Understanding Mass Spectrometry Graphs: A Guide

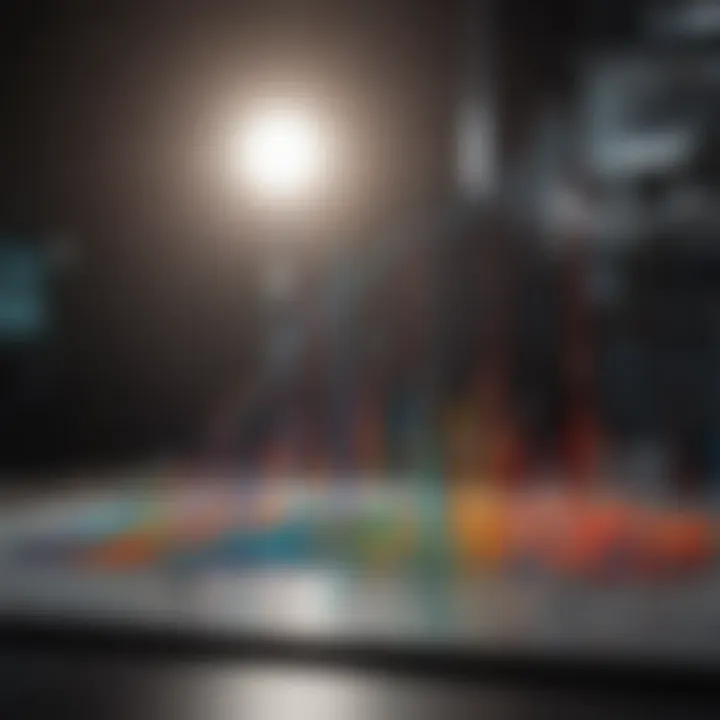
Intro
Mass spectrometry has emerged as a critical technique in analytical chemistry, offering profound insights into molecular structures and compositions. The graphs produced from this technology provide visual representations that are essential for understanding complex data. To decode these graphs effectively, one needs not only familiarity with the basic principles of mass spectrometry but also an appreciation for the nuances of data interpretation. This guide meticulously unpacks the key aspects surrounding mass spectrometry graphs, emphasizing their applications across diverse scientific fields.
Methodology
Overview of Research Methods Used
In the realm of mass spectrometry, various research methods underpin the creation and analysis of mass spectra. These methods typically involve:
- Ionization Techniques: Different techniques like Electron Ionization and Matrix-Assisted Laser Desorption/Ionization are used to ionize the sample.
- Mass Analyzers: Instruments such as Time-of-Flight (TOF) and Quadrupole Mass Filters separate ions based on their mass-to-charge ratios.
- Data Processing and Analysis: Sophisticated software is utilized to process raw data and generate interpretable graphs.
Data Collection Techniques
The accuracy and reliability of mass spectrometry graphs heavily depend on the methods applied during data collection. Important aspects include:
- Sample Preparation: Proper sample treatment is crucial for obtaining valid results. Contaminants or improper technique can lead to misleading spectra.
- Calibration: Regular calibration of instruments ensures high precision in the measurements.
- Replicate Analysis: Conducting multiple analyses of the same sample helps identify variability and ensure reproducibility of results.
Understanding the Graphs
Interpreting mass spectrometry graphs requires a fundamental understanding of spectral features:
- Peaks: Represents different ions detected during analysis. The height or intensity of a peak correlates with the ion's abundance.
- M/Z Ratio: The x-axis often denotes mass-to-charge ratios, allowing for identification of molecules.
- Resolution: The ability of the mass spectrometer to distinguish between closely spaced peaks.
"Interpreting mass spectra effectively necessitates knowledge of both the theoretical underpinnings and practical applications of mass spectrometry."
Future Directions
Upcoming Trends in Research
As technology advances, the field of mass spectrometry continues to evolve. Key trends include:
- Improvements in Resolution: Newer instruments promise higher resolution, enabling better separation of closely related substances.
- Integration with Other Techniques: Combining mass spectrometry with techniques like chromatography enhances analytical capabilities and offers more comprehensive data.
Areas Requiring Further Investigation
Despite advancements, challenges remain in the field. Notable areas for further exploration include:
- Complex Mixture Analysis: Techniques to analyze complex biological samples need refinement to improve accuracy and reliability.
- Data Interpretation Tools: Development of more advanced algorithms for data analysis could streamline the process and enhance understanding.
This guide aims to bridge the knowledge gap in mass spectrometry graphs, facilitating a richer understanding among students, researchers, and educators.
Prolusion to Mass Spectrometry
Mass spectrometry has become an invaluable tool in analytical chemistry, revealing intricate details about molecular structures and compositions. Understanding mass spectrometry is essential for researchers, educators, and students alike. This section presents a foundation that sets the stage for comprehending mass spectrometry graphs. These graphs are not mere representations; they are analytical narratives that convey complex information about the samples analyzed.
Definition and Purpose
Mass spectrometry (MS) is a technique used to identify compounds based on their mass-to-charge ratio. It provides qualitative and quantitative information about molecules, making it essential in various fields, including biochemistry, environmental science, and pharmacology. The core purpose of mass spectrometry is twofold:
- Identification: MS helps in identifying unknown substances by analyzing their unique mass spectra.
- Quantification: It allows researchers to measure the concentration of specific analytes within a sample accurately.
MS operates by ionizing chemical species and sorting the ions based on their mass. The resulting data enables scientists to understand not just what is present in a sample but also how much exists, providing deeper insights into its nature.
Brief Historical Context
The history of mass spectrometry dates back to the early 20th century. The initial steps were taken by J.J. Thomson in 1912 when he developed the first mass spectrometer. His work laid the groundwork for future advancements.
- In the 1940s, high-resolution mass spectrometry emerged, allowing for better separation of ions, leading to more detailed analysis.
- The 1960s saw the advent of gas chromatography-mass spectrometry, expanding the applications of mass spectrometry in analytical labs.
- With technological advancements, such as soft ionization techniques and improved data acquisition systems in the late 20th century, mass spectrometry experienced a significant transformation.
Today, mass spectrometry continues to evolve, integrating with other technologies and becoming a cornerstone in many scientific disciplines. As the technique advances, so does the complexity of the data it generates, making the understanding of mass spectrometry graphs even more crucial.
Mechanism of Mass Spectrometry
The mechanism of mass spectrometry is fundamental to understanding how this analytical technique operates. It forms the backbone of the entire process, delineating how molecules are ionized, analyzed, and detected. Understanding these mechanisms is crucial for interpreting mass spectrometry graphs. Several factors influence the efficiency and accuracy of mass spectra. The ionization processes and the various mass analysis techniques play a significant role in producing reliable data. By grasping these aspects, researchers can optimize mass spectrometry for their specific applications.
Ionization Processes
The ionization process is the initial step in mass spectrometry, where molecules are converted into ions. The choice of ionization technique greatly impacts the quality of the mass spectrum obtained. There are three primary ionization methods: Electron Impact Ionization, Chemical Ionization, and Electrospray Ionization.
Electron Impact Ionization
Electron Impact Ionization (EI) is a highly essential technique in the field of mass spectrometry. It generates ions by bombarding gas-phase molecules with high-energy electrons. This approach is widely used for analyzing small, volatile compounds. One key characteristic of Electron Impact Ionization is its ability to fragment ions effectively, which helps in structural elucidation. However, the potential for excessive fragmentation can be a disadvantage, as it may obscure crucial information about the parent ion.
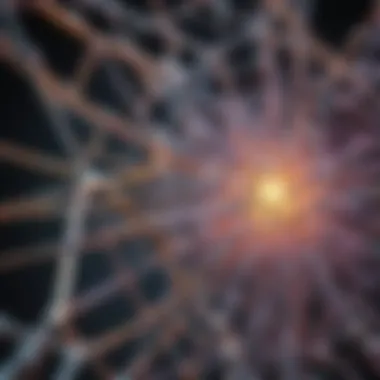
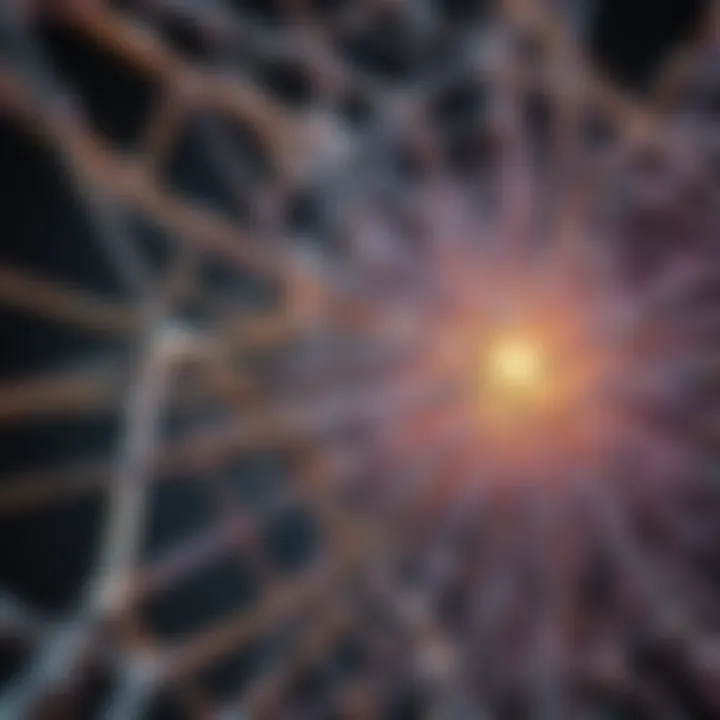
Chemical Ionization
Chemical Ionization (CI) differs from EI as it uses a reagent gas to produce ions. This method is considered softer than Electron Impact Ionization, resulting in less fragmentation of the target molecule. The key characteristic of Chemical Ionization is its ability to yield intact molecular ions, making it a more beneficial choice for identifying specific molecular weights accurately. However, it does require careful selection of the reagent gas, which can limit its application range.
Electrospray Ionization
Electrospray Ionization (ESI) is particularly valuable for analyzing large biomolecules, such as proteins and nucleotides. This technique generates ions by passing a solution through a high-voltage needle, resulting in charged droplets. The major benefit of Electrospray Ionization is its capability to produce multiple charged ions, allowing for the analysis of large molecules in the gas phase. Nevertheless, one downside is that ESI requires a liquid sample, which might not always be suitable for all analyses.
Mass Analysis Techniques
Once the molecules are ionized, the mass analysis techniques come into play. These techniques are responsible for measuring the mass-to-charge ratio of the ions produced. Each technique has its unique features, benefits, and limitations affecting the final results obtained in mass spectrometry.
Time-of-Flight (TOF)
Time-of-Flight (TOF) is an efficient method used to determine the mass of ions based on their time of travel in a vacuum. The key characteristic of TOF is its rapid acquisition of mass spectra. This speed makes it a popular option for high-throughput applications. TOF analysis permits the detection of a wide mass range without requiring a prior calibration. However, challenges exist with resolution and the ability to analyze complex mixtures due to ion swarm effects.
Quadrupole
Quadrupole mass analyzers operate by using oscillating electric fields to filter ions based on their mass-to-charge ratio. One of the significant advantages of the Quadrupole technique is its robustness and simplicity in use. It allows for selective ion detection, making it useful for targeted analysis in complex mixtures. However, Quadrupole has some limitations when it comes to higher mass resolution, which can complicate the analysis of closely related compounds.
Ion Trap
Ion Trap technology works by trapping ions in a confined space using electric or magnetic fields. A key feature of Ion Trap is its ability to accumulate ions, leading to improved detection sensitivity. This technique facilitates tandem mass spectrometry (MS/MS) capabilities, further enabling detailed structure analysis. However, Ion Trap analyzers might suffer from lower scan rates, which could affect large-scale analyses.
Components of the Mass Spectrometer
The mass spectrometer is a complex instrument that plays a crucial role in the analytical chemistry landscape. Understanding its components is essential for interpreting mass spectra effectively. Each part has a unique function, and their collective integration determines the performance and accuracy of the results. The three primary components are the ion source, the mass analyzer, and the detector. Below is a detailed examination of these components, highlighting their functions and significance in the process of mass spectrometry.
Ion Source
The ion source is the first module in a mass spectrometer. It is responsible for converting the sample, whether solid, liquid, or gas, into ions. This ionization is critical because mass spectrometry relies on the detection of charged particles. There are various techniques for ionization, including Electron Impact Ionization and Electrospray Ionization.
Each method has its own advantages. For example, Electron Impact Ionization is known for its effectiveness in analyzing volatile compounds. On the other hand, Electrospray Ionization is excellent for large biomolecules. The choice of ion source impacts the range of compounds that can be analyzed and is also vital for ensuring reproducible results. Proper optimization of the ion source can enhance sensitivity and minimize fragmentation, which can lead to clearer ion signals.
Mass Analyzer
Following the ion source, the mass analyzer separates ions based on their mass-to-charge ratios. This separation is pivotal, as it directly influences the resolution and accuracy of the resulting mass spectrum. There are several types of mass analyzers, including Quadrupole and Time-of-Flight (TOF).
- Quadrupole mass analyzers are widely used due to their robustness and speed. They can rapidly select ions of specific mass-to-charge ratios.
- Time-of-Flight (TOF) devices offer high resolution by measuring the time it takes for ions to travel a fixed distance. Higher mass ions take longer to reach the detector compared to lighter ones, thus providing a clear separation.
The mass analyzer is instrumental in providing quality data, allowing for better identification and quantitation of the compounds present in the sample. Each type possesses its unique characteristics, catering to different analytical needs.
Detector
The final component is the detector, which measures the abundance of ions produced by the mass analyzer. Detectors convert the ion signals into a measurable output that can be visualized as a mass spectrum. Common types include electron multipliers and Faraday cups.
- Electron Multipliers are highly sensitive detectors capable of detecting very low ion counts, which is crucial for trace analysis.
- Faraday Cups, while less sensitive, provide a precise measurement of ion current, making them suitable for quantitative analysis.
The effectiveness of a detector directly impacts the sensitivity and reliability of the mass spectrometry results. A well-calibrated detector ensures accuracy in the measurement of ion abundance, allowing for more precise interpretations of the mass spectrum.
In summary, the components of the mass spectrometer—ion source, mass analyzer, and detector—are integral to the operation and efficiency of the technique. Their roles are interlinked, and understanding these relationships can significantly enhance one's ability to interpret mass spectrometry data accurately and effectively.
Interpreting Mass Spectrometry Graphs
Interpreting mass spectrometry graphs is essential for understanding the data generated from mass spectrometry studies. These graphs provide insight into the molecular composition of various samples, enabling researchers to identify substances and assess their purity. By developing a strong foundation in reading and understanding these graphs, scientists can propose hypotheses, validate experiments, and make informed decisions about their research.
Understanding the Mass Spectrum
The mass spectrum is a graphical representation of ions detected by the mass spectrometer. Each peak in the spectrum corresponds to a specific ion, offering critical information about the sample being analyzed. Understanding the mass spectrum allows researchers to determine the molecular weight, structure, and abundance of components within their sample. This understanding is pivotal in a variety of fields, including analytical chemistry, biology, and pharmaceuticals.
Key Features
X-axis Interpretation
The X-axis of a mass spectrum represents the mass-to-charge ratio (m/z) of ions detected. This feature is key for understanding the molecular weights of compounds present in the sample. Each point on this axis corresponds to a unique ion, enabling researchers to pinpoint specific compounds.
The major characteristic of X-axis interpretation lies in its precision. Mass spectrometers can resolve ions with very small differences in mass, which is beneficial for detailed molecular analysis. One unique feature of this interpretation is its ability to differentiate between isotopes of the same element. However, misinterpretation can occur if the analyst overlooks significant peaks or noise in the data, leading to incorrect conclusions about the sample composition.
Y-axis Interpretation
On the Y-axis, the intensity of each ion is represented. This intensity reflects the abundance of each ion relative to the total number of ions detected. Therefore, high intensity indicates a larger amount of the specific ion in the sample.
The key characteristic of Y-axis interpretation is its direct correlation to ion abundance. This measure is popular among researchers as it provides valuable quantitative data regarding sample composition. A unique feature of this interpretation is its use in determining concentrations of substances in complex mixtures. Some disadvantages include potential errors in abundance estimation due to overlapping peaks, which can complicate analysis.
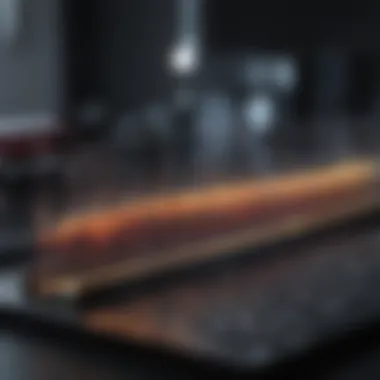
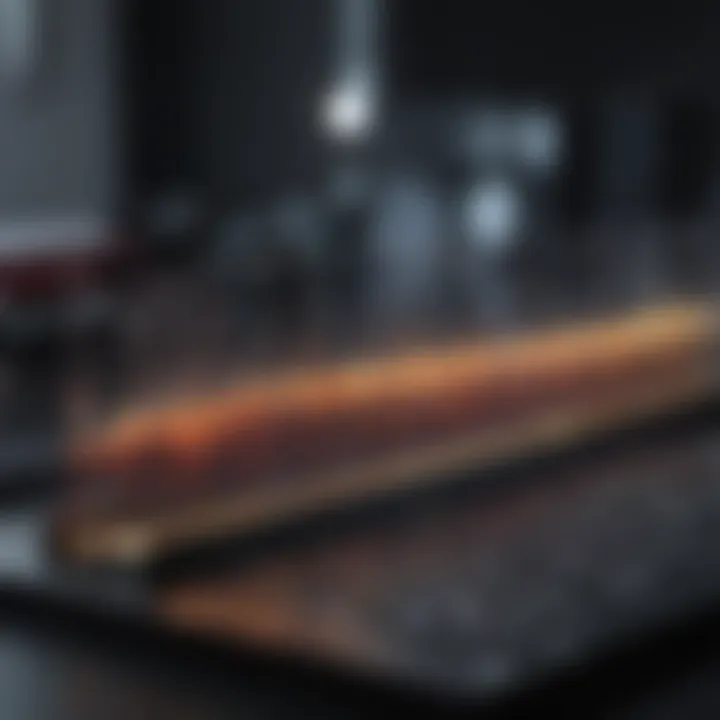
Isotopic Patterns
Isotopic patterns reveal the natural isotopic distribution of elements within a sample. Each element has a unique isotopic signature, which can help in validating molecular structures. Understanding these patterns is particularly crucial in organic chemistry and proteomics.
The defining feature of isotopic patterns is their distinctiveness. This characteristic helps researchers confirm the identity of compounds, making it a favorable choice in mass spectrometry analysis. The unique feature, in this case, is that isotopic patterns provide a fingerprint for molecules, aiding in distinguishing between similar compounds.
One advantage of incorporating isotopic patterns is the added layer of information they provide. However, these patterns may also introduce complexities in interpretation, especially when samples contain multiple elements with similar isotopic distributions.
In summary, a deep understanding of mass spectrometry graphs, especially the X-axis, Y-axis, and isotopic patterns, is crucial for accurate data interpretation and improved analysis in scientific research.
Factor Influencing Mass Spectrometry Graphs
Mass spectrometry graphs are crucial in analytical chemistry. However, the quality and accuracy of these graphs are influenced by various factors. Understanding these elements can profoundly enhance the reliability of the results obtained and provide deeper insights into molecular compositions. The following sections discuss three specific factors that significantly impact the results of mass spectrometry: sample preparation, instrument calibration, and temperature and pressure conditions. Each aspect plays an essential role in obtaining accurate mass spectra, which can affect scientific interpretations and subsequent applications.
Sample Preparation
Sample preparation is the first and often most critical step in the mass spectrometry process. The way a sample is handled greatly impacts the final results. Poorly prepared samples can introduce contaminants that interfere with the detection and quantification of the target analytes. It is important to follow specific protocols to ensure the sample is representative of the analyte characteristics. This includes ensuring that the sample is pure, free from impurities, and in the correct concentration range.
Key considerations in sample preparation include:
- Purity of the Sample: Contaminants can skew results.
- Concentration Levels: Too high or too low concentrations can lead to ion suppression or enhancement.
- Matrix Effects: The presence of other substances can influence ionization and mass detection.
Therefore, meticulous attention during sample preparation is essential to achieving high-quality mass spectra.
Instrument Calibration
Instrument calibration is fundamentally necessary in mass spectrometry. It ensures that the instrument is providing accurate mass-to-charge ratios consistently. Calibration involves comparing the instrument's readings with a known standard. Typically, calibration should be conducted regularly, especially when changes in the experimental setup occur or if the instrument has not been used for some time.
The main benefits of proper instrument calibration are:
- Improved Accuracy: Accurate results are directly linked to the reliability of the data.
- Reproducibility: Consistency across different runs enhances the credibility of the results.
- Detection Limits: Accurate calibration allows for better detection limits of low abundance analytes.
Failure to calibrate instruments can result in significant deviations, leading to misinterpretation of the sample’s molecular composition.
Temperature and Pressure Conditions
Temperature and pressure conditions during mass spectrometry cannot be overlooked. They influence the behavior of ions, which can directly affect the peaks observed in the mass spectrum. For instance, higher temperatures typically lead to enhanced ionization efficiency, while lower temperatures might cause reductions in ion generation.
Considerations in managing temperature and pressure include:
- Ion Stability: Ions generated under specific temperature and pressures might fragment differently, affecting the overall analysis.
- Vaporization of Analytes: Some analytes may not vaporize adequately under insufficient pressure or can lead to thermal decomposition at high temperatures.
In summary, controlling the temperature and pressure conditions can promote the generation of more accurate and reproducible results in mass spectrometry. Ensuring optimal conditions is crucial for harnessing the full potential of mass spectrometry in research applications.
Effective monitoring of these factors is not just necessary but indispensable for obtaining reliable mass spectrometry data. Accuracy hinges substantially on understanding and controlling these elements.
Advanced Mass Spectrometry Techniques
Advanced techniques in mass spectrometry are crucial for expanding the capabilities of this method in analyzing complex samples. They allow for high precision and better accuracy when interpreting mass spectra. Understanding these advanced techniques can help researchers to derive more meaningful results from their analyses. Two prominent methodologies under this umbrella include High-Resolution Mass Spectrometry and Tandem Mass Spectrometry (MS/MS).
High-Resolution Mass Spectrometry
High-Resolution Mass Spectrometry (HRMS) offers a significant enhancement over conventional mass spectrometers. It enables the differentiation of ions based on their mass with exceptional accuracy. Its high resolving power is essential for identifying compounds in complex mixtures, where subtle differences in mass could indicate entirely different substances.
The ability to resolve closely spaced peaks allows scientists to detect isomers and isotopes effectively. This can prove invaluable in various fields such as proteomics, metabolomics, and environmental studies. HRMS is particularly beneficial when working with substances that may share similar mass-to-charge (m/z) ratios but differ in structures and compositions.
In practice, HRMS employs various techniques, including Orbitraps and Ion Traps. These sophisticated instruments achieve better mass accuracy, typically in the range of parts per million (ppm), which can be critical in research where precise molecular weights are vital. The resulting high-fidelity data that HRMS provides enables more rigorous analysis, including quantitative determinations, and helps in confirming the structures of unknown compounds.
Tandem Mass Spectrometry (MS/MS)
Tandem Mass Spectrometry, commonly known as MS/MS, represents another level of analytical depth in mass spectrometry. This technique involves two stages of mass analysis, which adds a layer of selectivity and sensitivity in detecting target analytes. It enables the researcher to first select a specific ion for fragmentation before analyzing the resulting fragments.
The fragmentation pattern of specific ions provides insights about molecular structures. This makes MS/MS particularly useful in biomolecular analysis, including peptides and proteins. By analyzing the sequence of signals produced during fragmentation, one can deduce the arrangement of amino acids, thus facilitating protein identification and characterization.
MS/MS has various applications across scientific disciplines. In drug development, it serves to identify metabolite structures, helping researchers understand the pharmacokinetics. In the environmental field, it can track contaminants in biological samples with greater specificity.
MS/MS techniques are effective in complex sample environments, reducing interference and improving detection limits.
Both High-Resolution Mass Spectrometry and Tandem Mass Spectrometry significantly contribute to the robustness and precision of mass spectrometry as an analytical tool. Researchers who leverage these techniques are better equipped to navigate the complexities inherent in modern scientific inquiries, ultimately leading to more reliable and reproducible outcomes.
Applications of Mass Spectrometry Graphs
Mass spectrometry graphs play a crucial role in various scientific fields. Their ability to provide detailed molecular information allows researchers to analyze components in complex mixtures. Understanding these graphs enhances researchers' ability to make informed decisions based on empirical data. This section will highlight some key applications, illustrating their significance in practical scenarios and providing an overview of how these graphs contribute to scientific advancement.
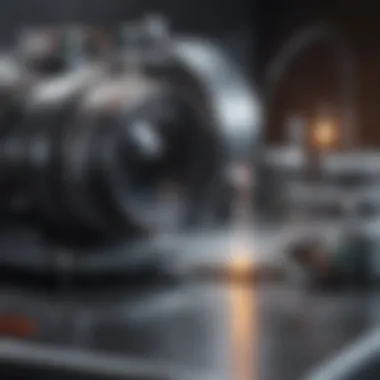
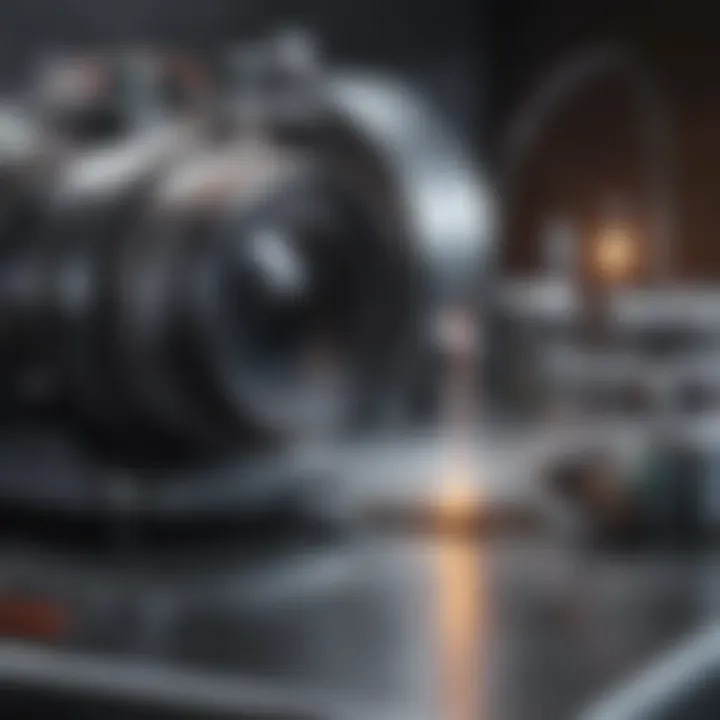
Biomolecular Analysis
In the realm of biomolecular analysis, mass spectrometry graphs are indispensable tools. They assist in the identification and quantification of biomolecules such as proteins, metabolites, and nucleic acids. One significant benefit of using mass spectrometry is its high sensitivity and specificity, allowing for the detection of low-abundance species within biological samples.
The process typically begins with sample preparation, followed by ionization and analysis, resulting in a mass spectrum that correlates to specific biomolecular components. Researchers can then use this data to explore biological processes, understand disease mechanisms, or study metabolic pathways. Furthermore, advancements in techniques like tandem mass spectrometry enable the analysis of complex mixtures, resulting in richer data sets that reveal more nuanced insights into cellular functions.
Environmental Monitoring
Environmental monitoring greatly benefits from the application of mass spectrometry graphs. These graphs help chemists and environmental scientists detect pollutants and toxins in various matrices, such as air, water, and soil. The ability to analyze trace levels of contaminants accurately contributes to monitoring environmental health and regulatory compliance.
For instance, mass spectrometry can be employed to monitor pesticide residues in agricultural products or to assess air quality by identifying volatile organic compounds. The precision of mass spectrometry ensures that even low concentrations of harmful substances can be identified, aiding in risk assessment and hazard analysis. This capability is essential for establishing safe environmental standards and devising effective remediation strategies.
Pharmaceutical Development
In pharmaceutical development, mass spectrometry graphs are vital during different stages of drug formulation and testing. These graphs provide insight into molecular weight, structure elucidation, and stability of drug candidates, making them essential for any new pharmaceutical endeavor.
During the drug development process, mass spectrometry can be utilized for pharmacokinetic studies. It allows scientists to monitor the absorption, distribution, metabolism, and excretion of drugs in biological systems, leading to optimized therapeutic strategies. Furthermore, by employing high-resolution mass spectrometry, researchers can identify impurities and degradation products, ensuring purity and efficacy of the final product.
Additionally, mass spectrometry also plays a role in the quality control of pharmaceutical products. It assures that the contents meet regulatory standards and that the formulations are consistent across batches, minimizing risks associated with variability and enhancing patient safety.
Overall, the applications of mass spectrometry graphs underscore their importance across multiple domains. Each area, from biomolecular analysis to environmental monitoring and pharmaceutical development, illustrates the versatility and significance of mass spectrometry as a tool for innovation and research.
Limitations of Mass Spectrometry
Mass spectrometry, while a cornerstone technique in analytical chemistry, inherently possesses limitations that should be recognized and understood. Addressing these limitations is crucial for researchers and analysts as they interpret data and draw conclusions. Understanding the challenges can foster more informed decisions when applying mass spectrometry, ensuring that the results are scrutinized within the confines of its operational bounds.
Sensitivity Issues
Sensitivity in mass spectrometry refers to the ability of the system to detect low concentrations of analytes in a sample. Although mass spectrometry is generally considered to be a highly sensitive technique, this sensitivity can be affected by various factors. The ionization efficiency of different compounds varies significantly, leading to the possibility that some analytes may be underrepresented in the final spectrum if their ionization occurs at a lower efficiency.
- Matrix Effects: The presence of other molecules in the sample can lead to ion suppression or enhancement, heavily influencing sensitivity. When a complex mixture is analyzed, the target analyte might not be the most abundant ion present, resulting in misleading concentrations.
- Detection Limits: Instruments may have specific detection limits that prohibit the identification of very low concentrations. For example, certain analytes may become undetectable due to scattered background signals or noise, requiring careful tuning of the analytical parameters to optimize performance.
These factors can skew results and lead to potential misinterpretation if analysts do not take them into account. The trade-offs between sensitivity and specificity must be critically evaluated in every experiment.
Complexity of Samples
Mass spectrometry excels in its ability to analyze a vast array of samples, from small organic compounds to large biomolecules. However, the complexity of the sample can pose significant challenges. A sample's complexity can manifest in several ways, complicating analysis and interpretation.
- Chemical Interference: In heterogeneous samples, different components can share similar mass-to-charge ratios, leading to overlaps in their corresponding peaks in the mass spectrum. This makes it difficult to distinguish between compounds effectively, and quantification can become inaccurate.
- Chromatographic Steps: Many protocols involve separation techniques, such as gas or liquid chromatography, before applying mass spectrometry. If the separation is insufficient, co-eluting compounds can contaminate the results and mislead interpretations.
- Requires Prep Work: Complex samples may necessitate extensive pre-processing, including extraction and purification steps, which can introduce variability and potential contamination. This additional workload can strain resources and time.
Understanding these complexities is vital for accurately interpreting mass spectrometry data. Analysts must employ thorough preparative techniques and remain vigilant about potential interferences when analyzing intricate mixtures.
"Awareness of the limitations of mass spectrometry is as important as mastering its advantages."
Future Perspectives in Mass Spectrometry
The field of mass spectrometry is continuously evolving. Future perspectives focus on enhancing techniques and expanding applications. This section will cover how these advancements influence both research and practical applications across various fields.
Emerging Technologies
New technologies are emerging that enhance the capabilities of mass spectrometers. For instance, developments in miniaturization are leading to portable mass spectrometry. This portability opens doors for field analysis, allowing researchers to conduct experiments outside the traditional lab environment.
Additionally, advancements in data analysis software are crucial. These programs can now process large datasets more efficiently, leading to faster interpretations. Machine learning and artificial intelligence are becoming integral. These technologies help in identifying patterns and anomalies in mass spectra that may not be visible to the human eye.
"The integration of AI in data interpretation could revolutionize how mass spectra are analyzed and understood."
Moreover, new ionization techniques are under development. Methods such as vacuum ultraviolet and laser-based ionization allow for the analysis of more complex samples. These techniques improve sensitivity and ensure that trace components can be identified with greater accuracy.
Integration with Other Analytical Techniques
The future of mass spectrometry also lies in its integration with other analytical methods. Combining mass spectrometry with chromatography, such as gas chromatography (GC-MS) or liquid chromatography (LC-MS), allows for better separation of complex mixtures. This integration results in clearer data and more precise identification of compounds.
In addition, mass spectrometry can complement NMR spectroscopy and X-ray crystallography. Using these methods together enhances the structural elucidation of compounds, providing insight into their composition and function.
Furthermore, the combination of mass spectrometry with imaging techniques opens pathways in biomedical research. For example, mass spectrometry imaging (MSI) provides spatial distribution of molecules within tissues, aiding in disease diagnosis and therapeutic monitoring.
End
The conclusion of this article encapsulates the crucial insights regarding mass spectrometry graphs. Understanding these graphs is vital for anyone in the field of analytical chemistry. They not only represent the data generated by mass spectrometers but also provide fundamental information about the nature of the samples analyzed. Properly interpreting mass spectra can lead to significant advancements in various scientific domains.
Through the discussions in this article, key elements have been highlighted, such as the importance of accurate sample preparation, instrument calibration, and the understanding of advanced mass spectrometry techniques. Each factor plays a pivotal role in ensuring the reliability of data represented in mass spectrometry graphs.
Here are some benefits of mastering mass spectrometry interpretation:
- Enhanced Analytical Skills: Proficiency in reading mass spectra can significantly improve one's analytical capabilities, aiding in identifying molecular structures.
- Accurate Data Interpretation: With a thorough understanding of graphs, researchers can uncover intricate details about their samples, leading to more informed decisions in their work.
- Broader Applications: Knowledge of mass spectrometry fosters innovation across various fields, including pharmaceuticals, environmental science, and biochemistry.
In consideration of these factors, synthesizing knowledge about mass spectrometry graphs enables researchers and practitioners to utilize this powerful analytical tool effectively, maximizing its potential across multiple scientific applications.
Key Takeaways
- Understanding Graph Interpretation: Familiarity with reading mass spectra allows for accurate identification of molecular components.
- Significance of Calibration: Regular calibration of the instrument ensures that the data collected is reliable and applicable.
- Sample Quality Matters: Proper sample preparation influences the results. It is essential to account for physical and chemical properties before analysis.
- Application Scope: Mastery over mass spectrometry techniques opens doors to numerous applications in varied fields such as drug development, environmental analysis, and biomolecular studies.
- Continued Learning: As technology evolves, remaining updated with emerging techniques will enhance analytical prowess.