space is limited.
Fill Factor
The fill factor (FF) indicates the quality of the solar cell and is calculated by the ratio of the maximum power output to the product of open-circuit voltage and short-circuit current. A fill factor close to 1 (or 100%) signifies a high-quality solar cell with minimal resistive losses. The formula is:
Where:
- Pmax: Maximum power output
- Voc: Open-circuit voltage
- Isc: Short-circuit current
The fill factor is influenced by:
- Material properties: The type of semiconductor affects internal losses.
- Manufacturing processes: Careful fabrication can enhance performance.
A higher fill factor indicates better cell performance, rendering it a metric of interest in both development and evaluation of solar technologies.
Temperature Coefficient
The temperature coefficient measures the effect of temperature on the performance of solar cells. Typically expressed as a percentage per degree Celsius, this metric indicates how much efficiency decreases as temperature rises. Most solar cells experience a decrease in efficiency with increasing temperature.
Understanding the temperature coefficient is essential for several reasons:
- Performance prediction: Knowing how cells perform under varying environmental conditions helps in designing systems for maximum yield.
- Long-term implications: High temperature coefficients may indicate a decrease in energy output over time.
- System design: Engineers can design solar installations that mitigate excessive heat.
Thus, the temperature coefficient helps predict the onset of thermal losses in operating conditions, indicating an area of performance to optimize.
"Efficiency, fill factor, and temperature coefficient are the backbone metrics guiding solar technology research and investments."
Current Trends in Solar Cell Technology
The field of solar cell technology is evolving rapidly, influenced by both scientific advancements and market demands. Understanding these trends is essential for those engaged in research and development, as well as for consumers interested in sustainable energy solutions. This section will explore significant current trends in solar cells, focusing on emerging materials and bifacial solar cells. These innovations promise to enhance efficiency, reduce costs, and broaden applications of solar technology.
Emerging Materials
Perovskite Solar Cells
Perovskite solar cells are a notable trend. Their structure, typically composed of a unique crystal arrangement, allows solar energy conversion at a low cost. The key characteristic of perovskite solar cells is their high efficiency, which has been rapidly increasing over the years. Some studies report efficiencies exceeding 25%. This is particularly significant because it offers a competitive alternative to traditional silicon-based cells.
One unique feature of perovskites is their variable bandgap, which makes them tunable for specific light absorption spectra. This adaptability can lead to better sunlight harvesting, especially in diverse environments. While perovskite solar cells show great promise, they also face challenges such as stability and scalability in production, which need addressing for wider adoption.
Organic Photovoltaics
Organic photovoltaics (OPVs) are another exciting area in solar technology. These solar cells use organic materials to convert sunlight into electricity. The main advantage of OPVs is their lightweight and flexibility, making them suitable for a variety of applications, including integration into building materials or mobile devices.
The distinctive feature of organic photovoltaics is their solution-based manufacturing process, which can be less energy-intensive compared to traditional methods. However, there are limitations, such as lower efficiencies compared to silicon cells. Despite this, ongoing research aims to improve performance and lifespan, making organic photovoltaics an attractive option in the solar landscape.
Bifacial Solar Cells
Bifacial solar cells represent a significant advancement in solar technology. These cells are designed to capture sunlight from both sides, which can increase the overall energy yield. The effectiveness of bifacial cells relies on several factors, including the installation environment and the reflective properties of surfaces below the solar panel.
The benefit of bifacial solar cells is seen in their ability to utilize diffused sunlight and reflected light, enhancing efficiency without requiring additional direct sunlight. This makes them particularly suitable for ground-mounted systems where the ground surface can improve the solar array's performance.
As the demand for more efficient and sustainable solar technologies grows, the trends highlighted here illustrate the direction of innovation. The focus on materials like perovskites and organics, along with advancements in bifacial designs, underscores the commitment to improving the efficiency and applicability of solar energy. This continuous evolution is key for addressing global energy challenges and advancing towards sustainable practices.
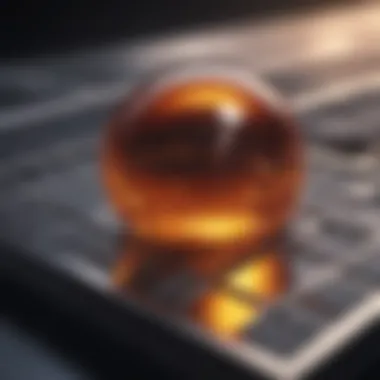
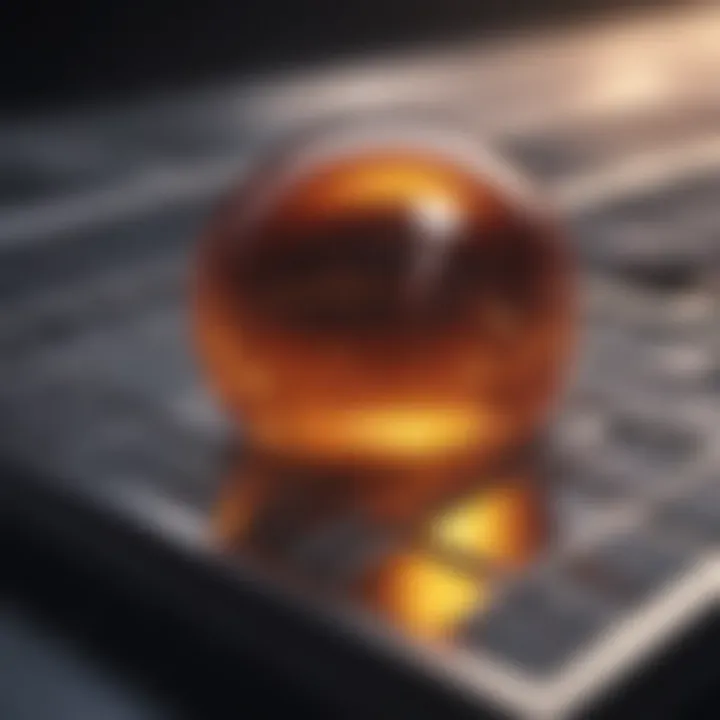
Challenges in Solar Cell Development
The development of solar cells faces multiple challenges that can hinder progress in achieving widespread implementation and efficiency. Addressing these challenges is crucial for the advancement of solar technology. The focus lies in understanding the specific obstacles related to material costs, energy conversion efficiency, and the environmental impact of manufacturing. By examining these elements, we can grasp how they influence not only the technology itself but also its adoption on a global scale.
Material Costs
Material costs significantly impact the manufacturing of solar cells. The price of key materials, especially semiconductors like silicon, affects the overall affordability of solar technologies. For instance, the fluctuation in silicon prices can lead to increased production costs, which in turn reflects on the final pricing of solar panels.
- Silicon is the most widely used material in solar cells, but there are alternatives such as cadmium telluride and gallium arsenide. Both required more specific fabrication techniques, often leading to higher expenses.
- While emerging technologies like perovskite cells promise reduced costs, their production processes are not yet fully optimized for commercial applications.
The goal is to find cost-effective materials without sacrificing efficiency. Research in this area is vital, as lower costs enable more people to access renewable energy technologies, enhancing solar energy's role in sustainable development.
Energy Conversion Efficiency Barriers
Energy conversion efficiency is a major focus in solar technology. High efficiency means that solar cells convert more sunlight into usable electricity, which is essential for maximizing energy output. However, achieving high efficiency presents various barriers:
- The theoretical efficiency limits, defined by concepts like the Shockley-Queisser limit, set boundaries on the maximum efficiency achievable by traditional silicon cells.
- There are losses during energy conversion processes, including thermal losses and recombination of charge carriers, that further reduce efficiency. Developing new materials and technologies that minimize these losses is critical.
Research continues in this domain, with innovations in multi-junction solar cells and techniques to enhance charge carrier mobility aiming to push efficiency boundaries. However, these innovations need to be cost-effective for widespread deployment, creating a complex balancing act.
Environmental Impact of Manufacturing
The environmental impact of solar cell manufacturing is another crucial challenge. While solar energy systems produce clean energy during their lifespan, the production process can have considerable environmental ramifications:
- The extraction of raw materials can lead to habitat destruction and pollution. For example, mining for silicon can have significant ecological consequences.
- Chemical processes used in manufacturing can also create hazardous waste materials. Proper management and recycling of these materials are necessary to mitigate environmental risks.
- Additionally, the energy used in manufacturing solar panels must be considered, as it can sometimes negate some benefits of the solar energy produced.
To address these challenges, manufacturers are exploring cleaner production methods and recycling programs for end-of-life solar panels. Striving for sustainable practices in production will enhance the overall appeal of solar technology as a green energy solution.
"Understanding and solving the challenges in solar cell development is critical for the transition to sustainable energy systems."
These challenges are interconnected and require concerted efforts from researchers, manufacturers, and policymakers. By overcoming material costs, energy conversion efficiency issues, and environmental concerns, the potential for solar technology can be fully realized.
Future Perspectives for Solar Technology
The future of solar technology presents a multitude of possibilities that can transform energy production and consumption. As we continue to face the impacts of climate change and the need for renewable energy sources, advancements in solar technology become increasingly essential. Here, we will examine two crucial aspects: integration with smart grids and advancements in energy storage solutions. These elements hold significant potential to optimize solar energy utilization, enhance efficiency, and promote sustainability.
Integration with Smart Grids
Smart grids represent a modernized electrical grid that utilizes digital communication technology to enhance the reliability and efficiency of electricity delivery. The integration of solar technology into smart grids can empower both individuals and communities. This integration helps in several ways:
- Real-time monitoring: It allows for the observation of solar energy production and consumption patterns, leading to optimized energy management.
- Dynamic pricing: Smart grids can enable consumers to take advantage of lower energy rates when solar generation is high, thus reducing energy costs.
- Distributed energy resources: They support a decentralized system that allows for contributions from various solar installations, thereby enhancing grid resilience.
The combination of solar energy systems with smart grids can facilitate seamless energy distribution and consumption. In addition to this, enhanced communication between solar installations and the grid can lead to better forecasting, ultimately leading to improved energy reliability.
Advancements in Energy Storage Solutions
Energy storage solutions are pivotal in maximizing the benefits of solar technology, particularly given the intermittent nature of sunlight. As advancements are made in energy storage, the potential for widespread solar adoption increases. Key developments include:
- Battery Technologies: Lithium-ion batteries have gained prominence due to their efficiency and decreasing costs. Other storage options, such as flow batteries and solid-state batteries, are also being explored for their potential long-term benefits.
- Grid-scale storage: Large-scale batteries can store excess energy generated during peak sunlight hours for use during non-production periods, enhancing the stability of energy supply.
- Decentralized storage: Home-based solar energy storage systems allow households to utilize their energy production more effectively and reduce reliance on the grid.
"Advancements in energy storage are essential for overcoming the challenges of solar energy production variability."
The fusion of improved storage technologies with solar energy can provide a more reliable power supply, making solar a more viable option for households and industries alike. Moreover, these improvements can also drive down costs associated with energy storage, making them a more attractive investment for consumers and businesses.
Ending
The conclusion serves a vital role in summarizing the principal insights discussed throughout the article. It encapsulates the essence of solar cell components, emphasizing their importance in the overall efficiency and functionality of solar technology. By dissecting various elements like semiconductors, conductive contacts, and protective coatings, this article sheds light on not just the operational principles, but also the advancements and challenges that influence solar energy production.
Summary of Key Points
In this article, several key points were highlighted:
- Definition of Solar Cells: An understanding of what solar cells are and their function within the renewable energy sector.
- Components Detailed: A thorough analysis of essential components, including the photovoltaic effect, semiconductors like silicon and gallium arsenide, and various layered structures.
- Performance Metrics: Evaluation of efficiency, fill factor, and the impact of temperature on performance.
- Current Trends: Exploration of emerging materials such as perovskites, along with innovations like bifacial solar cells.
- Challenges Addressed: Delving into issues like material costs and environmental impact, which continue to pose challenges for the industry.
- Future Outlook: Discussion of how integration with smart grids and advancements in energy storage can usher solar technology into a new era.
Call to Action for Research and Development
Encouraging continuous research in solar technologies is crucial. The need to innovate is paramount, particularly with regard to:
- Developing more affordable and sustainable materials without compromising efficiency.
- Enhancing the energy conversion efficiency of existing solar cells.
- Addressing the environmental concerns associated with production and disposal.
Furthermore, collaboration among researchers, engineers, and policymakers can foster a conducive environment for these advancements. Increased funding and support for cutting-edge research initiatives will pave the way for breakthroughs that can revolutionize the solar energy landscape.
By targeting these areas, the solar cell industry can not only overcome present barriers but also significantly contribute to a sustainable future.