Understanding Radiation Dose in Cancer Treatment
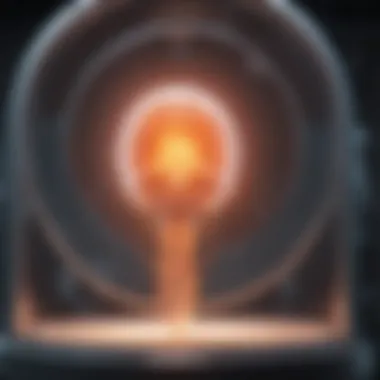
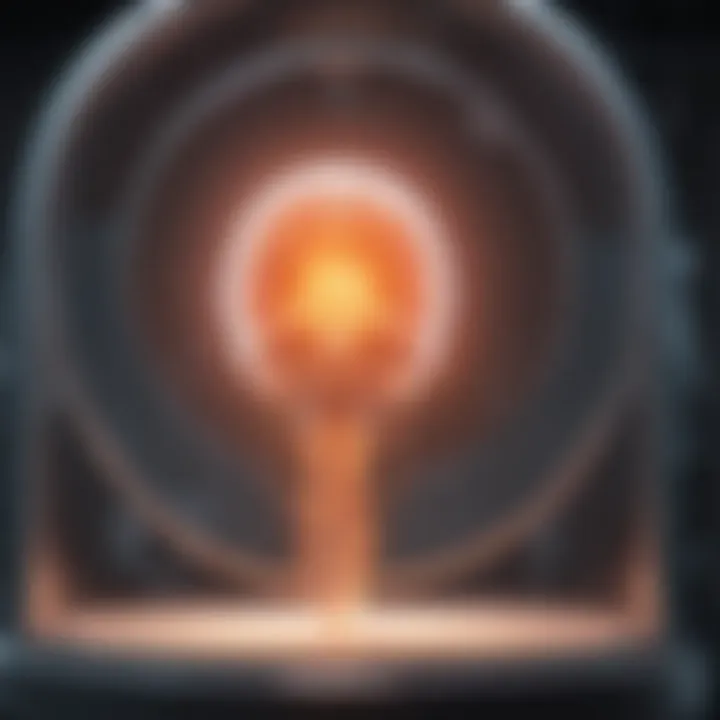
Intro
Radiation therapy is a cornerstone in the arsenal against cancer. Understanding radiation doses is crucial for maximizing treatment efficacy. Radiation dose refers to the amount of radiation energy absorbed by tissues and plays a significant role in both the effectiveness and safety of treatment.
The administration of radiation is not straightforward. Every patient's unique characteristics, such as age, overall health, and specific cancer type, influence how radiation is delivered. Thus, comprehending the intricacies behind dose calculations, including fractionation schemes and the balance between therapeutic and adverse effects, is essential.
Recent advancements in precision radiotherapy have transformed conventional approaches. This article seeks to explore these complexities. From the methodologies of dose calculation to ongoing research aimed at optimizing treatment outcomes, we aim to provide valuable insights into understanding the pivotal role of radiation doses in cancer therapy.
Methodology
Overview of Research Methods Used
The research methods utilized in this field are diverse and robust. Studies primarily rely on clinical trials and observational studies. Controlled trials impose specific dosing regimens to measure outcomes in a systematic fashion. Observational studies allow researchers to gather real-world evidence, further enriching the data landscape and informing treatment protocols.
Data Collection Techniques
Data collection for radiation dosing studies involves numerous techniques. Several of these include:
- Patient Surveys: Collecting data on patient experiences regarding side effects and treatment outcomes.
- Clinical Records: Reviewing historical data for patient demographics, imaging outcomes, and follow-up results.
- Radiation Tracking Software: Utilizing advanced software tools to measure and record doses delivered in real-time, enhancing accuracy.
"Radiation dose precision directly impacts treatment success, and its meticulous calculation is vital for improving patient outcomes."
Understanding the methodologies behind radiation dosing is essential for healthcare professionals striving to enhance their practice and optimize therapeutic effectiveness for their patients.
Future Directions
Upcoming Trends in Research
Emerging trends in radiation therapy research focus on integrating technologies such as artificial intelligence. These technologies promise enhanced precision in dose calculations, leading to more personalized treatment plans. New forms of imaging techniques are also being developed, providing better targeting of tumors while sparing surrounding healthy tissue.
Areas Requiring Further Investigation
While significant progress has been made, several areas warrant further investigation:
- Long-term Effects of Dosage: More research is needed on the long-term impacts of radiation doses on aging patients.
- Dose Escalation Studies: Investigating higher doses for specific sub-types of cancers could unveil new therapeutic avenues.
- Biomarkers for Response: Identifying biomarkers that could predict individual responses to radiation therapy could drastically improve treatment personalization.
Prelims to Radiation Therapy in Cancer Treatment
Radiation therapy plays a critical role in modern oncology, providing an effective treatment method for various cancers. Understanding its mechanisms and applications is essential for healthcare professionals, researchers, and students. This article sheds light on the significance of radiation therapy, leading into the specifics of radiation dose, which narrows down treatment efficacy and adverse effects. This introductory section highlights the importance of radiation therapy as a cornerstone in cancer treatment strategies, showcasing its potential benefits and considerations.
Overview of Radiation Therapy
Radiation therapy involves the use of high-energy radiation to damage the DNA inside cancer cells, fundamentally limiting their ability to grow and divide. The technique can be administered in various ways, including external beam radiation, internal radiation (brachytherapy), or systemic radiation. The choice of method depends on the type and location of the cancer, patient health, and treatment goals.
Radiation might be used as a primary treatment or as a complementary option alongside surgery, chemotherapy, and immunotherapy. By stimulating localized treatment while attempting to spare surrounding healthy tissue, radiation therapy remains a popular choice. The key lies in accurately calculating the radiation dose to optimize results while minimizing collateral damage.
History and Evolution of Radiation Treatment
The journey of radiation therapy began in the late 19th century with discoveries by pioneers such as Wilhelm Conrad Roentgen, who discovered X-rays, and Marie Curie, known for her work with radium. The initial applications were rudimentary, focusing primarily on superficial cancers. Over time, advances in technology and understanding of radiation physics led to more targeted approaches. The evolution has seen the development of linear accelerators, imaging technologies, and treatment planning software, all contributing to improved precision in radiation delivery.
Modern developments focus on enhancing dose delivery accuracy and reducing side effects. Innovations such as intensity-modulated radiation therapy (IMRT) and image-guided radiation therapy (IGRT) have further refined the technique. As our understanding of cancer biology and radiobiology progresses, radiation therapy is increasingly integrated into personalized medicine approaches.
Rationale Behind Radiation Use in Cancer
The rationale for using radiation therapy lies in its capacity to effectively target rapidly dividing cells, characteristic of many cancers. Radiation's effects depend on several factors, including the type of cancer, the overall clinical scenario, and individual patient factors. It functions through various mechanisms such as inducing direct DNA damage or generating free radicals that indirectly affect cellular integrity.
Moreover, the strategic use of radiation can be beneficial in multiple scenarios:
- Curative Intent: In some cancers, radiation therapy can clear tumors, providing a chance for long-term survival.
- Adjuvant Treatment: Following surgery, radiation therapy can eliminate residual cancer cells, reducing recurrence risk.
- Palliative Care: For advanced cancers, radiation can alleviate symptoms such as pain, improving the quality of life.
Understanding these facets offers a comprehensive view of how radiation therapy fits into the larger context of cancer management, prompting ongoing discussions on optimizing its use.
Understanding Radiation Dose
Radiation dose is a fundamental concept in cancer treatment, particularly in the realm of radiation therapy. Understanding this topic is crucial not only for clinicians but also for patients and their families. The appropriate assessment of radiation dose can determine the effectiveness of the treatment plan. It is essential to understand how a given dose impacts both cancer cells and surrounding healthy tissues.
In radiation therapy, the aim is to use high-energy radiation to damage the DNA of cancer cells, leading to cell death. However, incorrect dosing can lead to under-treatment, where the tumor fails to receive enough of the radiation needed to shrink or eliminate it. Conversely, over-treatment can result in unnecessary damage to healthy cells, causing severe side effects.
Thus, careful planning and optimal dosing are vital. They ensure that the desired therapeutic effect is achieved while minimizing adverse effects. This section discusses the definitions and the types of radiation doses utilized in medical practice.
Definition of Radiation Dose
Radiation dose is defined as the amount of radiation energy absorbed per unit mass of tissue. It is measured in Gray (Gy), where one Gray is equivalent to the absorption of one joule of radiation energy by one kilogram of matter. Understanding this definition is essential for healthcare providers involved in administering radiation therapy.
The radiation dose can be categorized into different types, which have specific implications in treatment planning. It is not only about how much radiation is given but also about how it is given.
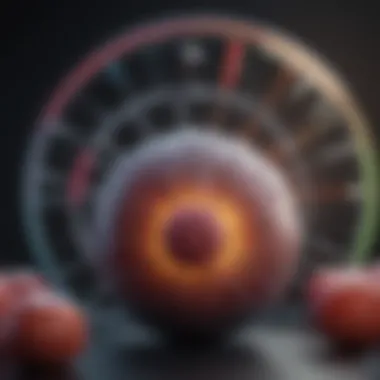
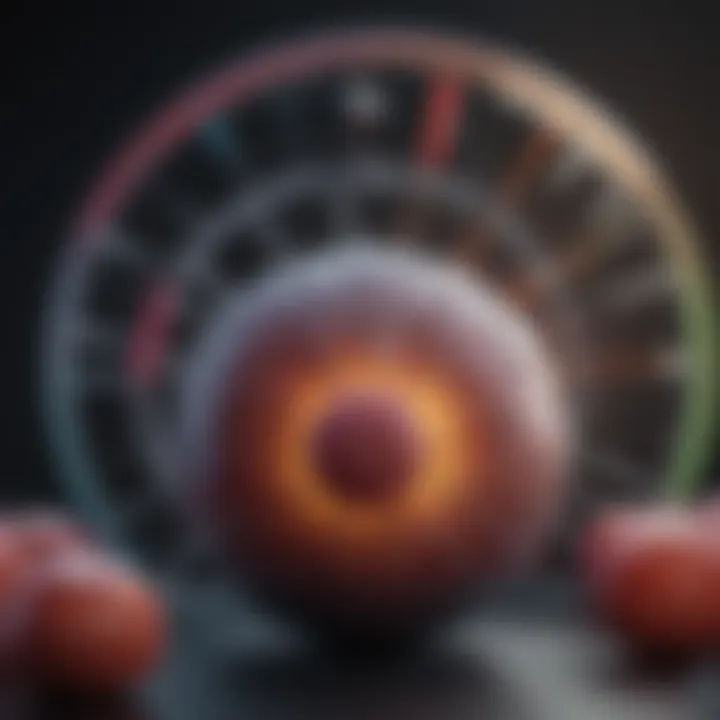
Types of Radiation and Their Doses
There are several types of radiation used in oncology, each with its distinct characteristics and dosing considerations. They can be broadly classified into two categories: ionizing radiation and non-ionizing radiation. Ionizing radiation has enough energy to remove tightly bound electrons from atoms, leading to cellular damage.
Types of Ionizing Radiation
- Photon Radiation: Commonly used in external beam radiation therapy; examples include X-rays and gamma rays. The doses vary depending on factors such as tumor type, size, and location.
- Particle Radiation: Includes protons and electrons. Proton therapy, for instance, is a type of particle radiation that allows for more precise targeting of tumors while sparing nearby healthy tissues. This can lead to different dosage requirements compared to standard X-ray therapy.
It is important to recognize the nuances in radiation doses across these various types. For example, photon therapy may require a higher total dose to achieve the desired effect when compared to proton therapy, which can achieve similar tumor control with a lower overall dose. This variation underscores the necessity of tailoring radiation therapy to the individual patient to maximize efficacy and minimize risks.
Mechanisms of Radiation Therapy
Understanding the mechanisms of radiation therapy is vital in comprehending how radiation works as a treatment for cancer. It enables healthcare professionals to predict treatment outcomes and anticipate patient responses. By grasping these mechanisms, it becomes clearer how radiation can selectively target cancer cells while minimizing damage to normal tissue. This balance is crucial in optimizing patient care and enhancing overall treatment strategies.
How Radiation Affects Cancer Cells
Radiation therapy primarily works by causing damage to the DNA within cancer cells. When cancer cells are exposed to radiation, the energy disrupts their molecular structure and can cause breaks in the DNA strands. This damage can lead to cell death or the inability for the cells to divide and replicate.
The effectiveness of radiation largely depends on the type of radiation as well as the timing and total dose delivered. Different types of radiation include X-rays, gamma rays, and particle beams, each affecting the cells in diverse ways. For instance, high doses of radiation can lead to immediate cell death, while lower doses may cause cells to enter a state of senescence, where they can no longer replicate but are still alive.
Factors such as cell cycle, oxygen levels, and the type of cancer play significant roles in determining how effectively radiation will work against the tumor. Cancer cells that are actively dividing are generally more sensitive to radiation compared to those that are in a resting phase. Additionally, oxygenated tumors tend to be more responsive to radiation, as oxygen enhances the damage caused by radiation.
Differentiating Between Cancer and Healthy Cells
One of the significant challenges in radiation therapy is differentiating between cancerous and healthy cells. While radiation can target cancer cells effectively, it can also impact surrounding healthy tissues. This is where precision in radiation dosing and delivery becomes essential.
Radiation therapy employs various techniques to minimize damage to normal cells. Advanced methods such as Intensity-Modulated Radiation Therapy (IMRT) allow for precise targeting of tumors while sparing healthy tissue. By modulating the dose and angle of radiation beams, oncologists can reduce the impact of radiation on healthy cells.
Effective differentiation is key to achieving balance in therapy. The goal is always to maximize the destructive potential on cancer cells while protecting healthy surroundings.
Additionally, the use of imaging technologies helps in guiding the radiation beams accurately to target tumors. This not only improves the effectiveness of the treatment but also helps in mitigating adverse effects on normal cells.
Radiation Dose Calculation
Radiation dose calculation is critical in maximizing the effectiveness of radiation therapy while minimizing harm to healthy tissues. Accurate dose calculation ensures that the tumor receives the appropriate amount of radiation needed to achieve the desired treatment outcome. Several factors must be carefully considered in this process to tailor the treatment to individual patients. These factors include mathematical models, tumor characteristics, and patient-specific information.
Mathematical Models in Dose Calculation
Mathematical models play a vital role in dose calculation. They help in simulating how radiation interacts with biological tissues, allowing radiation oncologists to predict the distribution of doses delivered within the body. These models account for various parameters, including the type of radiation used and its energy levels, as well as the geometry of both the tumor and surrounding tissues.
One common approach is the use of the linear quadratic model, which helps predict cell survival rates based on dose per fraction. This model is particularly useful in determining the optimal dose for fractionation schemes. Additionally, computational methods like Monte Carlo simulations can provide refined calculations of dose distributions, especially in complex scenarios involving irregular tumor shapes.
Factors Influencing Dose Distribution
Understanding the factors that influence dose distribution is crucial for effective radiation therapy. These include:
Tumor Size
Tumor size is a significant factor in radiation dose calculation. Larger tumors may require higher doses to achieve the same level of effectiveness as smaller ones. The key characteristic here is that as the size increases, the complexity of delivering an adequate dose safely also increases.
For instance, in larger tumors, not only is the central part often less responsive to radiation, but there is also greater surrounding healthy tissue at risk. This makes careful consideration of dose delivery imperative, balancing between sufficient dosage to the tumor and protecting healthy cells.
Location
The location of the tumor has specific implications for radiation dose. Tumors located near critical organs or structures necessitate more precise targeting techniques. This can lead to the choice of advanced technologies like Intensity-Modulated Radiation Therapy (IMRT), which allows more refined dose distributions while protecting adjacent healthy tissues.
A unique challenge with certain locations, such as those close to the spine or lungs, is the risk of collateral damage. Thus, understanding these positions enhances the planning of radiation therapies.
Surrounding Tissue
Surrounding tissue is another crucial element in dose calculation. The extent of dose exposure in healthy tissues around the tumor needs careful evaluation. High radiation doses can cause acute and chronic damage to these tissues, leading to side effects.
The unique feature of considering surrounding tissue is that it helps in determining the safe margin around the tumor operation. Effective dose calculation techniques aim to minimize radiation to these tissues while ensuring that the tumor receives adequate treatment. In this respect, the balance between maximizing tumor control and minimizing normal tissue complications is vital to achieving successful outcomes in radiation therapy.
Fractionation Protocols
In radiation therapy, fractionation protocols play a critical role in maximizing the effectiveness of treatment while minimizing damage to healthy tissues. Fractionation refers to the process of dividing the total dose of radiation into smaller doses, called fractions, delivered over a specified period. This approach allows for healthier tissue to recover between treatments, while continuously attacking the cancer cells. The importance of fractionation protocols cannot be overstated, as they impact not only the efficacy of treatment but also the patient's quality of life during and after therapy.
Concept of Fractionation in Radiation Therapy
Fractionation is based on the biological principle that cancer cells are more sensitive to radiation when they are actively dividing. By delivering radiation in smaller doses, clinicians can exploit this sensitivity. The scheduling of treatment affects tumor response significantly. Each fraction is typically given daily or several times a week, depending on the specific treatment plan. This strategy allows for a higher cumulative dose to be delivered safely, while also giving normal tissues time to recover from radiation exposure.
Types of Fractionation
Understanding the types of fractionation helps in determining the optimal treatment strategy for each patient. The three main types of fractionation are standard fractionation, hypofractionation, and hyperfractionation.
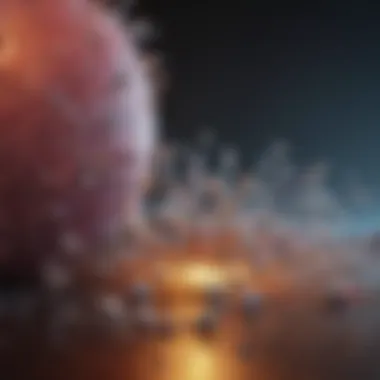
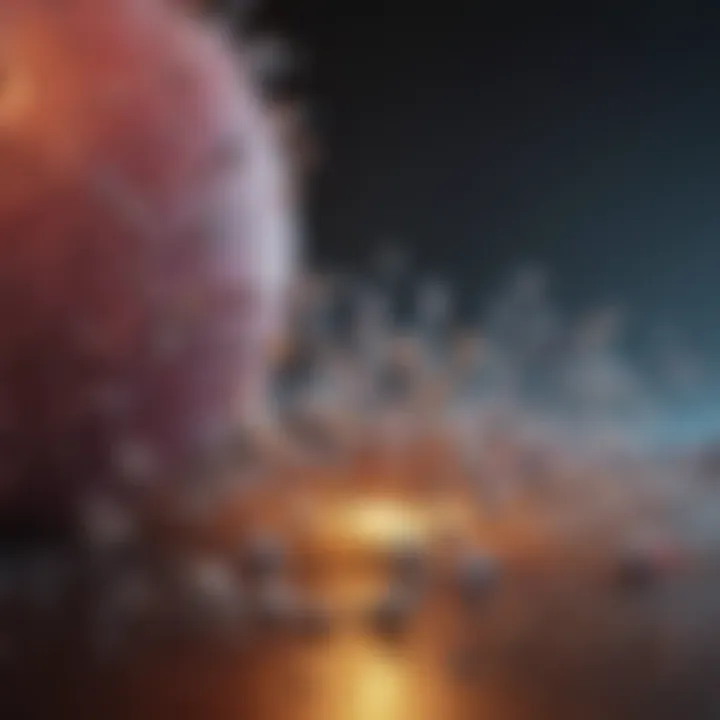
Standard Fractionation
Standard fractionation is the most commonly used protocol in radiation therapy. In this approach, large total doses are divided into many small doses, typically around 1.8 to 2.0 Gray (Gy) per day. This method allows for effective treatment of most common cancers, including breast and prostate cancers. The key characteristic of standard fractionation lies in its time-efficient delivery, making it a popular choice.
The benefits of standard fractionation include:
- Proven effectiveness across various cancer types.
- Routine application in clinical practice.
- Increased normal tissue sparing due to the longer recovery time between fractions.
However, the standard fractionation may not be suitable for all cases. Some tumors might require a different approach to maximize the benefits of radiation therapy.
Hypofractionation
Hypofractionation involves delivering larger doses of radiation per fraction over a shorter time period. For instance, a total dose may be delivered in fewer treatment sessions. This has been increasingly utilized for certain types of cancers, such as localized prostate cancer or early-stage breast cancer. The key characteristic of hypofractionation is that it reduces the overall treatment time, which can benefit patient convenience and lessen the burden of daily visits.
Advantages of hypofractionation include:
- Fewer treatment sessions, resulting in less disruption.
- Potentially improved tumor control due to the higher dose delivered in each session.
Nonetheless, hypofractionation is also associated with increased risk of toxicity to healthy tissues. This requires careful selection of patients and thorough planning.
Hyperfractionation
Hyperfractionation entails administering smaller doses of radiation multiple times in a day. This approach is directed primarily at specific tumor types that are known to respond better to the increased radiation dose. The key characteristic of hyperfractionation is the elevated total dose delivered in a shorter duration, which may help in overcoming tumor repopulation between treatments.
The unique feature of hyperfractionation provides:
- Enhanced tumor control in highly aggressive cancers.
- Reduced likelihood of tumor regrowth during treatment.
However, it mandates meticulous planning and monitoring due to possible cumulative effects on normal tissues.
Effective fractionation protocols are fundamental to optimizing radiation therapy outcomes, balancing efficacy whilst minimizing adverse effects.
In summary, the choice of fractionation protocol directly influences cancer treatment success. Different strategies like standard fractionation, hypofractionation, and hyperfractionation offer personalized approaches based on tumor characteristics and patient needs.
Personalization of Radiation Dose
Personalization of radiation dose in cancer treatment is crucial. Every patient is unique, with different tumor types, locations, and biological responses. Tailoring radiation doses to meet individual needs can significantly improve effectiveness while minimizing unnecessary harm to healthy tissues. This approach is increasingly vital as research stretches the boundaries of conventional methods.
Tailoring Dose to Individual Patient Needs
To ensure the most effective treatment, radiation oncologists consider numerous patient-specific factors. These include patient age, overall health, cancer stage, and treatment history. By assessing such elements, clinicians can determine the appropriate dose necessary for maximum tumor control.
*Benefits of individualizing radiation dose:
- Enhanced treatment efficacy: Aligning the dose with patient parameters can increase the chances of killing cancer cells while respecting normal tissues.
- Reduced side effects: Personalization often allows for minimized adverse effects, improving the patient's quality of life during and after treatment.
- Optimized treatment plans: Each patient may respond differently to radiation doses; by tailoring these plans based on personal needs, outcomes can be maximized. The medical community frequently employs advanced imaging technologies, such as MRI and CT scans. These tools help visualize tumors and surrounding organs accurately. As a result, oncologists can refine their targeted radiation approaches, ensuring that the prescribed dose matches the patient's specific physiological makeup.
Role of Genetic and Biological Markers
Genetic and biological markers influence how patients respond to radiation therapy. Tumors have distinct characteristics that might make them more or less susceptible to radiation. For instance, certain genetic mutations can indicate a tumor's likelihood of responding positively to a particular radiation dose.
Understanding these markers can:
- Direct treatment decisions: Genetic testing can indicate which patients might benefit from higher doses versus those more suited for lower doses.
- Facilitate research into precision medicine: Ongoing research continually uncovers new relationships between genetic factors and radiation response, paving the way for more effective treatment options.
- Aid in eligibility for clinical trials: Patients with specific markers might qualify for novel therapies targeting their unique backgrounds.
"Adapting radiation therapy to each patient's genetic profile is not just a trend; it's becoming an essential component of effective oncology."
The integration of molecular biology with radiation therapy is promising. By focusing on these elements, clinicians can refine radiotherapy protocols. Personalization based on genetic and biological markers may eventually lead to better overall outcomes in cancer treatment.
Adverse Effects of Radiation Therapy
Understanding the adverse effects of radiation therapy is crucial for both patients and healthcare providers in managing cancer treatment outcomes. While radiation therapy aims to eradicate cancer cells, it can also harm healthy tissues. Identifying and mitigating these adverse effects is essential in optimizing patient care and improving overall treatment efficacy. Awareness of potential side effects allows for better planning and supportive care, enhancing the quality of life for patients undergoing treatment.
Short-Term Effects
Short-term effects of radiation therapy often manifest within days or weeks of treatment. These effects can vary based on the radiation site, dosage, and individual patient response. Common short-term effects include:
- Fatigue: A prevalent symptom experienced by many patients after treatment. Fatigue may persist throughout the radiation course and often warrants management strategies.
- Skin Reactions: Irritation, redness, or peeling in the treated area is typical. Patients need to follow skincare instructions provided by their healthcare team to minimize discomfort.
- Nausea: For patients receiving radiation to the abdominal area, nausea and vomiting may occur, which can impact nutrition and hydration.
- Changes in Appetite: Some patients may experience a loss of appetite, making nutritional support important during treatment.
Proper monitoring and communication about these effects can empower patients to manage symptoms effectively. Healthcare teams should educate patients on recognizing symptoms early and provide supportive interventions.
Long-Term Consequences
Long-term consequences of radiation therapy can emerge months or years after treatment. These effects can significantly impact patients' quality of life and may vary considerably among individuals. Common long-term effects include:
- Secondary Cancers: One of the more serious long-term risks is the potential development of secondary malignancies due to previous radiation exposure.
- Changes in Organ Function: Radiation can affect the function of nearby organs, especially if the treatment area is in close proximity to critical structures. Effects might include cardiopulmonary issues after chest radiation or gastrointestinal problems after abdominal radiation.
- Fibrosis: Scar tissue formation in the treated area may lead to functional impairments or pain, which can be detrimental to a patient's well-being.
- Psychosocial effects: The experience of surviving cancer treatment can leave psychological scars, including anxiety or depression. Addressing these issues through counseling or support groups is beneficial for many patients.
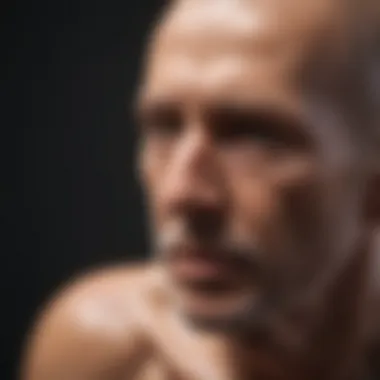
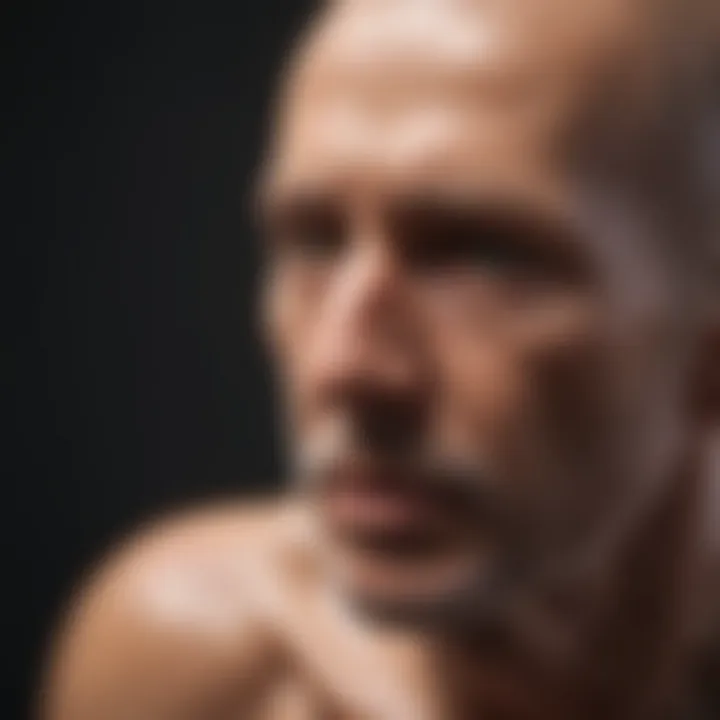
Patients must be counseled regarding potential long-term risks before initiating radiation therapy. This ongoing dialogue supports informed decision-making and encourages adherence to follow-up care protocols. Effective management strategies can mitigate these long-term consequences, ensuring that cancer survivors maintain a quality lifestyle.
"Awareness and education about both short-term and long-term effects help patients prepare for and navigate the complexities of cancer treatment with greater confidence."
Current Research and Innovations
In the realm of radiation therapy, ongoing research and innovative technologies are crucial. They aim to enhance treatment effectiveness, reduce side effects, and improve patient outcomes. Understanding these advancements can provide insight into the future of cancer treatment. New technologies often focus on achieving precision in delivering radiation doses. This improves targeting of tumors while sparing healthy tissue. As a result, cancer treatments can be less damaging and more tolerable for patients.
Emerging Technologies in Radiation Therapy
Proton Therapy
Proton therapy represents a significant shift in how radiation can be administered to cancer patients. Instead of using X-rays, proton therapy utilizes protons to treat tumors. One key characteristic of this method is its ability to precisely deliver radiation to the tumor's site, minimizing exposure to surrounding healthy tissue. This targeted approach reduces the risk of damage to organs near the tumor.
Proton therapy is particularly beneficial for treating pediatric patients. Their developing bodies are more sensitive to radiation, so precision is critical in their treatment. However, proton therapy can be more expensive than traditional therapies. It also requires specialized equipment and facilities.
Stereotactic Body Radiation Therapy
Stereotactic Body Radiation Therapy, or SBRT, offers another innovative approach. It delivers large doses of radiation to small, well-defined tumors. Personalized treatment plans enable high precision, allowing for fewer sessions while maintaining treatment effectiveness. A key characteristic of SBRT is its ability to treat tumors in challenging locations, such as the lungs or liver.
This method typically requires advanced imaging techniques to guide the treatment. Its unique feature is the rapid dose delivery over a limited number of sessions, which can improve patient convenience. However, SBRT may not be suitable for all types of tumors, especially large ones, as the concentration of radiation may increase the risk of damage to nearby healthy tissue.
Adaptive Radiation Therapy
Adaptive radiation therapy represents the next step toward personalized cancer treatment. It focuses on adjusting treatment plans based on the patient's changing anatomy or tumor response during the treatment course. This flexibility is crucial as tumors can vary in size and position over time. Adaptive radiation therapy ensures that the radiation dose remains optimal throughout treatment. It allows oncologists to respond to individual patient needs effectively.
This method can enhance the therapeutic ratio of radiation therapy, as it aims to maximize the dose to the tumor while minimizing exposure to healthy tissue. Although still in developmental stages, adaptive radiation therapy demonstrates promising potential. It proves to be an essential consideration in the future landscape of radiation oncology.
Clinical Guidelines and Protocols
Understanding clinical guidelines and protocols is vital in optimizing the effectiveness of radiation therapy in cancer treatment. These guidelines provide a structured approach that ensures patients receive the most appropriate doses tailored to their specific circumstances. They encompass regulatory recommendations, best practices, and evidence-based strategies aimed at minimizing risk while maximizing therapeutic outcomes. With the continuous evolution of cancer treatments and technologies, adhering to established protocols helps clinicians navigate complex treatment plans and enhances patient safety and efficacy.
Established Guidelines for Radiation Dose
Established guidelines for radiation doses provide a framework rooted in extensive clinical research and trials. Organizations such as the American College of Radiology (ACR) and the Radiological Society of North America (RSNA) publish recommendations that healthcare providers use to standardize doses across various treatment regimens. These guidelines refer to dose limits, treatment volume definitions, and patient-specific variations necessary for adjusting doses effectively.
Moreover, guidelines outline the importance of utilizing advanced imaging techniques to enhance accuracy in targeting and delivering radiation. Precise localization minimizes exposure to surrounding healthy tissues, mitigating adverse effects and improving outcomes. It is crucial for practitioners to regularly consult and update their knowledge of these guidelines, as they are periodically revised based on new findings.
Protocols for Different Cancer Types
Protocols for different cancer types reflect the unique biological and anatomical considerations of each malignancy. Each type of cancer responds differently to radiation therapy, necessitating tailored dosage and treatment plans. For instance:
- Breast Cancer: Protocols may dictate a combination of whole-breast irradiation and boost doses to the tumor bed, often following lumpectomy.
- Lung Cancer: Doses may vary between small cell lung cancer and non-small cell lung cancer. Stereotactic Body Radiation Therapy (SBRT) is a common approach for localized tumors, given its precision.
- Prostate Cancer: Treatment protocols often involve hypofractionation, where a higher dose per fraction is delivered, reducing total treatment days.
Adhering to specific protocols allows for an evidence-based approach to therapy, ensuring that patients receive optimal doses that consider tumor biology and patient health. Clinicians must remain knowledgeable about ongoing research in each cancer modality, as new protocols regularly emerge from clinical trials aiming to enhance treatment efficacy and safety.
The Future of Radiation Therapy
The future of radiation therapy holds significant promise in transforming cancer treatment paradigms. As technology evolves, the field is increasingly leaning towards precision medicine, which focuses on tailored treatments aimed at individual patient needs. This shift allows oncologists to optimize radiation doses and minimize adverse effects, marking a notable improvement in patient care. Developing and implementing advanced techniques is critical for better efficacy in treatment protocols, thereby leading to more favorable patient outcomes.
Towards Precision Medicine
Precision medicine signifies a tailored approach to medical treatment, with the primary objective of personalizing care based on an individual's unique genetic makeup, environment, and lifestyle choices. In the realm of radiation therapy, this method enhances the potential for effective treatment by ensuring that the dosage and method are specifically suited to the patient's cancer type and its molecular characteristics.
- Targeted Therapies: The rise of targeted therapies allows for specific cancer cells to be targeted while sparing healthy tissue. This means higher doses can be safely administered, improving the likelihood of success without significant side effects.
- Genomic Profiling: Genomic profiling is playing a vital role in treatment customization. By understanding the genetic mutations present in a patient's tumor, clinicians can select the most effective treatment route. It fosters a more nuanced understanding of how different tumors respond to radiation.
- Adaptive Treatment Plans: The implementation of adaptive treatment plans is becoming more common. As the patient undergoes therapy, real-time monitoring assists in adjusting the radiation dose according to the tumor's response, improving both effectiveness and safety.
Incorporating these techniques denotes a clear trend towards refining how radiation therapy is delivered. It assures that the future focuses not only on treating cancer but doing so with a high degree of personalization that aligns with each patient's biological makeup.
Integration with Other Treatment Modalities
The integration of radiation therapy with other treatment modalities signifies a pivotal shift in oncology. Combining different approaches enhances cancer treatment efficacy and mitigates the limitations inherent in any single modality.
- Chemotherapy and Radiation: There is a growing trend to combine chemotherapy with radiation. This dual approach capitalizes on the synergistic effects where chemotherapy may sensitize cancer cells to radiation, allowing lower doses to achieve the same effect.
- Immunotherapy and Radiation: Integrating immunotherapy with radiation therapy is proving beneficial. Radiation can enhance immune responses against tumors, making this combination attractive for treating resistant types of cancer.
- Surgical Intervention: In some cases, radiation can be administered pre-operatively or post-operatively to improve surgical outcomes. This integration is crucial for managing tumor size and preventing recurrence, leading to improved long-term survival rates.
Furthermore, as research progresses, innovative combinations of therapies could emerge, optimizing patient outcomes even further. A holistic approach that considers all potential therapies allows for strategic decisions tailored uniquely to the patient.
"The fusion of radiation therapy with other modalities presents a forward-thinking structure to cancer treatment."
Ending
Summarizing Key Insights
The complexities of radiation dosing are deeply interconnected with treatment outcomes. Key points discussed include:
- Dose Calculation Methods: The mathematical models that clinicians employ are essential for determining safe, effective doses tailored to individual patients.
- Fractionation Protocols: Different approaches to fractionation, such as standard, hypofractionation, and hyperfractionation, allow oncologists to personalize treatments, potentially improving efficacy.
- Adverse Effects: The balance between therapeutic benefits and the risk of adverse effects is crucial for treatment planning, necessitating ongoing assessment of patient responses.
- Emerging Technologies: Innovations such as proton therapy and adaptive radiation therapy reflect how advancements are enhancing the precision of treatment, leading to better outcomes with reduced side effects.
Outlook for Radiation Therapy in Oncology
Looking to the future, the role of radiation therapy in oncology is evolving. Several trends are noteworthy:
- Towards Precision Medicine: As research advances, there is a growing focus on personalized treatment plans that consider genetic and biological factors associated with tumors. This shift aims to tailor radiation doses more effectively, improving outcomes.
- Integration with Other Modalities: The intersection of radiation therapy with immunotherapy, targeted therapies, and surgical methods creates a multi-faceted approach to cancer treatment. This integrated strategy enhances overall patient management and maximizes therapeutic efficacy.