Understanding mRNA: Structure, Function, and Significance
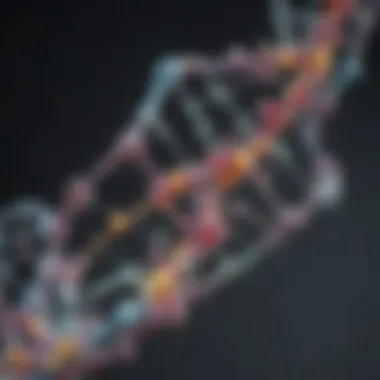
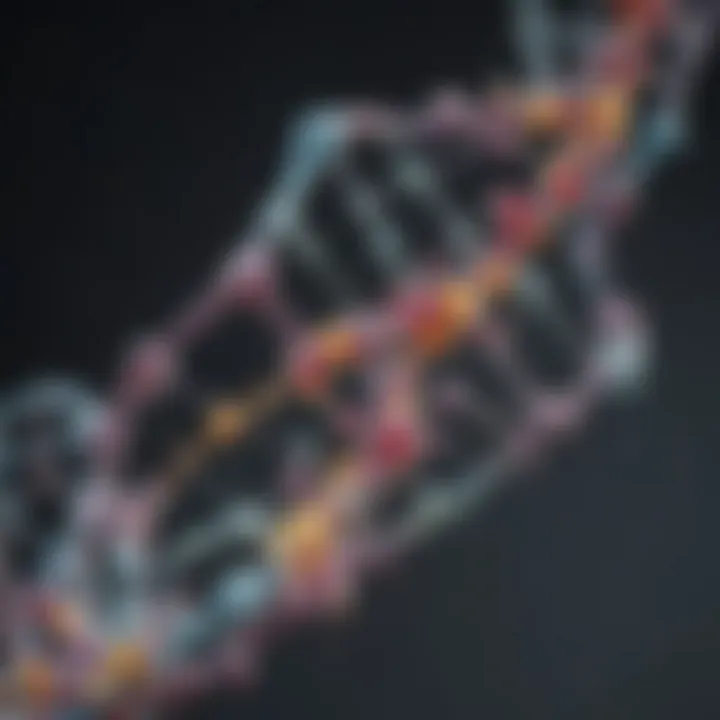
Intro
Messenger RNA, commonly known as mRNA, plays a crucial role in the process of gene expression. It serves as a vital intermediary between the genetic code for proteins contained in DNA and the functional proteins themselves. Understanding mRNA is essential for those studying molecular biology, genetics, or related fields.
As we delve into this article, we will explore mRNA's structural characteristics, its various functions within the cell, and its significance in the fields of biotechnology and medicine. In the era of rapid advancements in genetic therapies and vaccine development, an in-depth comprehension of mRNA is increasingly relevant.
This article will unravel key points such as:
- The molecular structure of mRNA and its components.
- The process of transcription, where mRNA is synthesized from DNA.
- Translation, the mechanism by which mRNA is used to assemble proteins.
- The importance of post-transcriptional modifications.
- The role of mRNA in contemporary medicine, particularly in relation to vaccines and therapeutics.
Through this comprehensive analysis, we aim not only to educate but also to foster a deeper understanding of the myriad functions of mRNA in biological systems.
Intro to mRNA
Messenger RNA, commonly referred to as mRNA, is a crucial molecule in the landscape of molecular biology. Understanding mRNA provides insights into fundamental biological processes, especially gene expression and protein synthesis. This section lays the groundwork for exploring mRNA in detail. The significance of mRNA cannot be overstated, as it serves as the intermediary between DNA and protein, translating genetic information into functional units in the cell.
The role of mRNA encompasses various critical functions. It carries the genetic blueprint from DNA in the nucleus, facilitating the process of translation in the cytoplasm. As such, it acts as a messenger, conveying essential instructions for protein synthesis. Moreover, mRNA is involved in intricate regulatory mechanisms that influence gene expression. Understanding these roles and the structure of mRNA is central to appreciating its importance in both cellular function and emerging biotechnologies.
Defining mRNA
Messenger RNA is a type of RNA that serves as a template for protein synthesis. It is synthesized from a DNA template during the process known as transcription. The structure of mRNA consists of a sequence of ribonucleotides, each composed of a ribose sugar, a phosphate group, and a nitrogenous base.
Different from DNA, mRNA is usually single-stranded and features uracil instead of thymine as one of its nucleobases. The sequence of bases in mRNA dictates the order of amino acids in a protein, making it essential for translating genetic information into functional proteins.
Furthermore, mRNA is characterized by modifications. The addition of a 5' cap and a poly-A tail at the 3' end enhances its stability and regulation during translation. Such features distinguish mRNA from other types of RNA, illustrating its unique role in cellular processes.
Historical Context
The understanding of mRNA has evolved significantly over the last several decades. Initial discoveries date back to the 1960s when researchers sought to elucidate the mechanisms of protein synthesis in cells. The concept of mRNA emerged as scientists like François Jacob and Jacques Monod in France proposed the existence of an intermediary molecule that conveys genetic information from DNA to ribosomes, the sites of protein synthesis.
Through subsequent research, the pivotal role of mRNA became clearer. By the 1970s, the process of transcription was thoroughly characterized, allowing for greater understanding of how mRNA is synthesized and utilized within cells. The development of biochemical techniques paved the way for further discoveries about mRNA's structure and function, leading to new insights into gene regulation and expression.
The elucidation of mRNA has not only changed our understanding of genetics but has also set the foundation for advancements in molecular biology and biotechnology.
As we progress through this article, we will dissect the complex structure and functions of mRNA, shining a light on its applications in medicine and biotechnology.
The Molecular Structure of mRNA
The structure of messenger RNA (mRNA) is critical for its role in the process of gene expression and ultimately in protein synthesis. Understanding its molecular configuration provides insights into its functionality. mRNA is not just a linear sequence; it possesses unique components that govern its stability, translation efficiency, and how it interacts within the cell.
Components of mRNA
Each component of mRNA plays a specific role in its structure and function, influencing its overall behavior in the cellular environment.
Ribonucleotides
Ribonucleotides are the building blocks of mRNA, consisting of a ribose sugar, a phosphate group, and one of four nitrogenous bases: adenine, guanine, cytosine, or uracil. Each ribonucleotide links together to form the mRNA strand. The specificity of the nitrogenous base encodes genetic information crucial for protein synthesis. Their flexibility and ability to form hydrogen bonds are key characteristics that allow ribonucleotides to provide both stability and adaptability in different cellular conditions. This adaptability makes ribonucleotides a vital choice for genetic information transfer.
Phosphate Backbone
The phosphate backbone provides structural integrity to the mRNA molecule. It consists of alternating phosphate and sugar groups, forming a robust chain that resists degradation. The primary characteristic of the phosphate backbone is its negative charge, which influences the interaction with ribosomes and other proteins. This charged nature enhances the solubility of mRNA in the cellular environment, making it a beneficial component for its functionality. However, its stability can be a double-edged sword; under certain conditions, changes in the environment can lead to hydrolysis of the backbone.
' Cap Structure
The 5' cap is a modified guanine nucleotide added to the beginning of the mRNA strand. Its role is crucial for mRNA stability and protection from exonucleases. Furthermore, the 5' cap is essential for the initiation of translation. The unique feature of the cap is that it enhances ribosome binding, facilitating an efficient translation process. This structure is favored because it significantly improves mRNA lifetime and efficiency in protein synthesis, although improper capping can lead to degradation.
Poly-A Tail
The poly-A tail is a stretch of adenine nucleotides added to the 3' end of the mRNA molecule. This tail has several key functions, including enhancing mRNA stability and regulating its transport out of the nucleus. The poly-A tail also aids in the translation efficiency by promoting ribosome interaction. Its unique feature is its length, which varies considerably across different mRNAs, reflecting the level of control over gene expression. However, mRNAs with very short poly-A tails may have reduced stability and translation capacity, illustrating the tail's critical role in cellular processes.
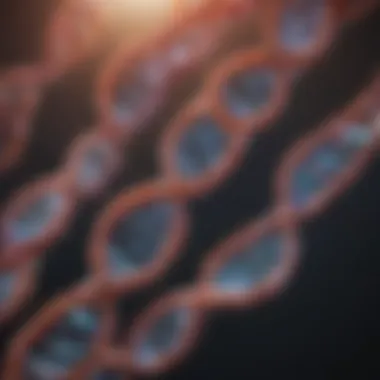
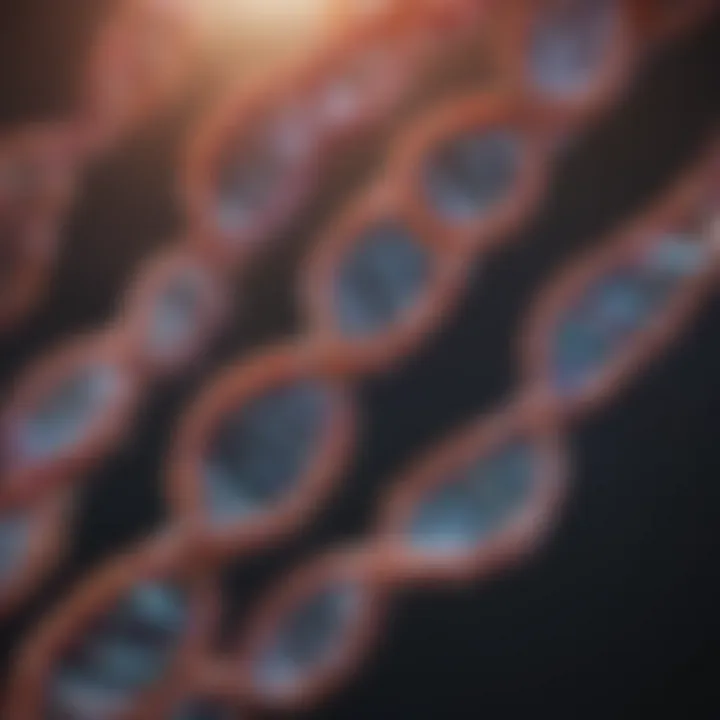
Structural Variations
mRNA can also exhibit structural variations that affect its stability and function, allowing for greater flexibility in the regulation of gene expression.
Splicing Variants
Splicing variants occur through alternative splicing, where pre-mRNA is processed to remove introns and join exons in various combinations. This mechanism yields different mature mRNA molecules from a single gene, enhancing protein diversity. The characteristic of splicing variants lies in their ability to produce multiple proteins from a single genetic strand, making it a highly beneficial process for cellular adaptability. However, this complexity might introduce challenges in maintaining proper regulatory mechanisms, potentially leading to diseases if misregulated.
Alternative Polyadenylation
Alternative polyadenylation refers to the generation of mRNA isoforms with distinct 3' ends due to variations in polyadenylation signals. This results in different lengths of poly-A tails, potentially influencing the stability and translation of the mRNA. The primary characteristic of this variation is its ability to regulate gene expression at multiple levels. It offers flexibility and control in gene regulation, which is critical in developmental processes and responses to cellular signals. However, the consequences of misregulated alternative polyadenylation may lead to pathological conditions, showcasing its significance in precision genetics.
Synthesis of mRNA
The synthesis of messenger RNA (mRNA) is a critical process in the expression of genes. It serves as the intermediary between DNA and proteins, embodying not just the information encoded in DNA but also playing a key role in regulating cellular activities. The mRNA synthesis process, including transcription and the role of RNA polymerase, underscores the intricate mechanisms that facilitate gene expression. Understanding this topic is essential for grasping how proteins are ultimately synthesized, which is fundamental to all biological functions.
Transcription Process
Transcription is the first step in mRNA synthesis. This process occurs within the cell nucleus, where the DNA resides. During transcription, the DNA double helix unwinds to expose a segment of the gene that is to be transcribed. The enzyme RNA polymerase binds to a specific region called the promoter on the DNA strand. This binding is crucial as it determines where transcription will initiate.
Once attached, RNA polymerase moves along the DNA template strand, synthesizing a complementary strand of RNA. Thymine in the DNA is replaced by uracil in the RNA. This synthesis process continues until RNA polymerase encounters a termination sequence, signaling the end of transcription. The resulting mRNA strand is a transcript of the gene that can move to the cytoplasm for translation into protein.
Role of RNA Polymerase
RNA polymerase is an essential enzyme in the transcription process, with several key functions. There are different types of RNA polymerase, but RNA polymerase II is primarily responsible for synthesizing messenger RNA in eukaryotes.
• Binding: RNA polymerase locates the promoter region and binds to the DNA, initiating the transcription process.
• Synthesis: It catalyzes the formation of phosphodiester bonds between ribonucleotides, growing the RNA chain.
• Editing: After synthesis, RNA polymerase checks for errors in the new RNA strand.
RNA polymerase is not only a facilitator but also a regulator of gene expression. It can respond to various signals within the cell, adjusting the level of mRNA produced based on the needs of the cell. This adjustability makes RNA polymerase vital for maintaining cellular homeostasis and responding to environmental changes.
"RNA polymerase serves as the gatekeeper of gene expression, dictating when and how much mRNA is synthesized."
In summary, the synthesis of mRNA through the transcription process and the pivotal role of RNA polymerase are foundational components of gene expression. These mechanisms not only drive the production of proteins essential for cellular function but also play a significant role in the regulation and adaptation of biological systems.
mRNA in Protein Synthesis
The role of messenger RNA (mRNA) in protein synthesis is critical and cannot be overstated. mRNA carries the genetic information from DNA, which resides in the nucleus, to the ribosomes in the cytoplasm where proteins are synthesized. This process is fundamental for cellular function, as proteins are involved in almost every biological process.
Translation Mechanism
Translation is the process by which the information encoded in mRNA is used to produce proteins. This mechanism occurs in three main steps: initiation, elongation, and termination.
- Initiation: The small ribosomal subunit binds to the 5' cap of the mRNA. This is followed by the assembly of the complete ribosome, which includes the large subunit.
- Elongation: tRNA molecules, which carry specific amino acids, read the codons on the mRNA. Each tRNA matches with the corresponding codon and adds its amino acid to the growing polypeptide chain. This part of translation continues until a stop codon is reached.
- Termination: Upon reaching a stop codon, the translation process halts, and the newly formed polypeptide chain is released. The ribosomal subunits then disassemble, ready to initiate another round of translation.
This process demonstrates how mRNA acts as a blueprint for protein synthesis, converting the genetic code into functional products. It is the interface through which the cell translates genetic information into actions.
Ribosome Structure
Ribosomes themselves are complex molecular machines responsible for synthesizing proteins by translating mRNA. They consist of ribosomal RNA (rRNA) and proteins, forming two subunits: a small subunit and a large subunit.
- Small Subunit: This part is essential for reading the mRNA. Its structure ensures that the correct tRNA matches with the mRNA codons, allowing for accurate translation.
- Large Subunit: This component is responsible for forming peptide bonds between amino acids. It has active sites that catalyze the reaction between tRNA and amino acids, facilitating protein construction.
Ribosomes can be free-floating in the cytoplasm or attached to the endoplasmic reticulum, influencing whether the synthesized proteins are secreted or used within the cell.
The dynamic nature of ribosomes allows cells to efficiently produce proteins required for various functions and responses to environmental changes.
In summary, mRNA is indispensable in protein synthesis, operating through a well-orchestrated mechanism of translation. It interfaces closely with ribosomes to ensure the fidelity and efficiency of protein production, thus playing a key role in maintaining cellular vitality and functionality.
Post-Transcriptional Modifications
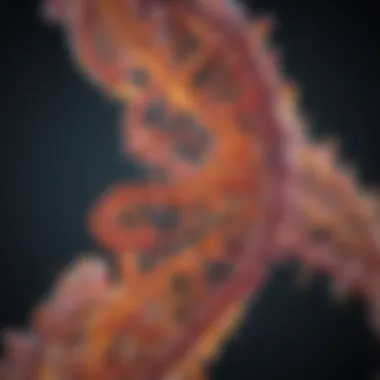
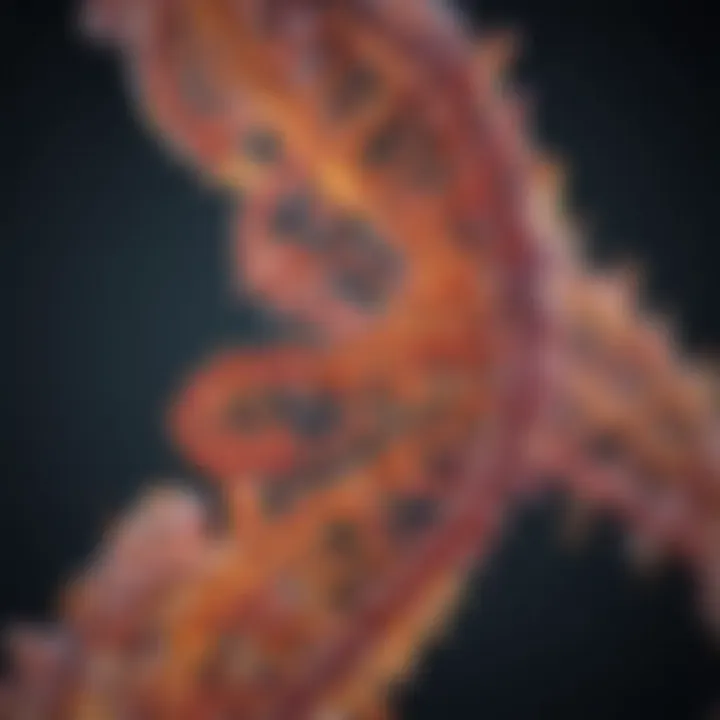
Post-transcriptional modifications are critical processes that occur after the synthesis of mRNA from DNA. These modifications significantly influence the stability, localization, and translational efficiency of mRNA molecules. They enable the cell to fine-tune gene expression in response to various internal and external signals. Understanding these modifications is essential for appreciating how mRNA plays its pivotal role in cellular function and overall gene regulation.
Capping
The capping of mRNA is a vital modification that involves the addition of a 7-methylguanylate cap to the 5' end of the mRNA transcript. This cap protects the mRNA from degradation by exonucleases and assists in the initiation of translation. The cap structure is essential for the recruitment of ribosomes, the cellular machinery responsible for protein synthesis. Additionally, it facilitates mRNA export from the nucleus to the cytoplasm. The presence of a cap enhances the stability of the mRNA molecule, ensuring that it can be translated effectively into proteins.
Editing and Splicing
The editing and splicing of mRNA are crucial in generating mature mRNA capable of directing protein synthesis. Editing can involve changes to specific nucleotides within the mRNA sequence, altering the coding potential of the transcript. This means that a single mRNA molecule can produce different protein products through alternative splicing. Splicing removes non-coding regions known as introns and joins coding regions called exons. This selective inclusion of exons allows for the generation of multiple protein isoforms from a single gene, providing a mechanism for increasing the diversity of the proteome.
The flexibility offered by editing and splicing emphasizes the complexity of gene regulation at the mRNA level.
These post-transcriptional modifications not only influence which proteins are produced but also how and when they are produced. Understanding these processes is essential for researchers aiming to manipulate gene expression in therapeutic settings or for biotechnological applications.
Regulation of mRNA Function
Regulation of mRNA function is a critical area of study in molecular biology. It encompasses the various mechanisms that control mRNA levels in the cell, directly impacting gene expression. Proper regulation is essential for cellular homeostasis, differentiation, and response to environmental changes. The intricate process ensures that genes are expressed at the right time, place, and amount, which is vital for organismal development and function.
Gene Expression Control
Gene expression control refers to the cellular processes that determine when and how much of a gene product is produced. mRNA plays a key role in this system. Various mechanisms come into play in this regulation, such as transcription regulation, mRNA stability, and translational control.
- Transcription Regulation: This is the first step where signals or stimuli can increase or decrease the creation of mRNA from DNA. Transcription factors bind to specific DNA sequences to promote or inhibit transcription.
- mRNA Stability: The half-life of mRNA in the cytoplasm influences how much protein can be produced. Specific sequences in the mRNA can signal the cell to degrade the RNA, effectively controlling its presence.
- Translational Control: This encompasses how efficiently an mRNA is translated into protein. Various factors, including feedback mechanisms, can alter the efficiency of the translation process.
RNA Interference
RNA interference, or RNAi, is another significant regulatory mechanism. This biological process regulates gene expression by using small RNA molecules. These molecules can silence specific mRNAs, thus preventing their translation into protein. RNA interference operates through the following:
- Small Interfering RNAs (siRNAs): These are double-stranded RNA molecules that can bind to mRNAs, leading to their degradation.
- MicroRNAs (miRNAs): These are small, single-stranded RNA molecules that usually bind to the 3’ untranslated region of target mRNAs. They often inhibit translation rather than causing degradation.
- Dicer and RISC: These are key components in the RNAi pathway. Dicer processes long double-stranded RNAs into small fragments. RISC (RNA-induced silencing complex) then binds to these fragments to exert their silencing effects.
RNA interference has become a focus in therapeutic strategies, including the development of treatments for various diseases.
In summary, regulation of mRNA function is fundamental to cellular life. Understanding these processes helps in grasping how cells adapt and respond to their environment. It also has implications in biotechnology and medicine, making it a vital area of ongoing research for future innovations.
mRNA and Cellular Localization
The localization of mRNA within the cell is crucial for its functional efficacy. Understanding how mRNA is transported and localized provides insight into gene expression regulation and the overall cellular dynamics. Cellular localization impacts protein synthesis, ensuring that mRNA is present at the right place and time, thereby influencing the efficiency of translation. When mRNA reaches specific locales, it can engage the necessary machinery for protein synthesis, giving rise to localized protein production which is vital for cellular function.
Transport Mechanisms
Transport mechanisms for mRNA involve several pathways and molecules that facilitate the movement of mRNA from the nucleus to cytoplasmic sites where translation occurs. After synthesis in the nucleus, mRNA undergoes several modifications before it is exported. The nuclear pore complexes play a significant role in this transport, serving as gateways that regulate the movement of mRNA out of the nucleus. Specific proteins, such as exportins, bind to mRNA and assist in its passage through these complexes. This transport is selective and requires energy, underscoring the importance of ensuring that only properly processed mRNA is translated.
Key factors that influence mRNA transport include:
- The presence of the 5' cap and poly-A tail, which protect the mRNA and are recognized by transport proteins.
- Interaction with RNA-binding proteins that guide mRNA to specific sites within the cell.
- Involvement of motor proteins, such as kinesins, which move mRNA along the cytoskeletal tracks to designated sites.
Translation Site Localization
Once mRNA reaches the cytoplasm, localization becomes critical for translation. Different mRNAs find their way to specific regions within the cell based on signal sequences or structures that dictate their destination. For example, some mRNA molecules are directed to the endoplasmic reticulum (ER) where they are translated into secretory proteins. Others may be localized to dendrites in neurons, which is essential for synaptic protein synthesis.
Factors affecting translation site localization include:
- Localization signals within the mRNA sequence that direct it to particular cellular compartments.
- Interaction with protein complexes, such as the RNP (ribonucleoprotein) complexes, that facilitate the anchoring of mRNA to specific sites.
- Environmental cues that influence mRNA distribution and its subsequent translation.
Proper cellular localization of mRNA ensures efficient protein synthesis, vital for maintaining cellular functions and responses to changing conditions.
Overall, understanding mRNA localization in cells enhances the comprehension of how proteins are produced in specific contexts, providing a clearer picture of cellular behavior and the regulation of gene expression.
mRNA in Biotechnology
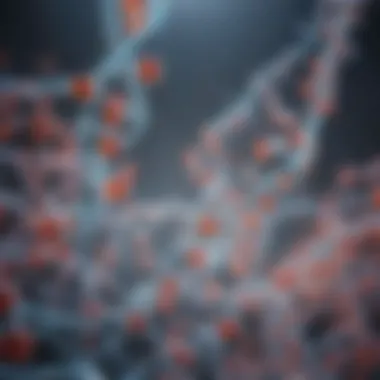
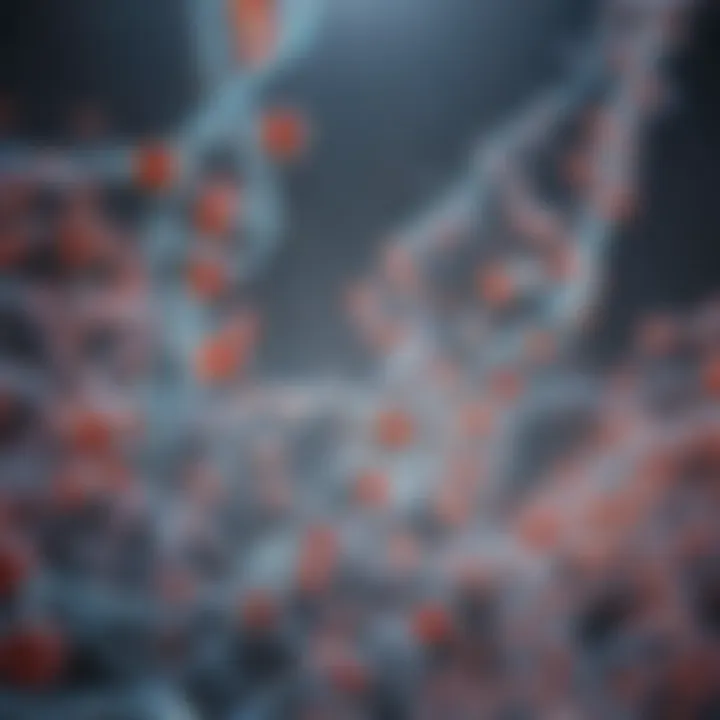
The role of messenger RNA in biotechnology is increasingly significant. As our understanding of mRNA has evolved, its applications have expanded dramatically. Researchers are utilizing mRNA for various innovative purposes, primarily in the fields of medicine and therapeutic interventions. This section explores two predominant areas: mRNA vaccines and gene therapy applications. Each area showcases the potential benefits and some challenges associated with mRNA use in modern science.
mRNA Vaccines
mRNA vaccines represent a breakthrough in immunization technology. Unlike traditional vaccines that often use weakened or inactive pathogens, mRNA vaccines deliver a piece of genetic code that instructs cells to produce a harmless protein unique to a virus. This prompts the immune system to recognize and mount a defense against the actual virus if encountered.
The success of the Pfizer-BioNTech and Moderna vaccines against COVID-19 exemplifies the power of mRNA technology. The rapid development of these vaccines demonstrated a new paradigm for vaccine creation. They were designed and produced within months, showcasing how effective mRNA can be when addressing emerging health threats. Key advantages of mRNA vaccines include:
- Rapid development: The coding sequence can be adjusted swiftly to address new pathogen variants.
- Strong immune response: mRNA vaccines tend to elicit a robust and long-lasting immune response.
- No live virus used: This lowers the risk of vaccine-induced disease.
However, there are challenges too. The storage requirements for mRNA vaccines necessitate cold chain logistics, which can make distribution difficult in certain areas. Additionally, there is ongoing research to determine long-term effects of these vaccines, although current data is favorable.
"mRNA technology is revolutionizing how we think about vaccine development and response to new diseases."
Gene Therapy Applications
Gene therapy shares a close relationship with mRNA technology. This approach aims to treat or prevent diseases by modifying genes within an individual’s cells. There are several strategies within gene therapy that utilize mRNA to achieve therapeutic effects. For instance, mRNA can be used to encourage the production of specific proteins that might be lacking or malfunctioning in genetic disorders.
One strong point of mRNA in gene therapy is its flexibility. Researchers can design mRNA to target specific cells, making it possible to localize treatment effectively. Areas of application include:
- Cancer treatment: Targeting tumor cells with mRNA codes to produce proteins that induce an anti-tumor immune response.
- Rare genetic disorders: By providing mRNA that codes for a missing protein, gene therapy can potentially rectify the underlying cause of these illnesses.
Despite the promise, gene therapy also faces hurdles. Issues such as effective delivery systems for mRNA and the body’s immune response to administered mRNA are active areas of research. It is crucial to overcome these challenges to make gene therapies more successful in clinical settings.
Future Perspectives
The field of messenger RNA (mRNA) research is rapidly evolving. Understanding its structure and function opens doors to innovative applications in science and medicine. As researchers continue to uncover the complexities of mRNA, several aspects merit attention for future exploration.
One significant area is the expanding realm of gene editing technologies. Advancements such as CRISPR-Cas9 have heightened the interest in utilizing mRNA for precise genomic modifications. This method offers potential for correcting genetic disorders, thus providing a pathway for therapies that were previously thought unattainable.
Moreover, the evolution of mRNA vaccines during the COVID-19 pandemic exemplifies a breakthrough in vaccine technology. The adaptability of mRNA makes it suitable for rapid development against various pathogens. Future vaccines may take advantage of this feature to combat not just infectious diseases, but also cancers.
Researchers must consider the implications of these innovations in ethical terms. Discrepancies in access to therapies and the risk of misuse are critical points of discussion.
"The future of mRNA research is not merely academic; it impacts global health and societal structures."
In this section, we delve deeper into two emerging themes in mRNA research: emerging research areas and potential therapeutic innovations.
Emerging Research Areas
Emerging research areas in mRNA technology encompass a broad spectrum of scientific inquiry. Here are some key realms being actively explored:
- Next-Generation Vaccines: Researchers are investigating how mRNA can be utilized beyond vaccines for viral infections. This includes development against diseases like malaria and HIV.
- mRNA Therapeutics for Cancers: Immunotherapy strategies are focusing on mRNA to induce immune responses that target tumors. This area shows promise for personalized treatment plans based on individual genomic profiles.
- Regenerative Medicine: The use of mRNA in cell reprogramming is an exciting frontier. Scientists are working with mRNA to convert somatic cells into pluripotent stem cells, potentially leading to breakthroughs in tissue repair and organogenesis.
Continued exploration in these areas holds the potential to revolutionize healthcare and address unmet medical needs.
Potential Therapeutic Innovations
Potential therapeutic innovations involving mRNA are breaking ground and include several fascinating concepts:
- Cancer Vaccines: Developing mRNA vaccines specifically tailored to provoke an immune response against tumor antigens. This approach aims at targeting cancer cells while sparing healthy ones.
- Autonomous mRNA Delivery: Novel delivery systems are being developed to enhance mRNA stability and localization. This aims to improve the efficiency of mRNA therapies.
- Combination Therapies: Combining mRNA therapies with existing treatments. For example, integrating mRNA cancer vaccines with immune checkpoint inhibitors could enhance overall efficacy.
The End
The conclusion serves as a vital component of any comprehensive analysis on mRNA. Here, it synthesizes the extensive discussions on the molecular intricacies, functional roles, and biotechnological implications of messenger RNA. By revisiting the core themes of the article, readers are offered a consolidated understanding of mRNA's significance in cellular processes and its applications in modern medicine.
Understanding mRNA equips students, researchers, educators, and professionals with insights that extend beyond biological concepts. Its complex structure, including the 5' cap, 3' poly-A tail, and various post-transcriptional modifications, is essential for appreciating the nuances of gene expression and regulation.
Moreover, exploring applications such as mRNA vaccines highlights the transformative impact of this molecule in the field of biotechnology. With emerging research areas pointing to novel therapeutic innovations, the relevance of mRNA continues to grow. This culmination of key insights underscores not only the foundational biological principles but also the forward-looking aspects of mRNA.
Summary of Key Points
- Molecular Structure: mRNA comprises ribonucleotides, a phosphate backbone, and capping structures critical for stability and translation.
- Production and Function: mRNA is synthesized during transcription, where RNA polymerase plays a key role, ultimately guiding protein synthesis.
- Post-Transcriptional Modifications: Modifications such as capping, splicing, and polyadenylation enhance mRNA stability and functionality.
- Regulation and Localization: The regulation of mRNA is key to gene expression control, while its localization within cells supports efficient translation.
- Biotechnological Advancements: mRNA technology is pivotal in the development of vaccines and gene therapies, marking advancements in medical science.
In summary, the conclusion not only wraps up the various discussed elements but also emphasizes the importance of understanding mRNA in both basic biology and applied sciences. Recognizing mRNA's capabilities and potential inspires future research and applications that may change the landscape of medicine.