Exploring Viral Vectors in Gene Therapy
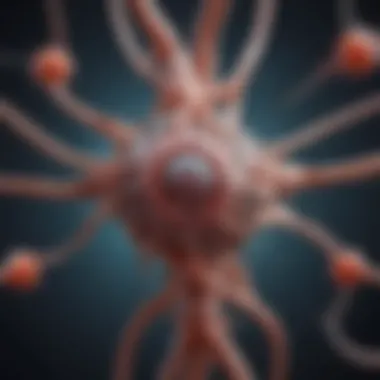
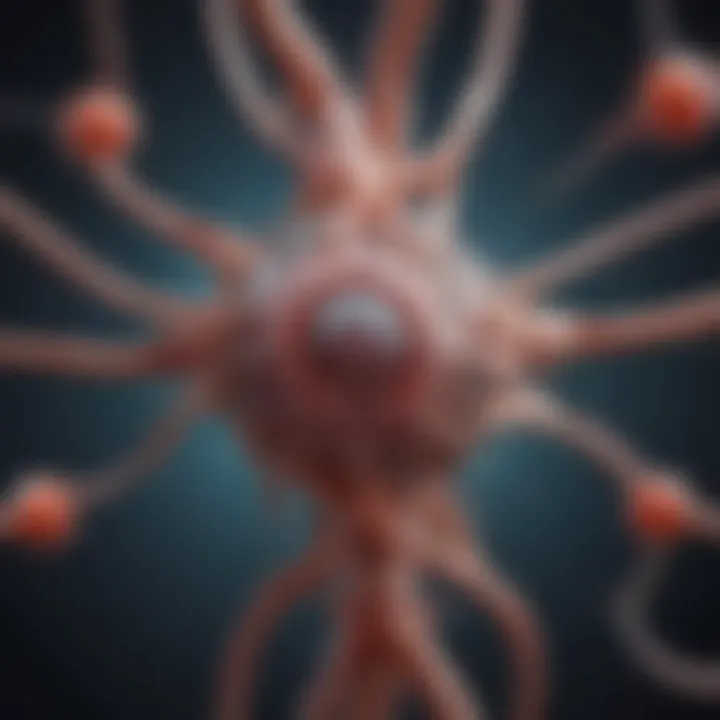
Intro
The landscape of gene therapy is intricate and rapidly evolving. Viral vectors play a crucial role in this field, serving as vehicles to deliver genetic material into cells. Understanding these vectors, their types, and their mechanisms is essential for advancing therapeutic approaches. Gene therapy aims to treat or even cure genetic disorders by altering defective genes. The selection of an appropriate viral vector is a fundamental step in this process.
Methodology
Overview of Research Methods Used
This article synthesizes available literature on viral vectors, focusing on aspects such as their biology, engineering, and application in gene therapy. A systematic review of recent studies and clinical trials informs the classification and evaluation of each vector's efficiency and effectiveness.
Data Collection Techniques
To gather information, various academic databases were explored including PubMed, ScienceDirect, and articles from prominent journals such as "Nature" and "The Journal of Gene Medicine". The emphasis was on peer-reviewed studies that present data on the use and effectiveness of different viral vectors in human therapies. Comparative analyses of case studies were also utilized to highlight successes and challenges associated with specific vectors.
Types of Viral Vectors
Gene therapies rely on different viral vectors, each with unique properties. Below are the main types:
Retroviruses
Retroviruses are RNA viruses that integrate into the host's genome. Their ability to induce stable gene expression makes them suitable for certain gene therapies. However, they pose risks such as insertional mutagenesis, leading to oncogenesis in some cases.
Adenoviruses
Adenoviruses are potent vectors that can carry large genetic payloads. They do not integrate into the host genome, reducing long-term risks. Despite this advantage, their transient expression and immune responses limit effectiveness in some applications.
Adeno-Associated Viruses (AAV)
Adeno-associated viruses are small viruses that are particularly useful for gene delivery due to their low immunogenicity and ability to induce long-lasting effects. AAVs can persist in the host's cells without integration, which lowers the risk of disrupting essential genes.
Lentiviruses
Lentiviruses, a subclass of retroviruses, can infect dividing and non-dividing cells alike. They offer stable expression of therapeutic genes. Like retroviruses, there is a potential risk of insertional mutagenesis. However, advancements in vector design have aimed to reduce these risks.
Future Directions
Upcoming Trends in Research
The field of viral vectors continues to evolve. Key trends include the development of more targeted delivery systems that improve the specificity of gene therapy. Innovations may include engineered nanoparticles combined with viral vectors to enhance targeting and reduce side effects.
Areas Requiring Further Investigation
Despite advancements, challenges remain. Further research is needed to understand the long-term effects of gene therapies using various viral vectors. Evaluating immune responses and refining vector design to minimize adverse effects are priorities in ongoing research.
A deeper understanding of viral vectors will enhance their applications in gene therapy and significantly impact various genetic disorders.
Prelims to Gene Therapy
Gene therapy represents a promising frontier in modern medicine, targeting the root of genetic diseases by correcting or replacing defective genes. This innovative approach seeks to mitigate the effects of inherited conditions, genetic disorders, and even some acquired diseases. The importance of understanding gene therapy lies in its potential to revolutionize treatment paradigms and improve patient outcomes by addressing the underlying genetic issues at their source.
This section offers foundational knowledge that sets the stage for discussing various viral vectors. These vectors are essential for delivering therapeutic genes into target cells, facilitating the gene therapy process. Their characteristics, effectiveness in gene delivery, and clinical applications are central to comprehending gene therapy as a field. Understanding the fundamentals of gene therapy is crucial for professionals, educators, and researchers as they explore the intricate dynamics between viral vectors and therapeutic applications.
Definition and Historical Context
Gene therapy refers to the technique that modifies a person's genes to treat or prevent diseases. The idea of manipulating genes for therapeutic purposes dates back several decades, with initial experimentation and conceptual foundations laid in the 1970s and 1980s. The first clinical trial to apply gene therapy was conducted in 1990, involving the treatment of a young girl with severe combined immunodeficiency (SCID). Despite successes, the journey has not been without challenges. Early trials faced numerous setbacks, including safety concerns and ethical debates, leading to a temporary halt in progress.
Throughout the years, technology and knowledge have evolved. Advances in molecular biology and genetic engineering have paved the way for safer and more effective methods in gene manipulation. The historical context of gene therapy highlights its iterative nature, where each success informs future directions, and challenges act as learning experiences that shape ongoing research and development.
Importance of Viral Vectors
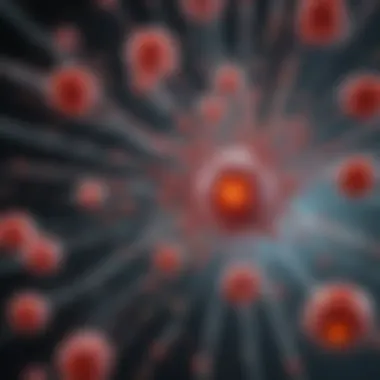
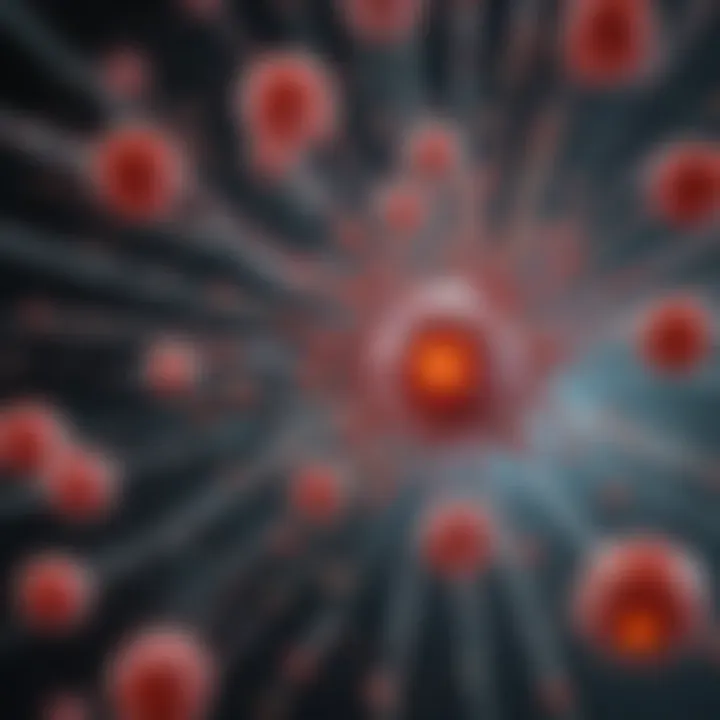
Viral vectors are pivotal in the execution of gene therapy. They serve as vehicles to deliver therapeutic genes into cells effectively. The importance of viral vectors can be emphasized through several key points:
- Efficiency of Gene Delivery: Viral vectors can penetrate cells more efficiently than non-viral methods. Their natural ability to infect host cells means they can introduce genetic material with high efficacy.
- Diversity of Options: Different types of viral vectors, such as retroviruses, adenoviruses, and adeno-associated viruses, provide a range of choices depending on the specific needs of the gene therapy application. Each vector type has distinct characteristics, making them suitable for various therapeutic scenarios.
- Potential for Long-term Expression: Some viral vectors can integrate into the hostβs genome. This insertion can lead to long-term expression of the therapeutic gene, offering sustained benefits for patients with chronic conditions.
- Advancements in Safety: Recent developments have resulted in engineered viral vectors that exhibit reduced immunogenicity and targeted delivery, which enhances their safety profiles and efficacy for clinical application.
"Viral vectors embody the bridge between genetic understanding and therapeutic application; their importance cannot be overstated in the context of advancing gene therapy rigorously."
In summary, the pivotal role that viral vectors play in gene therapy underscores their significance in modern medicine. Understanding the utility of these vectors is essential for researchers and clinicians who aim to leverage gene-based therapies for improved patient health.
Understanding Viral Vectors
Understanding viral vectors is fundamental for comprehending advancements in gene therapy. Viral vectors act as delivery vehicles, transporting genetic material into cells. This task is crucial because many genetic disorders arise from defective genes. By employing these vectors in therapeutic contexts, researchers aim to restore normal function through gene correction or replacement.
The significance of viral vectors in gene therapy lies in their ability to carry and introduce therapeutic genes safely and effectively. They exploit natural mechanisms from viruses that already transduce cells efficiently. Thus, viral vectors can facilitate more precise targeting of the genes of interest compared to traditional methods.
Additionally, the selection of the appropriate viral vector is vital for the specific application intended. Factors such as the desired duration of gene expression and the cell types targeted greatly influence this choice.
Professional research in this field reveals the ongoing advances in vector engineering that enhance their specificity, tropism, and safety. The importance of understanding how these vectors function enables researchers to creatively address challenges and develop successful therapies for previously untreatable conditions.
What is a Viral Vector?
A viral vector is a modified virus used to deliver genetic material into cells. This process is primarily for therapeutic purposes, such as correcting defective genes or introducing new genes to help fight diseases. The viral component is manipulated to remove pathogenic properties while retaining its ability to deliver genetic material.
Viral vectors can come from various virus families, each belonging to different categories. Notably, the most commonly employed vectors include retroviruses, adenoviruses, and adeno-associated viruses.
Key characteristics of viral vectors include:
- Tropism: The specific cell types a viral vector can infect.
- Capacity: The amount of genetic material a vector can carry.
- Durability: How long the introduced gene remains functional within the target cells.
These features dictate how effective a viral vector can be in specific therapeutic contexts.
Mechanisms of Viral Transduction
Viral transduction involves several steps, allowing the virus to infect a host cell and deliver genetic material. The mechanisms can vary based on the type of viral vector utilized.
- Attachment: The virus binds to specific receptors on the cell surface. These receptors determine the specificity of the viral vector.
- Entry: The viral particle enters the cell, either through membrane fusion or endocytosis. The method of entry can influence the efficiency of gene transfer.
- Release of Genetic Material: Once inside the cell, the virus sheds its protein coat, releasing its genetic material into the cytoplasm or directly into the nucleus.
- Integration or Expression: Depending on the vector type, the genetic material may integrate into the host genome or exist as an episome. The latter entails transient expression without integration.
Types of Viral Vectors
Viral vectors play a critical role in the development and application of gene therapy. Their unique ability to deliver genetic material into cells allows for targeted gene editing and therapy, which can significantly advance treatment options for various genetic disorders. Understanding the different types of viral vectors is essential as each type possesses unique features and capabilities. These characteristics influence their functional effectiveness and suitability for specific therapies. Notably, careful consideration of the chosen vector can determine treatment success, making this topic paramount in the field of gene therapy.
Retroviruses
Characteristics of Retroviruses
Retroviruses are a class of RNA viruses known for their ability to integrate into the host cell's genome. A notable characteristic is their reverse transcriptase enzyme, which converts their RNA into DNA. This allows retroviruses to integrate into the DNA of the host cell, which can lead to stable and long-term expression of the therapeutic gene. The integration capability of retroviruses makes them a preferred choice for applications requiring persistent gene expression. However, this characteristic can also result in potential insertional mutagenesis, which poses risks to the host.
Applications in Gene Therapy
In gene therapy, retroviruses are commonly used to treat various genetic disorders. They have been applied effectively in therapies for conditions like severe combined immunodeficiency (SCID) and beta-thalassemia. Their ability to stably integrate enables long-lasting therapeutic benefits, which is crucial for chronic conditions. Despite their effectiveness, the reliance on dividing cells for successful transduction limits their application in non-dividing cells such as neurons.
Advantages and Limitations
One advantage of retroviruses is their robust gene delivery system which ensures sustained gene expression over time. However, limitations include the risk of insertional mutagenesis and their dependence on host cell division. This makes retroviruses less suitable for therapies targeting non-dividing cells. Furthermore, immune responses can limit their effectiveness in vivo.
Adenoviruses
Characteristics of Adenoviruses
Adenoviruses are non-enveloped viruses with double-stranded DNA genomes. They are characterized by their large capacity for genetic material insertion, allowing the introduction of substantial therapeutic genes. A significant aspect of adenoviruses is their ability to infect both dividing and non-dividing cells, which enhances their versatility as a vector choice for various treatments.
Applications in Gene Therapy
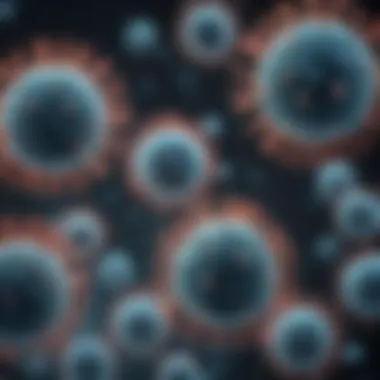
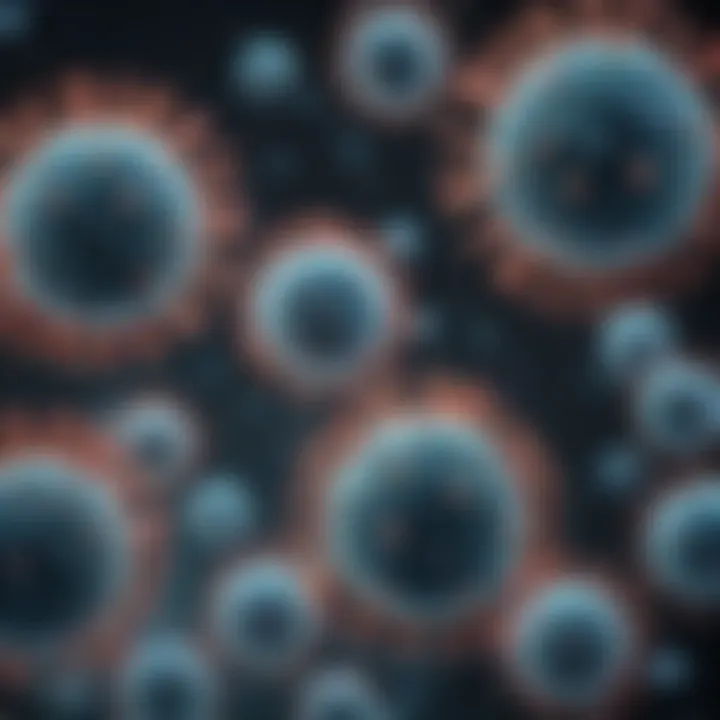
In gene therapy, adenoviruses are used for delivering genes to treat a range of diseases, including cancer and genetic disorders like cystic fibrosis. Their robust infectivity and ability to induce strong immune responses can be harnessed for vaccine development as well. However, this immunogenicity can also present challenges, necessitating careful assessment in therapeutic contexts.
Advantages and Limitations
The primary advantage of adenoviruses lies in their capacity to carry large amounts of genetic material and infecting many cell types. However, their significant immunogenicity can lead to transient expression of the therapeutic gene. They often trigger strong immune responses that limit their effectiveness in long-term therapies. Additionally, repeated administration can be problematic due to pre-existing immunity in the patient population.
Adeno-Associated Viruses (AAV)
Characteristics of AAV
Adeno-associated viruses are small viruses that can integrate into the host genome but have a low risk of causing disease. Their advantages include a relatively simple structure and the ability to produce a stable immune response. A notable characteristic is their small payload capacity, making them suitable for delivering smaller therapeutic genes effectively.
Applications in Gene Therapy
AAVs are used to treat numerous genetic disorders such as hemophilia and muscular dystrophy. Their ability for long-term expression without harmful immune responses makes them suitable for chronic conditions. AAVs have gained traction in ocular gene therapies, where they deliver genes to retinal cells effectively.
Advantages and Limitations
The significant advantage of AAVs is their safety profile and prolonged expression of therapeutic genes. However, their lower packaging capacity can be a limitation when larger genes need to be delivered. Additionally, pre-existing immunity in patients may affect the efficacy of AAV treatments, limiting their widespread application.
Lentiviruses
Characteristics of Lentiviruses
Lentiviruses, a subset of retroviruses, have the capability to infect non-dividing cells, a feature that significantly expands their potential applications. They possess a unique ability to integrate into the host genome efficiently. Their adaptability stems from effective mechanisms allowing stable gene incorporation, which enhances therapeutic longevity.
Applications in Gene Therapy
Lentiviruses are widely applied in gene therapy protocols, particularly for hematological disorders. They have been notably used in therapies for sickle cell disease and certain types of cancers. Their ability to transduce non-dividing cells like neurons is a powerful asset for neurological therapies.
Advantages and Limitations
One clear benefit of lentiviruses is their ability to provide stable, long-term gene expression across diverse cell types. However, the risk of insertional mutagenesis and potential safety concerns pose significant challenges. Regulatory hurdles also impact the development of lentiviral therapies, which can slow their application in real-world settings.
Other Emerging Viral Vectors
Herpes Simplex Virus Vectors
Herpes simplex virus vectors are gaining attention in gene therapy due to their large genome capacity and ability to infect neurons effectively. This characteristic makes them suitable for targeting neurological disorders. They can deliver therapeutic genes with good efficiency and can establish latency, offering potential for long-term therapy.
Sendai Virus Vectors
Sendai virus vectors are promising due to their non-integrating nature and capacity to transduce a variety of cell types, including stem cells. They serve as useful tools for gene editing and regenerative medicine, presenting an advantage in terms of safety without risk of insertional mutagenesis.
Newly Engineered Viral Vectors
The field is evolving with efforts to engineer viral vectors to overcome existing limitations. New technologies aim to improve specificity, reduce immune responses, and enhance delivery capacity. These advancements are paving the way for safer and more effective gene therapies in the future.
"Understanding the different types of viral vectors is essential as each type possesses unique features and capabilities."
Evaluating Efficacy and Safety
The evaluation of efficacy and safety is a critical component in the use of viral vectors for gene therapy. As these vectors are intended to deliver therapeutic genes to specific cells, their performance directly influences the clinical outcome. Understanding the balance between effective gene delivery and the potential for adverse effects is essential. Safety concerns can significantly impact patient outcomes and acceptance of new therapies. It is paramount for researchers and clinicians to establish protocols that assess both the desirable and undesirable effects of these vectors.
A rigorous evaluation process involves examining the efficiency of the viral vector in achieving its intended function, such as the successful incorporation and expression of the therapeutic gene within target cells. This assessment is particularly important as it helps identify how factors such as vector type, delivery method, and patient-specific conditions can influence effectiveness. By determining the optimal conditions under which these vectors perform, researchers can optimize their usage in clinical settings.
In addition, an understanding of safety considerations must include monitoring for unintended genetic modifications, immunogenic responses, and overall patient well-being. Addressing these issues ensures that the potential risks do not outweigh the benefits of gene therapy. This careful scrutiny forms the backbone of clinical trials, guiding advancements in gene therapy techniques.
Clonal Variation in Gene Delivery
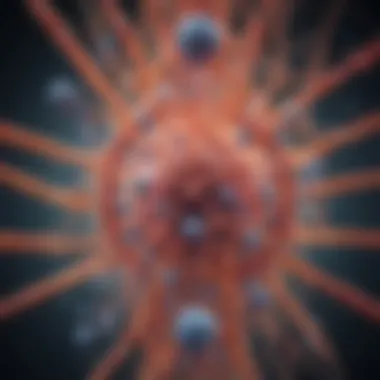
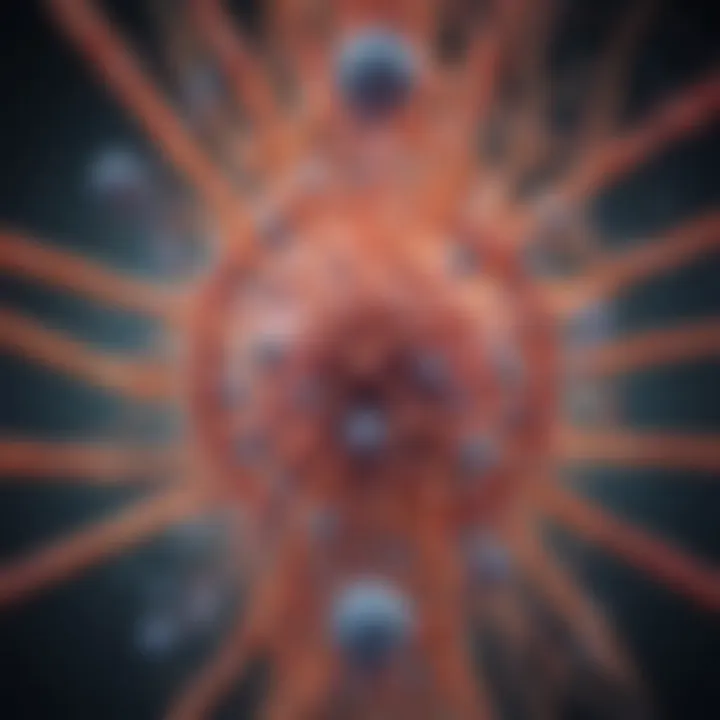
Clonal variation refers to the differences in how various clones of cells respond to gene delivery via viral vectors. This phenomenon is significant in gene therapy as it can influence the overall success of treatment modalities. Different cells can exhibit varying levels of susceptibility to viral vectors, which potentially affects the efficiency of gene transfer.
One must appreciate that the genetic and phenotypic heterogeneity among target cells contributes to this variation. For instance, not all cells within a tissue or tumor will express the same receptors, impacting how effectively a viral vector can enter and deliver its payload. Furthermore, the growth environment can also play a role. Factors such as hypoxia or nutrient availability can alter cellular response to viral infections, influencing the overall success of treatment.
To mitigate these effects, ongoing research focuses on identifying markers that can predict which cells will respond favorably to specific vectors. By improving our understanding of clonal variation, we can enhance vector targeting and improve therapeutic outcomes.
Immunogenic Reactions
Immunogenic reactions present a significant challenge in the use of viral vectors for gene therapy. The body's immune system is designed to recognize and eliminate foreign particles, including viral vectors. Therefore, any immune response can compromise the effectiveness of the gene therapy. An immune reaction may not only reduce the efficiency of vector delivery but can also lead to harmful side effects.
When evaluating these reactions, there are two main types to consider:
- Adaptive immune responses: These include T-cell mediated responses and antibody production. They can lead to clearance of the therapeutic vector before it achieves its intended purpose.
- Innate immune responses: This involves immediate responses from the immune system, often resulting in inflammation. Such responses can vary based on the vector used, with some vectors eliciting stronger reactions than others.
It is crucial to preform preclinical studies that assess the immunogenicity of viral vectors before initiating human trials. Adjusting the design of vectors to reduce their immunogenicity while maintaining efficacy is a focus of current research efforts. Strategic engineering and delivery methods can help create vectors that minimize pathogenic responses, allowing for more effective and safer applications of gene therapy.
"A thorough understanding of both clonal variation and immunogenic reactions is essential to fully realize the potential of viral vectors in gene therapy. Only then can we advance the field and improve patient outcomes."
Future Directions in Viral Vector Research
Viral vector research is continuously evolving, reflecting advances in genetic engineering and a deeper understanding of viral mechanisms. This section explores the significance of research in this area. Future directions shape therapeutic applications, enhance safety profiles, and improve efficiency in gene delivery.
One major focus is the optimization of existing viral vectors. This involves modifying viral genomes to increase their therapeutic efficacy while minimizing side effects. For instance, genetic engineering of viral vectors is crucial for tailoring them to specific cell types, thereby improving the specificity of treatments for genetic disorders.
Another important aspect is the development of next-generation viral vectors. These encompass newly engineered vectors with enhanced capabilities. Emerging technologies, such as CRISPR gene editing, offer promising approaches to create viral vectors that can target specific genetic mutations more effectively. This strategic targeting can potentially revolutionize how we approach treatments for genetic diseases.
"Future research will not only refine existing vectors but also introduce innovative platforms that enhance our ability to deliver gene therapies more safely and effectively."
Furthermore, addressing the immunogenicity of viral vectors remains vital. Reducing the immune response to these vectors is necessary for improving the longevity and efficacy of gene therapies. Therefore, researchers are investigating strategies to modify viral proteins or shield them from immune detection.
Genetic Engineering of Viral Vectors
Genetic engineering plays a pivotal role in advancing viral vectors used in gene therapy. By manipulating viral genomes, researchers can enhance the delivery and expression of therapeutic genes. This engineering allows the modification of various aspects such as vector tropism, replication capacity, and the expression of foreign genes. Tuning these factors can lead to greater control over the therapeutic outcomes and minimizes unintended genetic changes.
For example, tailoring the surface proteins of viral vectors can enhance their affinity for specific cell types. Such specificity increases the chances of effective gene transfer, particularly in target tissues like the liver or muscle. Additionally, researchers are exploring the engineering of helper viruses to improve the stability and delivery of gene payloads.
Thus, genetic engineering of viral vectors ensures more effective, targeted, and safer gene therapies. This approach opens doors for personalized medicine, where therapies can be custom-made based on individual genetic profiles.
Enhanced Delivery Mechanisms
Delivery mechanisms are as critical as the vectors themselves in the realm of gene therapy. Enhancements in delivery mechanisms aim to ensure efficient, precise, and safe delivery of genetic material to target cells. Researchers are examining strategies to promote passive and active targeting of viral vectors.
One promising method involves using biomaterials such as nanoparticles that can escort viral vectors to the desired sites in the body. These nanoparticles can further protect the vectors from degradation and promote their endocytosis by target cells. This hybrid approach can increase the circulation time of viral vectors in the bloodstream, enhancing their overall effectiveness.
Moreover, the use of tracers or imaging techniques is gaining traction. These technologies allow for real-time tracking of viral vectors within the body, providing valuable insight into their distribution and interactions with target cells. With better tracking, researchers can optimize protocols further, adjusting dosages and delivery timings for improved outcomes.
Overall, enhancing delivery mechanisms is critical. It not only improves the success rate of gene therapies but also contributes to their safety and efficiency. As these mechanisms evolve, the possibilities for innovative treatments that can address a myriad of genetic disorders become increasingly feasible.
Epilogue
In evaluating the role of viral vectors in gene therapy, it is vital to recognize the significant advancements that they enable in treating genetic disorders. This article has explored various types of viral vectors, their specific characteristics, applications, and the inherent limitations they possess. Understanding these elements is essential not only for researchers and clinicians but also for students and educators who seek to grasp the ongoing evolution of gene therapy methodologies.
The conclusion emphasizes that while the prospects of gene therapy are promising, particularly with the rapid development of viral vectors, careful consideration must be given to long-term effects and safety profiles associated with these vectors. The discussion outlined here provides a basis from which future researchers can build.
"Advances in viral vector technology are critical to the success of gene therapy and its mainstream application in clinical settings."
Summary of Key Points
- Diverse Viral Types: Each viral vector type discussed, including retroviruses, adenoviruses, AAV, and lentiviruses, has unique traits tailored for specific therapeutic needs.
- Mechanisms of Action: Understanding how these vectors deliver therapeutic genes is crucial for improving efficacy and safety in treatments.
- Applications and Limitations: The practical applications of these vectors range from cancer treatment to genetic disorders, though limitations like immune response and insertional mutagenesis need addressing.
- Future Research Directions: The field is rapidly evolving, with genetic engineering and enhanced delivery systems poised to expand the capabilities of existing vectors.
Implications for Future Research
The implications for future research in viral vectors are manifold. Enhancing the specificity and efficiency of gene delivery can lead to better therapeutic outcomes. Exploring new viral vector engineering methods, such as the use of CRISPR technology, might allow for even more targeted approaches to gene therapy.
Moreover, understanding immunogenic responses will be essential for ensuring patient safety. The development of novel viral vectors may necessitate interdisciplinary collaboration, combining insights from genetics, virology, and bioengineering.
Continued investment in this area will yield the potential for breakthrough therapies that could one day address previously untreatable conditions, reinforcing the need for ongoing exploration and evaluation of viral vectors in gene therapy.