Bacterial RNA Sequencing: Methodologies and Insights
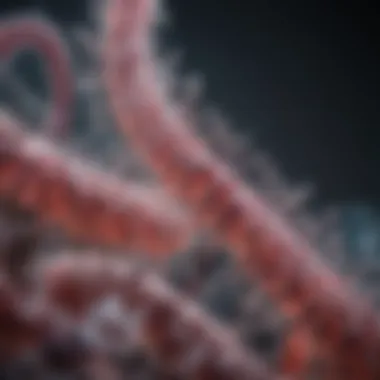
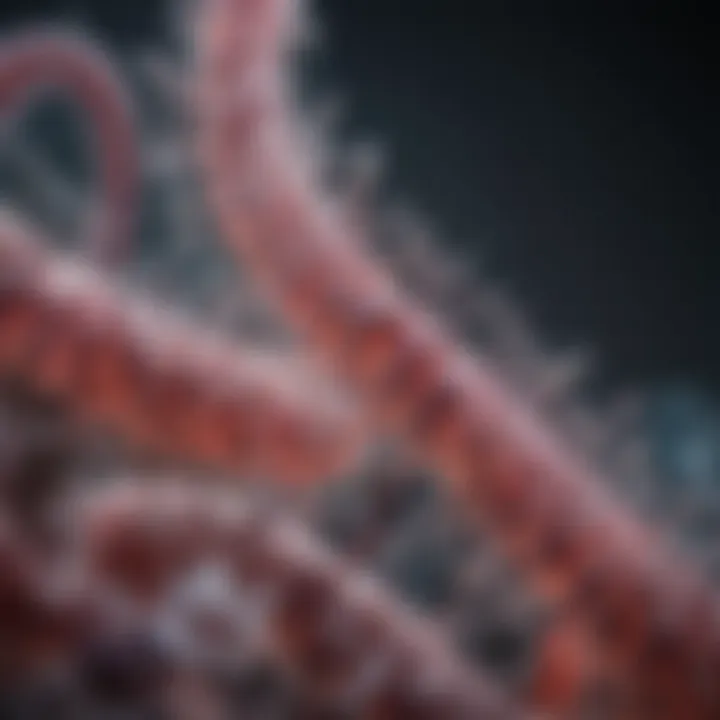
Intro
Bacterial RNA sequencing has become a cornerstone in the realm of microbiology, propelling our understanding of gene expression and the inner workings of microbial ecosystems. With the rapid evolution of technology and methodologies, researchers can now delve deeper into the intricacies of bacterial life. This journey through RNA sequencing reveals how these small entities play substantial roles in various ecological niches, from soil to the human gut.
The significance of bacterial RNA sequencing lies in its ability to provide a real-time snapshot of microbial activity. Traditional methods often fell short in vividly illustrating the dynamic nature of bacterial populations. However, the advent of RNA sequencing fills this gap, allowing us to explore not only what genes are present but also which are actively expressed at any given moment. This vital aspect opens doors to various applicationsβfrom environmental monitoring to disease diagnosis.
As we embark on this comprehensive examination, it's essential to highlight the methodologies used in bacterial RNA sequencing, the obstacles encountered, and the implications these studies hold for future research. The following sections will guide the reader through the multifaceted world of bacterial RNA sequencing, emphasizing the critical role it plays in deciphering complex biological interactions.
Prolusion to Bacterial RNA Sequencing
Bacterial RNA sequencing emerges as a pivotal tool in the modern scientific toolkit, shedding light on the intricate world of microbes. This approach goes beyond simple gene identification, diving into the dynamic landscape of gene expression, regulatory mechanisms, and their responses to environmental stimuli. By decoding the various RNA molecules present in bacteria, researchers gain insights that are not only fundamental for microbiology but also for clinical applications and biotechnological innovations.
The importance of this topic is manifold. At its core, bacterial RNA sequencing helps provide clarity about how bacteria operate, what signals they respond to, and how they evolve. Understanding these aspects can aid in addressing pressing challenges such as antibiotic resistance and microbial pathogenicity. Furthermore, the data gathered can pave ways to enhance microbial applications in areas like agriculture or bio-remediation.
The ability to analyze RNA on such a large scale provides numerous benefits, including but not limited to:
- Greater accuracy in identifying gene expression levels.
- Insight into the roles of non-coding RNA, which are often overlooked yet critical in regulation.
- The potential to discover novel bacterial species or strains that were previously unrecognized.
However, certain considerations must be taken into account. The complexity and variability of bacterial populations can present challenges in obtaining reliable data. Factors such as growth conditions, sample processing, and sequencing technology can influence results in significant ways.
"In the world of microbial research, understanding RNA offers a window into cellular processes that remain largely shrouded in mystery."
This introductory section establishes the foundation for understanding the core principles and methodologies surrounding bacterial RNA sequencing and highlights its invaluable contribution to advancing our comprehension of microbial life.
Definition and Importance
Bacterial RNA sequencing refers to the high-throughput sequencing of RNA from bacterial cells to identify and quantify gene expression. The process allows scientists to capture a snapshot of the active genes at any given time, providing a window into the organism's function under specific conditions. The importance of this technique lies in its ability to illuminate the relationships between the genetic blueprint of bacteria and their phenotypic behaviors.
In practical applications, this means unlocking the potential for advancements in several fields, including:
- Studying bacterial responses to antibiotics, which can guide treatment strategies.
- Gaining insights into beneficial microbial communities for applications in agriculture and health.
- Understanding mechanisms of pathogenicity, which can drive new vaccine developments.
Historical Context
The historical backdrop of bacterial RNA sequencing is essential to appreciate its evolution and significance today. Initially, the study of bacterial RNA was limited to tedious methods that did not provide a comprehensive view of bacterial gene expression. The real game changer came with the advent of sequencing technologies in the mid-2000s, notably with the development of next-generation sequencing (NGS) platforms.
Before these advancements, researchers relied heavily on methods like Northern blotting and reverse transcription followed by quantitative PCR, which were either low-throughput or lacked the depth of analysis necessary for comprehensive studies. The shift to NGS not only democratized access to sophisticated sequencing capabilities but also drastically reduced costs and timelines, ushering in an era of rapid discoveries in microbial biology.
Now, as we navigate this landscape, understanding the historical context of bacterial RNA sequencing serves to highlight both the challenges faced and the remarkable progress made in the field. Embracing this knowledge allows researchers to build on past experiences, fine-tuning methodologies for future explorations.
Core Principles of RNA Sequencing
Understanding the core principles of RNA sequencing is fundamental for grasping the evolving landscape of bacterial genomics. These principles lay the groundwork for how researchers obtain, process, and interpret RNA data from bacteria. Recognizing the mechanisms and types of RNA sequencing not only enriches our comprehension of microbial behavior but also opens new avenues for advancements in medical and environmental microbiology.
Basic Mechanisms of RNA Sequencing
At its core, RNA sequencing is a powerful tool that uses high-throughput sequencing technologies to reveal the presence and quantity of RNA in a biological sample. This process often involves three crucial steps: extraction of RNA from cells, conversion of RNA to complementary DNA (cDNA) through reverse transcription, and then sequencing the cDNA.
Each step demands precision. Extracting RNA focuses on preserving the integrity of RNA molecules, as they can be fragile and prone to degradation. After extraction, the transformation to cDNA is pivotal because it stabilizes the sequence and prepares for the next generation of sequencing techniques. Sequencing itself, commonly executed via platforms like Illumina, produces vast amounts of data that reflect the transcriptome of bacterial species under study.
The result is a comprehensive view of gene expression patterns, which can vary significantly based on environmental factors, stress responses, or developmental stages. Such insights are gaining significant traction in microbial ecology and pathogenesis studies.
Types of RNA Sequencing
There are diverse types of RNA sequencing techniques, each addressing different layers of gene expression and regulation. Understanding these can help researchers determine which approach best suits their specific objectives and provide richer data. Here are the main types:
mRNA Sequencing
mRNA sequencing, usually referred to as mRNA-seq, is perhaps the most commonly utilized method in RNA sequencing. This technique focuses primarily on capturing the messenger RNA, which is the transcript that conveys genetic information from DNA to the ribosome, where proteins are synthesized. One compelling aspect of mRNA sequencing is its ability to analyze not only the quantity of mRNA but also its structure and splicing variants.
The main advantage of mRNA-seq lies in its ability to provide a detailed landscape of active gene expression in cells. Researchers harness this method to identify genes that are upregulated or downregulated under specific conditions, such as during bacterial infection or in response to antibiotics. However, one limitation to consider is that mRNA-seq may miss non-coding RNAs and other vital transcriptional elements, potentially skewing the interpretation of a bacteriumβs overall transcriptomic profile.
Small RNA Sequencing
Small RNA sequencing hones in on the smaller RNA molecules, typically less than 200 nucleotides long, that play critical roles in gene regulation and cellular functions. These include microRNAs, small interfering RNAs, and other regulatory entities. The beauty of this technique is its focus on the subtler, yet pivotal, layers of gene regulation that traditional mRNA sequencing might overlook.
Small RNA sequencing has grown in popularity because it offers insights into the post-transcriptional regulation of gene expression, revealing how bacteria adapt to environmental stresses or regulatory signals. However, one must tread carefully, as the preparation and sequencing libraries can sometimes yield low throughput or limited coverage for certain RNA types, potentially impacting comprehensive analysis.
Total RNA Sequencing
Total RNA sequencing, on the other hand, captures the full spectrum of RNA in a cell, including coding and non-coding regions. This method has the distinct advantage of offering a holistic view of the transcriptome. By preserving all RNA types during the analysis, researchers can compare the full range of expressions and discover interactions that might otherwise go unnoticed.
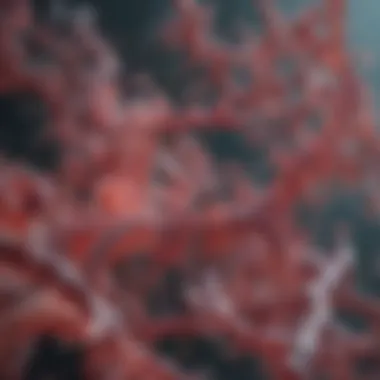
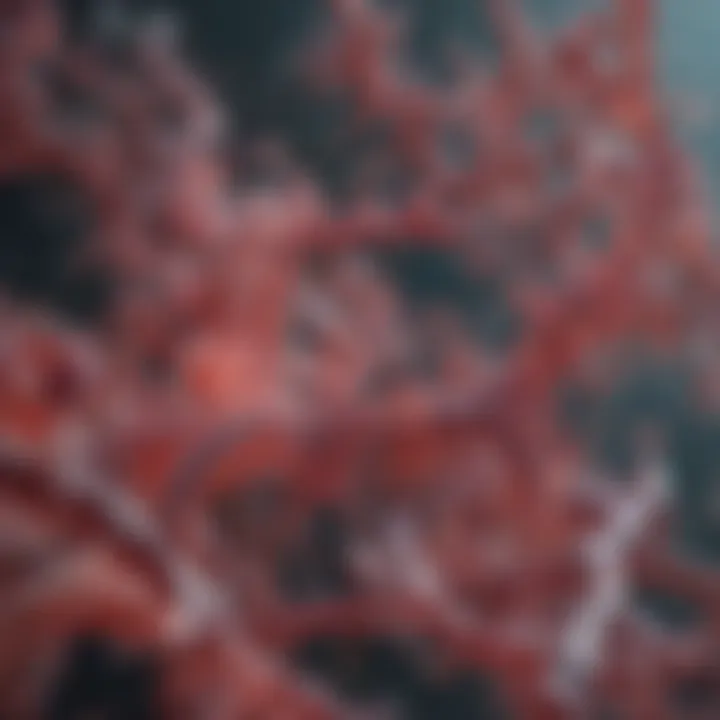
The ability to analyze everything from mRNA to ribosomal and transfer RNA, as well as non-coding RNAs, makes total RNA-seq a powerful choice for comprehensive bacterial transcriptome characterization. However, this inclusive technique is not without its challenges; the complexity of the data can lead to overwhelming volumes of sequencing data, necessitating robust bioinformatics tools for effective analysis.
Total RNA sequencing provides a complete snapshot of the RNA landscape, crucial for understanding complex regulatory networks in bacteria.
Each RNA sequencing type offers unique insights and poses its own set of challenges. Scientists must consider their specific goals, the nature of their samples, and the expected outcomes when selecting the appropriate method for their research.
Methodologies in Bacterial RNA Sequencing
In the realm of bacterial RNA sequencing, methodologies serve as the backbone for accurate and effective results. The choice of method can significantly affect the quality of data obtained, leading to profound implications in fields such as microbial ecology, disease research, and biotechnology. By understanding various methodologies, particularly in RNA extraction, library preparation, and sequencing platforms, researchers can optimize their efforts to unravel the complex layers of bacterial gene expression and regulation.
RNA Extraction Techniques
The extraction of RNA is a pivotal step in bacterial RNA sequencing. It directly impacts the quality and quantity of the material available for downstream applications. There are three primary approaches to RNA extraction, each with its own nuances and advantages.
Physical Methods
Physical methods of RNA extraction typically involve mechanical disruption to break down cellular structures and release RNA. This can include techniques such as bead beating or grinding. One key attribute of physical methods is their ability to minimize chemical interference, which can sometimes pose challenges in the purity of RNA.
However, while these methods can yield high-quality RNA, they tend to be resource-intensive and may not be feasible for all laboratory setups. Also, the efficacy can vary significantly depending on the bacterial species, affecting reproducibility across different research scenarios. In summary, physical methods are a viable option but may require considerable investment in equipment and optimization for specific bacterial strains.
Chemical Methods
Chemical methods leverage specific reagents to lyse cells and release RNA. Commonly used agents include phenol-chloroform or guanidine thiocyanate. These methods are advantageous due to their capacity for batch-processing samples and their relative speed. Furthermore, they can efficiently extract RNA from tough bacterial membranes, a characteristic that is particularly beneficial.
Nevertheless, the downside is their dependence on hazardous chemicals, which pose safety concerns and may require careful handling. Additionally, achieving complete removal of the chemicals can sometimes be a challenge, which might affect the subsequent sequencing results. Thus, while chemical methods excel in efficiency, they call for stringent safety protocols and cleanup procedures.
Enzymatic Methods
Enzymatic methods utilize various enzymes, such as lysozyme or proteinase K, to facilitate the breakdown of cell wall structures, allowing RNA to be released. These techniques are often seen as a middle ground between physical and chemical methods. One remarkable feature of enzymatic methods is the selectivity they provide, allowing researchers to target specific cellular components. This can lead to enhanced purity levels compared to other methods.
On the flip side, enzymatic reactions require precise conditions and timing, which can introduce variability if not monitored carefully. Moreover, some bacterial species may not be as responsive to enzymatic treatment, limiting the applicability of this approach in diverse research scenarios. Hence, enzymatic methods can be a powerful tool in the RNA extraction toolkit, though they may necessitate fine-tuned protocols to maximize efficiency.
Library Preparation Protocols
Once RNA is successfully extracted, the next crucial step is library preparation. This involves converting RNA into a form suitable for sequencing, typically by creating complementary DNA (cDNA). Proper library preparation is critical as it directly affects sequencing depth, accuracy, and overall data quality. Different protocols exist, often tailored to specific sequencing technology but generally follow three main stages: cDNA synthesis, amplification, and indexing.
Sequencing Platforms
The choice of sequencing platform essentially dictates the entire workflow of RNA sequencing in bacteria. Here, we delve into three prominent sequencing technologies, each contributing its unique attributes to the overall landscape of bacterial RNA sequencing efforts.
Illumina Technologies
Illumina technologies dominate the RNA sequencing arena, primarily owing to their high throughput and cost-effectiveness. This platform utilizes a sequencing by synthesis approach, which allows for rapid generation of large volumes of data. A critical characteristic of Illumina is its unmatched accuracy, ensuring that the sequencing reads can reliably reflect the underlying RNA populations.
Nonetheless, the trade-offs include challenges with sequencing reads of longer lengths and difficulties in de novo assemblies. Moreover, the initial setup costs and reagents can be significant, creating a barrier for smaller laboratories.
Pacific Biosciences
Pacific Biosciences offers a different strategy via single-molecule real-time (SMRT) sequencing technology. This method excels in generating longer reads, which can unravel complex bacterial transcriptomes with higher resolution. A notable aspect of Pacific Biosciences is its ability to provide insights into isoforms and structural variants due to the longer sequence reads.
However, this technology's costs are generally higher, making it less accessible for some research teams. Plus, while it delivers valuable data, the throughput is lower compared to Illumina, which can be limiting for large-scale studies. Therefore, the choice of using Pacific Biosciences often pertains to specific experimental needs rather than broad applicability.
Oxford Nanopore Technologies
Oxford Nanopore Technologies brings a distinct and innovative edge with its portable sequencing devices that allow for real-time sequencing. This flexibility enables fieldwork and on-site analyses, making it a benedict choice for researchers looking to dynamically assess bacterial populations in their natural habitats.
A unique feature of Oxford Nanopore is its capability to sequence native RNA molecules, preserving modifications and allowing better insights into post-transcriptional modifications. Nevertheless, the accuracy of reads is sometimes a concern, and data may require additional processing. Moreover, while these devices are portable and user-friendly, they typically demand a significant investment in both time and resources to establish a dependable workflow.
Each of these methodologies and platforms contributes to the broader understanding of bacterial RNA sequencing, offering distinct advantages depending on the research questions posed. The interplay of these techniques ensures that researchers can tailor their approaches, optimizing outcomes and addressing the multifaceted nature of microbial transcriptomics.
Data Analysis in RNA Sequencing
When diving into the world of bacterial RNA sequencing, the spotlight on data analysis cannot be overstated. Data analysis is the backbone that validates and synthesizes the vast amounts of information generated through sequencing technologies. Without robust analysis, the raw data remains an unhelpful pile of information, clouding the clarity of biological insights.
The significance of data analysis lies in its ability to distill complex datasets into understandable and actionable intelligence. It helps in recognizing patterns that might otherwise slip under the radar. This capacity for simplification and clarity is crucial, particularly when one considers the burgeoning data volume brought forth by modern sequencing methods. Thus, this section elucidates the tools and methodologies pertinent to data analysis, providing crucial insights for researchers and practitioners alike.
Bioinformatics Tools
Data analysis in RNA sequencing is heavily reliant on bioinformatics tools, which serve as the link between raw data and meaningful interpretations. Here's a closer look at the particular tools vital to this process.
Alignment and Assembly Tools
Alignment and assembly tools are pivotal in the entire RNA sequencing data analysis journey. These tools work to compare sequenced reads to a reference genome, ensuring that the data collected accurately reflects the biological realities being studied. A commonly utilized tool in this category is STAR (Spliced Transcripts Alignment to a Reference), known for its speed and accuracy when handling large datasets.
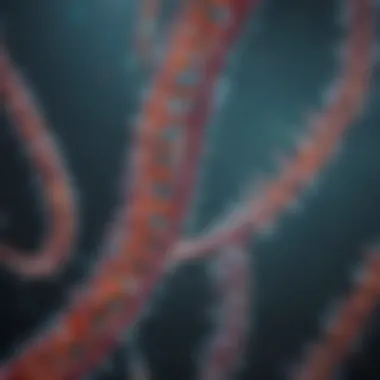
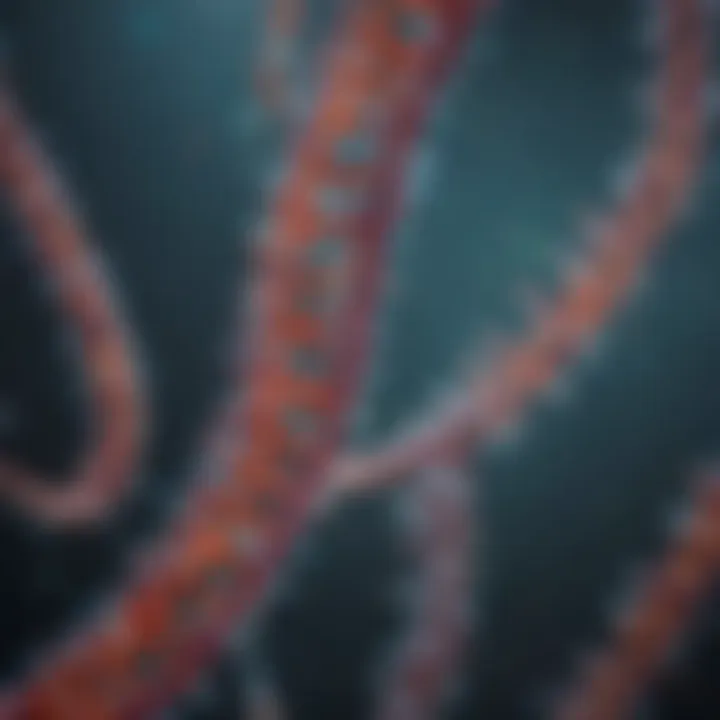
The key characteristic of alignment and assembly tools is their ability to handle vast volumes of data with precision. Their contribution to the overall goal of RNA sequencing analysis is indispensable, as they lay the groundwork for downstream analyses. However, these tools have unique features, such as being able to process both unpaired and paired-end reads, allowing for greater flexibility based on experimental design.
Nevertheless, their disadvantages include the necessity for extensive computational resources and time commitments, especially with particularly large datasets.
Quantification Software
Moving beyond alignment, quantification software serves a different yet similarly critical purpose in RNA sequencing data analysis. These specialized tools calculate the abundance of RNA transcripts, paving the way for gene expression studies. Some noteworthy examples include DESeq2 and edgeR, both of which are commonly used for differential expression analysis.
What sets quantification software apart is its sophisticated statistical models that account for various biases and variations in data, ensuring reliability in results. This characteristic makes it a go-to choice for researchers looking to extract meaningful insights from their sequencing data. A unique feature of such software is its capability to manage complex experimental designs, which is particularly advantageous as research projects often grow in scope.
However, as advanced as these tools are, they do come with limitations. The learning curve associated with these software applications can be steep, posing challenges for those new to bioinformatics.
Statistical Analysis Programs
Lastly, statistical analysis programs play an undeniable role in the interpretation of resultant data from RNA sequencing. These programs, like R and Python libraries, offer researchers the analytical prowess necessary for deeper insights into their datasets. They help in deciphering biological significance through rigorous hypothesis testing and data visualization techniques.
A distinctive feature that makes these programs so esteemed in the scientific community is their flexibility and customizability. These programs allow researchers to craft tailored analyses that fit their specific research requirements, thereby increasing the robustness of findings.
The advantages of statistical analysis programs include their ability to integrate vast datasets from various sources and facilitate complex analyses. Conversely, these tools may require a fair amount of statistical knowledge, which can hinder those who lack a strong mathematical background.
Interpreting Sequencing Data
After extracting and analyzing data, interpreting sequencing outcomes is where researchers find value. This process involves understanding the biological implications of the data, requiring a comprehensive grasp of the experimental context and the underlying biology of the organisms studied.
Successfully interpreting sequencing data hinges on a blend of computational insight and biological comprehension. With the integration of robust statistical models, researchers can draw more informed conclusions, crucial for advancing our understanding of microbial ecosystems.
Applications of Bacterial RNA Sequencing
The implications of bacterial RNA sequencing extend beyond the confines of the laboratory, influencing various domains including environmental science, medicine, and agricultural practices. Understanding these applications not only reveals the versatility of RNA sequencing techniques but also underscores their pivotal role in modern biological research. This section delves into two major categories of application: research and development, alongside clinical implications, emphasizing their significance in each field.
Research and Development
Gene Expression Studies
Gene expression studies are central to deciphering the complexities of bacterial life. Through analyzing mRNA levels, researchers can pinpoint how bacteria respond to environmental changes or stressors. This analysis allows for a deeper understanding of metabolic pathways and the identification of key regulatory genes. Gene expression studies provide a window into the dynamic nature of bacterial populations, showcasing how they can adapt to various stimuli.
A key characteristic of gene expression studies is their ability to capture the temporal changes in RNA levels. This makes it a popular choice for researchers aiming to understand how bacterial cells modulate their responses over time.
Notably, one unique feature of these studies is their reliance on advanced sequencing technologies, which can provide insights at resolutions that were previously unattainable. However, the disadvantage lies in the requirement for high-quality RNA samples. Any degradation can lead to skewed results, complicating data interpretation.
Regulatory Network Analysis
Regulatory network analysis investigates the intricate web of interactions that control gene expression in bacteria. This approach is vital in understanding how genes cooperate and react to various external factors, essentially revealing the governance of cellular processes.
The key characteristic of regulatory network analysis is its potential to map out complex relationships between genes, proteins, and metabolites. This capability makes it an invaluable tool for researchers working to untangle the pathways that dictate bacterial behavior. One unique feature is the ability to employ computational models that combine sequencing data with predictive analytics, enhancing our understanding of regulatory mechanisms. However, constructing reliable models can be a daunting task, often hindering effective conclusions due to the intricacies of biological systems.
Clinical Implications
Antibiotic Resistance Studies
Antibiotic resistance studies utilize bacterial RNA sequencing to decipher the genetic basis of resistance mechanisms. In an era where multi-drug resistant pathogens pose significant threats to public health, understanding these mechanisms is crucial. Through this analysis, researchers can identify specific genes responsible for resistance, paving the way for novel therapeutic strategies.
A beneficial aspect of antibiotic resistance studies is the insight they provide into prevalent resistance genes in clinical isolates. This information not just aids in treatment decision-making but also informs public health strategies. One notable feature of these studies is the capability to track the evolution of resistance in real time. On the flip side, the extensive data generated can be overwhelming, making it challenging to discern clinically relevant information amidst potential noise.
Pathogen Identification
Pathogen identification has been revolutionized by RNA sequencing, enabling rapid and precise diagnosis of infectious diseases. By analyzing the RNA profiles of suspected pathogens, researchers can differentiate between closely related species, considerably improving identification accuracy.
The key advantage of RNA-based pathogen identification is its ability to detect not only the presence of a pathogen but also its active state in a given sample. This means that researchers can ascertain if a bacterium is alive and, therefore, potentially pathogenic. One unique feature is the adaptability of sequencing protocols to various sample types, from clinical specimens to environmental samples. However, the challenge often lies in the interpretation of complex data, requiring specialized knowledge and tools to translate findings into clinical action.
In summary, the applications of bacterial RNA sequencing in research and clinical settings are crucial for advancing our understanding of microbiology and improving public health outcomes. The insights garnered from these applications highlight the profound impact of RNA sequencing technologies in both fundamental research and practical healthcare.
Challenges in Bacterial RNA Sequencing
Bacterial RNA sequencing, while pioneering and invaluable in biological research, isn't without its hurdles. Understanding these challenges becomes critical for researchers and practitioners who aim to harness its full potential. Each issue presents not only obstacles but also areas for improvement and innovation. This segment will delve into two main challenges: the technical limitations inherent to the methodologies used and the complexities surrounding data overload and interpretation issues.
Technical Limitations
The technical limitations in bacterial RNA sequencing often stem from the very nature of the sampling and sequencing processes.
- Species Diversity: One of the foremost challenges arises from the vast diversity among bacterial species. This diversity can lead to uneven coverage of RNA transcripts, meaning some bacterial genes may be underrepresented in the sequencing output. As a result, researchers may miss critical genetic insights due to insufficient sampling of specific organisms.
- RNA Stability: The stability of RNA is another hurdle. RNA is notoriously fragile and can degrade rapidly if not handled or stored correctly. This makes experiments highly sensitive to delays or improper procedures in extraction and processing.
- Contamination Risks: During RNA extraction, bacteria may be contaminated by other microbial species. This can skew results. In a mixed culture environment, distinguishing RNA from target bacteria amidst the background noise can be an exercise in futility.
"The challenges in RNA sequencing are not just technical barriers; they highlight the need for precise techniques to ensure the integrity and comprehensiveness of the data."
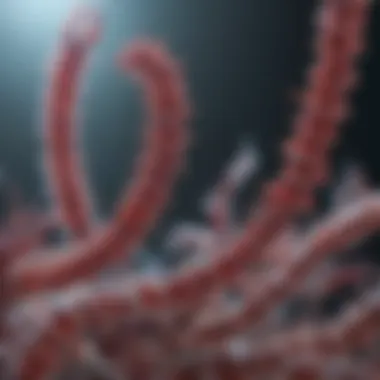
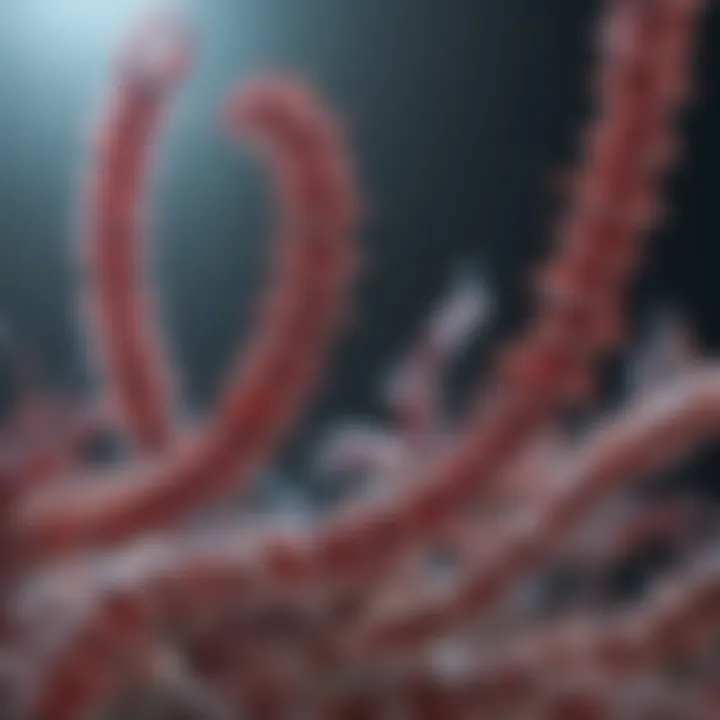
Given these limitations, addressing them requires development of improved methodologies and stringent procedural controls.
Data Overload and Interpretation Issues
Data overload represents a significant challenge following the sequencing process. With vast amounts of sequence data generated, the real challenge is in the analytical phase.
- Complexity of Analysis: The sheer volume of data produced can overwhelm standard bioinformatics tools. Often, researchers find themselves with mountains of data yet lacking the resources or computational power to analyze it effectively.
- Precision vs Volume: There's a trade-off between the amount of data collected and the depth of analysis. Sometimes, focusing on broad trends in gene expression can lead to oversimplification; important nuances might be overshadowed by the sheer amount of data.
- Interpretation of Findings: Even when the data has been processed, interpreting it can be an arduous task. Researchers must navigate through statistical noise and ascertain relevant biological insights from the results. Misinterpretation can lead to erroneous conclusions, affecting subsequent research or even clinical applications.
Advancements and Innovations
In the realm of bacterial RNA sequencing, advancements and innovations are not merely conceptual; they represent pivotal shifts that can redefine our understanding of microbial life. These developments have enhanced the ability to analyze bacterial behavior, gene expression, and the complex relationships within microbial communities. The significance of exploring these elements is manifold.
One of the defining characteristics of progress in this field has been the move toward more high-throughput methodologies. With the ability to sequence multiple samples simultaneously, researchers can gather extensive data that elucidate even subtle variations in gene expression across different environmental conditions or treatment scenarios. This is critical for understanding how bacteria adapt and respond to their surroundings.
Across the board, advancements are inching us closer to real-time sequencing capabilities, allowing scientists to monitor bacterial dynamics as they happen. Such a capability can transform our approach to studying pathogenic bacteria, helping us intervene in significant infections by tracking their genetic changes almost instantaneously. This level of insight was unfathomable just a few years ago.
Emerging Technologies
The landscape of bacterial RNA sequencing is being reshaped by emerging technologies. Innovations such as single-cell RNA sequencing are especially noteworthy, as they allow for the examination of individual bacterial cells rather than averaging results across a population. This reveals heterogeneity in gene expression, which can often be the key to understanding adaptations and resistance mechanisms.
Other technologies include:
- Nanopore sequencing, known for its portability and efficiency which can significantly shrink the timeframe from sample to results.
- CRISPR-based sequencing methods, which enhance specificity in capturing RNA populations, thus allowing a more nuanced view of gene expression trends.
The adoption of these technologies brings with it opportunities for customization in experimental design. For instance, researchers can tailor sequencing strategies to target specific bacterial types or behaviors, rendering the process both efficient and relevant.
Integration of Machine Learning
Incorporating machine learning into bacterial RNA sequencing offers a fascinating glimpse into the potential for data-driven discoveries. The sheer volume of data generated through sequencing can overwhelm traditional analysis methods; machine learning algorithms can help to parse through this extensive information systematically.
The magic lies in the ability of these algorithms to identify patterns that may not be immediately discernible to human eyes. Consider that fluctuations in gene expression across various conditions can be analyzed, allowing for predictions about bacterial behavior in response to environmental changes. This kind of intelligence can directly influence the development of antibiotics and therapeutics tailored to target specific bacterial responses.
For instance, algorithms can be trained to recognize signatures of antibiotic resistance within bacterial RNA, thus facilitating more timely and informed decision-making in clinical settings. This merger of technology and biology is exceptionally promising and could pave the way for unprecedented breakthroughs in personalized medicine, targeting bacterial infections more effectively.
The integration of machine learning solutions into RNA sequencing marks a paradigm shift, highlighting a future where bioinformaticians and biologists work hand-in-hand to decipher the story hidden in genetic data.
In summary, as we explore the advancements and innovations central to bacterial RNA sequencing, it is clear that the potential for impactful discoveries is vast. Researchers stand on the cusp of a transformative era, one that not only promises to enhance our understanding of microbiology but also reshapes methodologies in clinical and environmental applications.
Future Directions in Bacterial RNA Sequencing
The field of bacterial RNA sequencing is poised at the brink of significant breakthroughs. As researchers continue to explore this domain, the importance of future directions cannot be overstated. New techniques, evolving technologies, and interdisciplinary approaches promise to enhance our understanding of microbial systems and their interactions. The future of bacterial RNA sequencing not only aims at refining existing methodologies but also seeks to open new avenues for exploration in microbial ecology and therapeutic applications.
These advancements are highly relevant, as they may contribute substantially to our understanding of gene regulation, antibiotic resistance mechanisms, and microbial interactions within ecosystems. Rather than merely being an accumulation of data, the integration of innovative technologies will allow scientists to paint a more holistic picture of bacterial life and behavior.
Potential Research Avenues
Several potential research avenues are emerging within the field of bacterial RNA sequencing:
- Single-Cell RNA Sequencing: This technique has the power to uncover the complexities of gene expression at the single-cell level, helping to reveal how individual bacteria respond to their environment. Insights gleaned from this approach could drastically alter our understanding of heterogeneity among microbial populations.
- Longitudinal Studies: Examining RNA changes over time in response to environmental pressures or treatment scenarios could lead to a deeper comprehension of bacterial adaptation and resistance mechanisms. Such studies may yield pivotal information for developing new antibiotic strategies.
- Integration with Metagenomics: Combining bacterial RNA sequencing with metagenomic approaches could provide a more robust picture of the interactions occurring within microbial communities. By understanding the gene expression profiles in tandem with the genetic makeup of entire communities, researchers could trace the pathways of microbial metabolism and its implications for biogeochemical cycles.
In essence, these avenues invite a fresh paradigm of interrogating microbial life, guiding researchers towards answers that can have profound implications for ecology, health, and industrial applications.
Impact on Microbial Ecology Studies
The impact of bacterial RNA sequencing on studies of microbial ecology cannot be overlooked. By offering real-time insights into microbial gene expression, this sequencing technology enhances our ability to understand complex ecological interactions. Here are several notable ways in which it is making a difference:
- Understanding Community Dynamics: RNA sequencing enables researchers to assess how microbial communities fluctuate in response to environmental changes or disturbances. This understanding enhances our capability to predict community responses to future changes.
- Identifying Key Players: By capturing the transcriptomic landscape, researchers can pinpoint which organisms are actively influencing processes like nutrient cycling or disease in a given ecosystem. This can lead to targeted strategies for managing ecosystems or mitigating disease outbreaks.
- Facilitating Better Models: Improved data from RNA sequencing can refine ecological models, allowing for enhanced prediction of interactions within microbial communities. This helps in understanding not just individual species behavior, but the collective impact of diverse microorganisms.
Predictive models informed by RNA sequencing data can ultimately guide conservation strategies for endangered ecosystems and inform bioremediation efforts in polluted environments.
In summary, the future of bacterial RNA sequencing is laden with promising prospects. As we refine methodologies and enhance our approach to integrate this tool into broader ecological studies, the potential emerges for revolutionary discoveries that could reshape our understanding of both bacterial biology and ecological health.
Feelings about this topic are not just academic; they represent a crucial interface between human health, environmental sustainability, and technological innovation.
Closure
Bacterial RNA sequencing holds a vital position in microbial studies, aiding in the understanding of complex biological systems. This method transcends mere genetic cataloging; it unlocks the intricate roles of genes and their expressions within various environments. The implications of bacterial RNA sequencing touch upon numerous domains, from clinical applications such as pathogen identification to research initiatives examining evolutionary dynamics within microbial communities.
Summary of Key Insights
In summary, the exploration of bacterial RNA sequencing presents several key insights:
- Impact on Gene Expression Analysis: This methodology sheds light on gene expression profiles, identifying active genes under specific conditions. It paves the way for novel discoveries in gene function and regulation.
- Technological Advancements: Continuous enhancements in sequencing technologies have sharpened the precision of RNA detection and quantification, making it more accessible for researchers worldwide.
- Environmental Relevance: Analyzing RNA provides a snapshot of microbial activity in diverse habitats, far surpassing traditional DNA-based methods, which often fail to account for transient or active forms of RNA.
- Challenges in Data Handling: While the benefits are profound, challenges persist. The sheer volume of data generated necessitates robust bioinformatics tools to ensure accurate interpretation and meaningful insights.
Final Thoughts on Bacterial RNA Sequencing
As we look toward the future, bacterial RNA sequencing will become increasingly influential. The potential for integration with machine learning techniques is likely to further augment the analytical power of this field. Moreover, the availability of various sequencing platforms fosters innovation and adaptation in research practices.
It's clear that bacterial RNA sequencing is not merely a technical endeavor; it's a window into the living world of microbes. As the scientific community continues to delve into the complexities of microbial life, understanding these dynamics through RNA sequencing will be instrumental in addressing broader ecological challenges and advancing medical research. This capacity to interpret microbial behavior ultimately holds promise for real-world applications, from environmental conservation to public health.