From DNA to RNA: Understanding the Transcription Process
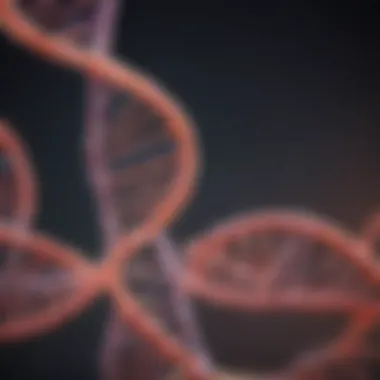
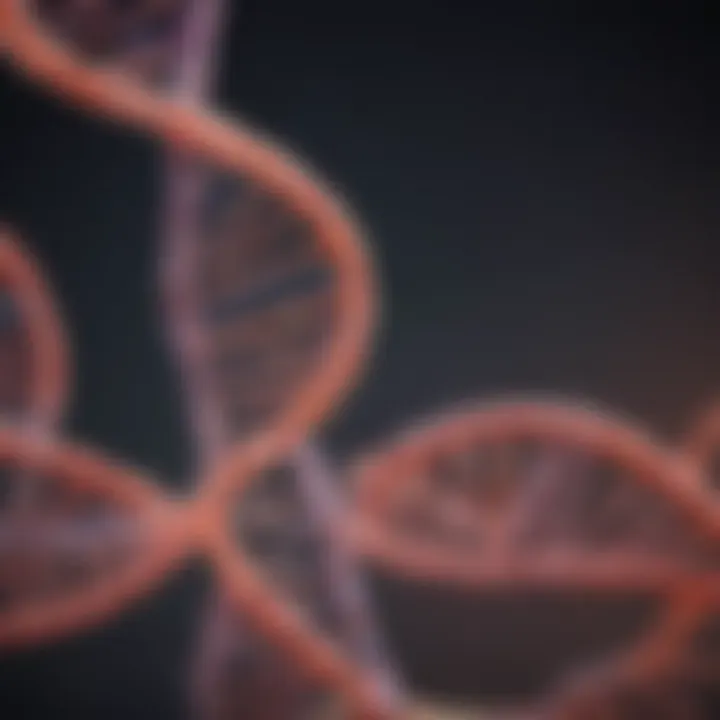
Intro
The transition from DNA to RNA is a crucial aspect of molecular biology, encapsulating the journey of genetic information in living organisms. Understanding this process is essential for a wide range of fields, including genetics, biotechnology, and medicine. The mechanisms of transcription, where DNA is transcribed into messenger RNA, are intricate yet fascinating. During this process, different enzymes and factors interact to ensure the accurate synthesis of RNA. Moreover, the significance of RNA cannot be understated, as it plays a central role in the synthesis of proteins, which are vital for all cellular functions.
This article aims to provide a comprehensive overview of this transition, detailing the fundamental processes involved in transcription, the roles of various enzymes, and the implications for protein synthesis. The genetic code, stored in DNA, is translated into an active form through RNA, allowing cells to function and adapt to their environment. As we explore these concepts deeper, it becomes clear that understanding the transcription process not only informs our knowledge of biology but also has profound implications for developments in research and therapeutic interventions.
Methodology
Overview of research methods used
To analyze the transcription process, a combination of experimental and computational methods has been utilized. Techniques such as RNA sequencing, polymerase chain reaction (PCR), and chromatin immunoprecipitation (ChIP) have provided critical insights into the dynamics of transcription. These methods allow scientists to observe the behavior of RNA polymerase, the enzyme responsible for synthesizing RNA from a DNA template, and examine the regulation of gene expression in real time.
Data collection techniques
Data collection in transcription studies often involves:
- RNA-Seq: This high-throughput sequencing method enables the quantification of RNA levels in various conditions, providing a snapshot of gene expression.
- ChIP-Seq: This technique helps researchers identify the binding sites of transcription factors and other regulatory proteins on DNA.
- In vitro transcription assays: These assays allow for the detailed study of transcriptional activities under controlled conditions.
Through these methodologies, researchers can gather significant data that contributes to understanding how genetic information is expressed.
Future Directions
Upcoming trends in research
As the field evolves, several trends in transcription research are emerging. For instance, the integration of single-cell RNA sequencing is gaining popularity, allowing scientists to study transcription at an individual cell level. This approach can reveal the heterogeneity in gene expression that occurs among cells in a tissue, leading to new insights into developmental processes and disease mechanisms.
Areas requiring further investigation
Despite the advancements in understanding transcription, there are still many questions that remain unanswered. Areas such as:
- The precise mechanisms of transcription factor interactions.
- The role of non-coding RNAs in transcription regulation.
- The effects of chromatin structure on transcription efficiency.
These topics necessitate further investigation to illuminate the complexities of gene expression regulation, potentially leading to new therapeutic strategies.
In summary, the transition from DNA to RNA involves a myriad of processes that are vital for life. A comprehensive understanding is essential for future scientific advancements.
Prelims to DNA and RNA
The exploration of DNA and RNA is fundamental to understanding the essence of life itself. These molecules not only encode genetic information but also play critical roles in the expression of this information into functional proteins. This introduction serves as a foundation for delving into the complexities of transcription, the process through which DNA is converted into RNA, setting the stage for further analysis in the article.
The Genetic Blueprint
DNA, or deoxyribonucleic acid, serves as the blueprint for life. It contains the instructions required for the growth, development, and functioning of living organisms. Every cell in a multicellular organism houses a copy of this genetic information. To understand this biological instruction set, one must recognize that DNA consists of nucleotides, which are comprised of a sugar, a phosphate group, and nitrogenous bases. The sequence of these bases determines the genetic code.
RNA, or ribonucleic acid, plays a pivotal role in the transition from genetics to functional proteins. Unlike DNA, RNA is often single-stranded and contains uracil in place of thymine. There are several types of RNA, each with specific functions in the cell.
Key Differences Between DNA and RNA
Understanding the differences between DNA and RNA is crucial for grasping their respective roles in molecular biology. Here are some key distinctions:
- Structure: DNA is a double-stranded helix, while RNA is single-stranded.
- Sugar Component: DNA contains deoxyribose sugar; RNA contains ribose sugar, which has an additional oxygen atom.
- Nitrogenous Bases: DNA has adenine, thymine, cytosine, and guanine, whereas RNA has adenine, uracil, cytosine, and guanine.
- Function: DNA's primary role is the long-term storage of information, whereas RNA acts as a messenger carrying instructions from DNA for controlling the synthesis of proteins.
The understanding of these basic elements provides insight into how genetic information is expressed and regulated. The next section will discuss transcription, emphasizing its definition and importance in the broader context of genetics.
The Concept of Transcription
Transcription is a vital biological process that forms the foundation of gene expression. It serves as the initial step in converting the genetic information encoded in DNA into functional products, primarily proteins. Understanding transcription is essential not only for comprehending how genes are expressed but also for grasping the intricacies of cellular functions.
Definition and Importance
Transcription can be defined as the process by which an RNA molecule is synthesized from a DNA template. This is achieved through the action of enzymes, primarily RNA polymerase. The significance of transcription lies in its role as a regulatory mechanism that determines when, where, and how much of a particular gene product is produced. Without transcription, the intricate web of processes that lead to protein synthesis would be impossible.
Transcription initiates with the recognition of a specific gene's promoter region by RNA polymerase. This is followed by the unwinding of the DNA double helix, paving the way for the synthesis of a complementary RNA strand. The resultant mRNA strand carries a copy of the information encoded in the DNA, allowing it to be transported from the nucleus to the cytoplasm, where translation occurs.
The importance of transcription extends beyond mere gene expression. It plays a role in cellular responses to environmental changes, contributes to developmental processes, and aids in maintaining cellular homeostasis.
Transcription Factors and Their Roles
Transcription factors are proteins that regulate the transcription process by binding to specific DNA sequences. They can either promote or inhibit the transcription of a gene, acting as crucial regulators within the gene expression framework. Their interplay is complex, affecting the efficiency and accuracy of RNA synthesis.
Key roles of transcription factors include:
- Enhancing promoter activity: Certain transcription factors can increase RNA polymerase's ability to bind to a promoter, thereby initiating transcription more effectively.
- Repressing transcription: Other factors can prevent RNA polymerase from attaching to the promoter, inhibiting the transcription of specific genes.
- Facilitating the assembly of the transcription complex: Transcription factors can recruit additional proteins necessary for transcription to occur smoothly.
In summary, the concept of transcription is not merely a technical process but a cornerstone of genetic regulation and cellular function. Understanding it not only illuminates the path from DNA to RNA but also highlights the intricate controls exerted within cellular systems.
Molecular Players in Transcription
The process of transcription is complex and necessitates the collaboration of various molecular players. Understanding these participants is critical for comprehending how genetic information flows from DNA to RNA. Each player contributes uniquely, making transcription a finely-tuned process sensitive to numerous biological factors. Their interplay serves to ensure that genes are accurately expressed. When examining this topic, it is evident that the role of these molecular players can directly influence cellular function and the overall health of the organism.
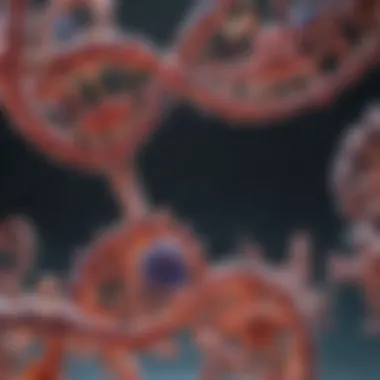
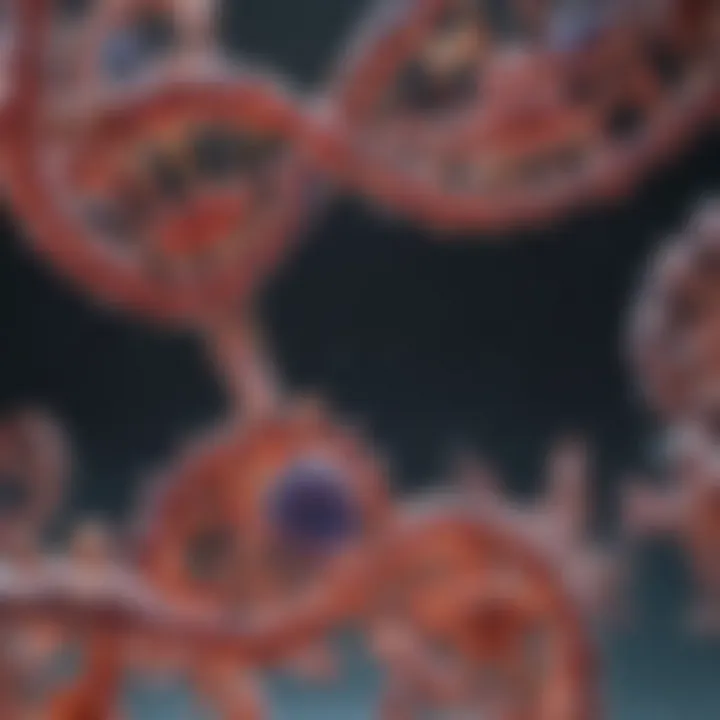
Role of RNA Polymerase
RNA polymerase is the enzyme primarily responsible for synthesizing RNA from a DNA template. There are several types of RNA polymerase, but in eukaryotic cells, RNA polymerase II is essential for mRNA synthesis. Its function begins when it binds to the promoter region of a gene, marking the starting point of transcription.
RNA polymerase has several critical roles:
- Binding to DNA: It recognizes specific DNA sequences located at the start of genes, allowing it to initiate the transcription process accurately.
- Synthesis of RNA: Once bound, RNA polymerase unwinds the DNA and synthesizes a complementary RNA strand by adding ribonucleotides. The nucleotides are assembled in the 5' to 3' direction, reflecting the sequence encoded in the DNA.
- Proofreading: RNA polymerase incorporates a proofreading mechanism to ensure accuracy during RNA synthesis by removing incorrectly paired nucleotides. This reduces the chance of errors in the RNA product.
Understanding the significance of RNA polymerase is crucial for appreciating overall gene expression and the potential implications for genetic research.
DNA Sequences That Facilitate Transcription
Certain DNA sequences play a vital role in transcription, guiding the binding and activity of RNA polymerase. These sequences not only serve as starting points but also influence the frequency of transcription. The elements of DNA that facilitate transcription include:
- Promoter Regions: Located upstream of genes, promoters contain specific motifs that are recognized by RNA polymerase and transcription factors. These regions are essential for the initiation of transcription.
- Enhancers: Enhancers are regulatory sequences that can be found thousands of bases away from the promoter. They enhance the transcription levels of specific genes, acting exponentially. They work through the looping of DNA, bringing distant enhancer regions in proximity to the promoter, thus facilitating RNA polymerase's access.
- Silencers: These are sequences that suppress transcription when bound by repressor proteins. Their role is crucial in gene regulation, adding layers of control to when and how much a gene expresses itself.
By comprehending the diverse roles of these sequences, researchers can better understand transcriptional regulation and the complexity of gene expression.
Involvement of Promoters and Enhancers
Promoters and enhancers are integral components of the transcriptional machinery. The promoter is often described as the main hub where RNA polymerase begins its transcription task. Without a functional promoter, RNA polymerase cannot initiate transcription, making it impossible for a gene to be expressed.
Enhancers provide additional layers of control and can dramatically increase the likelihood of transcription occurring. While promoters are essential for all genes, enhancers allow for fine-tuning, and they are gene-specific. Some critical aspects include:
- Spatial Influence: Enhancers can function at a distance, interacting with the promoter through DNA looping mechanisms, thus providing dynamic regulation based on cellular context.
- Cell-Specific Regulation: Different cell types express distinct sets of enhancers, leading to unique patterns of gene expression necessary for the function of varied tissues. This is particularly evident in complex organisms.
- Developmental Timing: Enhancers can also be involved in temporal regulation, switching genes on and off during different stages of development, ensuring appropriate gene expression at each step.
Together, promoters and enhancers illustrate the intricate regulatory systems that dictate transcription, showcasing the complexity and sophistication of molecular biology.
Understanding the various molecular players involved in transcription is essential for grasping the nuances of gene expression. Their roles and interactions can have significant implications in areas like genetics, biotechnology, and medicine.
The Process of Transcription
Transcription is a pivotal process in the flow of genetic information from DNA to RNA. This process not only serves as a bridge between the genetic code and the proteins that execute various cellular functions but also underscores the significance of gene expression regulation. Understanding transcription involves examining not only its basic mechanics but also its implications on cellular behavior and functionality.
Initiation of Transcription
The initiation phase marks the beginning of transcription, where several key steps come into play. It starts when RNA polymerase, the main enzyme responsible for synthesizing RNA, binds to a specific region of the DNA known as the promoter. This region contains specific sequences that are recognized by transcription factors, which facilitate the recruitment of RNA polymerase.
Once the RNA polymerase is in position, the DNA strands unwind, exposing the sequence that will be transcribed. It's essential that this process is tightly regulated, as incorrect initiation can lead to failed or erroneous gene expression. The selectiveness of this phase is crucial for ensuring that only the required genes are transcribed in accordance with cellular demands.
Elongation Phase
After the initiation, the elongation phase occurs, characterized by the actual synthesis of RNA. RNA polymerase moves along the DNA template, adding complementary ribonucleotides to build the growing RNA strand. Notably, the enzyme synthesizes RNA in the 5’ to 3’ direction. Unlike DNA replication, only one strand is synthesized at a time in transcription.
During this phase, the DNA strands rewind behind the RNA polymerase, allowing the process to maintain a steady state while also protecting the DNA’s integrity. The accuracy of elongation is vital, as misincorporation of nucleotides can lead to mutations in the resulting RNA, ultimately affecting protein synthesis.
Termination Mechanism
The transcription process concludes with the termination mechanism. This phase is critical as it ensures that RNA synthesis stops at the right moment, producing a complete and functional RNA transcript. Termination is triggered by specific sequences in the DNA that signal RNA polymerase to detach from the DNA template.
Once the transcription is complete, the new RNA strand is released. This transcript may undergo further modifications before it can participate in the broader processes of protein synthesis. Understanding the nuances of termination helps clarify how genes are turned on and off, impacting the overall expression profile of the cell.
In summary, the process of transcription is a complex yet well-coordinated sequence of events that is crucial in gene expression regulation. The steps of initiation, elongation, and termination each contribute to the precision of RNA synthesis, reinforcing their significance in biological systems.
Post-Transcriptional Modifications
Post-transcriptional modifications play a critical role in the maturation of RNA molecules. After transcription, the primary RNA transcript, known as precursor mRNA or pre-mRNA, undergoes several key modifications before it is ready for translation into proteins. These changes enhance the stability, transport, and functionality of RNA. Without these modifications, the RNA would be less effective in conveying the genetic information encoded in DNA.
Capping and Polyadenylation
Capping is a vital step in RNA processing that occurs at the 5' end of the RNA molecule. A special structure known as the 5' cap is added shortly after transcription begins. This cap protects the RNA from degradation by exonucleases and facilitates ribosome binding during the translation process. Moreover, it plays roles in the nuclear export of mRNA and is essential for proper 5' end recognition by the protein synthesis machinery.
On the other end, polyadenylation involves adding a long stretch of adenine ribonucleotides, called poly(A) tail, at the 3' end of the RNA molecule. This modification also contributes to RNA stability and enhances the efficiency of translation. The length of the poly(A) tail can influence the lifespan of the mRNA in the cell, with longer tails generally correlating with increased stability.
These two modification processes are crucial because they help ensure a mature mRNA molecule is ready for the next stages of protein synthesis.
Splicing Process and Its Significance
Splicing is another fundamental post-transcriptional modification. During this process, introns, or non-coding regions, are removed from the pre-mRNA molecule, while exon regions are joined together. This modification creates a continuous coding sequence that can be translated into a protein.
The significance of splicing cannot be overstated. It contributes to the diversity of proteins through a phenomenon called alternative splicing. This allows a single gene to produce multiple protein isoforms by including or excluding certain exons in the final mRNA product. As a result, splicing adds a layer of complexity to gene expression and enhances the versatility of proteins that can be generated from the genome.
Understanding these processes provides insight into the regulation of gene expression and how cells control protein synthesis. Post-transcriptional modifications are not merely a set of steps; they are essential for proper cell function, responding to environmental signals, and maintaining cellular homeostasis.
"Post-transcriptional modifications are crucial for mRNA stability, localization, and translation efficiency. They shape the RNA landscape and influence cellular outcomes."
Types of RNA and Their Functions
Understanding the different types of RNA is essential for grasping how genetic information is expressed in living organisms. Each type of RNA plays a unique role in the cell, contributing to the overall process of gene expression and protein synthesis. This article focuses on three primary forms of RNA: messenger RNA (mRNA), ribosomal RNA (rRNA), and transfer RNA (tRNA). Each has a specific function that enhances cellular activities and promotes the correct translation of genetic information.
Messenger RNA (mRNA)
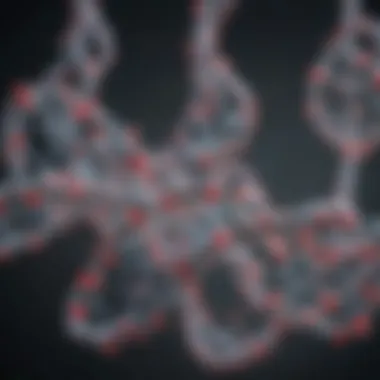
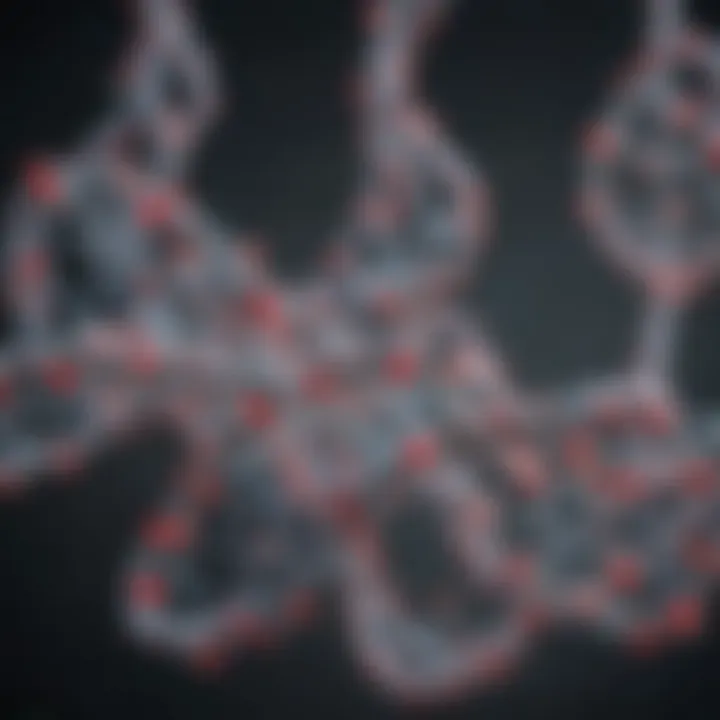
Messenger RNA, or mRNA, is pivotal in the transition from DNA to protein. It serves as a transcript of the genetic code, carrying the information necessary for assembling amino acids into proteins. mRNA is produced during transcription when RNA polymerase synthesizes it based on the DNA template. This process ensures the genetic instructions encoded within DNA are conserved, allowing them to be translated into functional proteins.
The structure of mRNA is designed for effectiveness. It typically contains a coding sequence that dictates the order of amino acids. Additionally, mRNA has a 5' cap and a poly-A tail at the ends, which play crucial roles in stability and translation efficiency. The cap protects the mRNA from degradation and aids in the initiation of translation. The poly-A tail contributes to mRNA stability and helps in the export of the mRNA from the nucleus to the cytoplasm.
Ribosomal RNA (rRNA)
Ribosomal RNA, rRNA, constitutes the core component of ribosomes, the molecular machines responsible for synthesizing proteins. It plays both structural and functional roles in protein synthesis. Ribosomes are composed of two subunits: the large and small subunits, each containing its rRNA and proteins. The rRNA not only provides a scaffold for the ribosomal proteins but also catalyzes the formation of peptide bonds between amino acids during translation, making it a component of a ribozyme.
The significance of rRNA cannot be understated. It is essential for the proper functioning of ribosomes, ensuring accurate translation of the mRNA sequence into a polypeptide. Various types of rRNA are present in both prokaryotic and eukaryotic cells, differing slightly in their sequences and sedimentation rates. This diversity reflects the evolutionary adaptations of ribosomes in different organisms.
Transfer RNA (tRNA)
Transfer RNA, or tRNA, is vital for translating the mRNA sequence into a corresponding protein. Each tRNA molecule is equipped with an anticodon, a sequence that pairs with a specific codon on the mRNA strand. tRNA’s primary role is to deliver the appropriate amino acid to the ribosome during translation. This ensures that the protein is synthesized in accordance with the mRNA template.
The tRNA structure is unique and optimized for its function. It has a cloverleaf shape, allowing it to recognize and bind specific amino acids and interact efficiently with the corresponding codon on mRNA. There are 20 different amino acids, each associated with one or more tRNA molecules. This redundancy provides a level of robustness in the translation process, minimizing errors arising from potential mismatches.
"Each type of RNA has distinct roles, making the coordination between them essential for accurate and efficient protein synthesis."
The Role of RNA in Protein Synthesis
The process of protein synthesis is a fundamental aspect of cellular function. RNA plays a central role in this mechanism. Without it, the genetic instructions contained within DNA could not be effectively transformed into functional proteins, which are vital for countless biological activities. Thus, understanding how RNA operates in protein synthesis is crucial for students, researchers, and professionals alike.
RNA's function in protein synthesis mainly revolves around three types: messenger RNA (mRNA), ribosomal RNA (rRNA), and transfer RNA (tRNA). Each of these RNA types contributes uniquely to the synthesis process. The interaction between these RNA molecules forms a complex network that ensures proteins are synthesized accurately and efficiently.
Translation Overview
Translation is the process by which the sequence of nucleotides in mRNA is converted into a sequence of amino acids, forming a protein. This occurs in the ribosomes, where rRNA and tRNA play critical roles.
The steps in translation can be divided into three main phases:
- Initiation: The ribosome assembles around the mRNA. The first tRNA, carrying an amino acid, pairs up with the start codon on the mRNA. This signals the beginning of translation.
- Elongation: The ribosome travels along the mRNA, facilitating the binding of tRNA molecules to their corresponding codons. Each tRNA carries a specific amino acid, which is added to the growing polypeptide chain. The process continues until a stop codon is reached.
- Termination: The completed polypeptide is released from the ribosome when a stop codon is encountered. This marks the end of translation, resulting in a newly synthesized protein.
"The precision of translation determines the functionality of proteins, impacting cellular structure and function directly."
Codon Recognition and Amino Acid Translation
The codon recognition process is key in translation. Each codon—composed of three nucleotides—corresponds to a specific amino acid. For instance, the codon AUG codes for the amino acid methionine, which also serves as the start signal for translation.
The tRNA molecules possess a specific three-nucleotide sequence known as the anticodon. This anticodon is complementary to the mRNA codon, allowing for accurate binding.
The correlation between codons and amino acids can be summarized as follows:
- AUG - Methionine (Start)
- UAA, UAG, UGA - Stop Codons
- UUU - Phenylalanine
- GGC - Glycine
This system of coding ensures that the translation process is not only precise but efficient.
In summary, the role of RNA in protein synthesis is indispensable. Each type of RNA has a distinct and necessary function. From initiating translation to ensuring proper amino acid sequences, RNA facilitates the accurate synthesis of proteins that are essential for life.
Regulatory Mechanisms Impacting RNA Production
The regulation of RNA production is a crucial aspect in understanding cellular function. Various mechanisms govern how genes are expressed, influencing both the quantity and timing of RNA synthesis. These regulatory processes ensure that the appropriate genes are activated under specific conditions. This is vital not only for normal cell function but also for responding to environmental changes and developmental cues.
Regulatory mechanisms can be broadly categorized into transcriptional control and post-transcriptional modifications. Each of these plays a significant role in determining the ultimate outcome of gene expression.
Gene Regulation at the Transcription Level
At the transcription level, several factors modulate RNA synthesis. These include transcription factors, enhancer regions, and repressors. Transcription factors are proteins that bind to specific DNA sequences, thereby facilitating or hindering the transcription process. They act like switches that can turn genes on or off, depending on cellular needs.
Essentially, transcription factors are the gatekeepers of gene expression.
Promoters are specific DNA sequences located at the beginning of a gene. They serve as binding sites for RNA polymerase, the enzyme responsible for synthesizing RNA. Enhancers and silencers, located farther away from the gene they regulate, can also influence transcription by looping the DNA to interact with the promoter region via protein-mediated mechanisms.
The interplay between these elements is complex but essential for fine-tuning gene expression, ensuring that cells can adapt their protein outputs based on internal and external stimuli.
Influence of Non-coding RNAs
Non-coding RNAs (ncRNAs) are critical players in the regulation of gene expression. Unlike messenger RNA, which carries coding sequences, ncRNAs do not translate into proteins. Nonetheless, they perform various regulatory functions that are essential for the proper expression of genes.
There are several types of non-coding RNAs, including:
- MicroRNAs (miRNAs): These small RNA molecules can bind to complementary sequences on messenger RNAs, leading to their degradation or inhibition of translation.
- Long non-coding RNAs (lncRNAs): These longer molecules may affect gene expression by interacting with chromatin-modifying complexes, promoting or repressing transcription.
- Small interfering RNAs (siRNAs): They can interfere with the expression of specific genes, contributing to processes like gene silencing.
The impact of non-coding RNAs illustrates how gene expression is regulated not only by the presence of active coding sequences but also through intricate networks of regulation that include these non-coding entities.
In summary, regulatory mechanisms impacting RNA production are multifaceted and involve a dynamic interplay between various factors. Understanding these processes enhances comprehension of how genes are expressed and regulated, reflecting their significance in both normal physiology and disease states.
Implications of RNA Research
The exploration of RNA research holds significant implications across various scientific fields. With the extensive study of RNA's roles in cellular processes, its potential applications become increasingly evident. Researchers are not only unveiling the complex interactions between RNA and proteins but also how these interactions affect gene expression and overall cellular function. In this context, understanding RNA paves the way for advancements in medicine, genetics, and biotechnology.
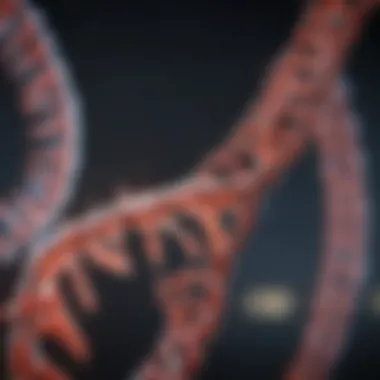
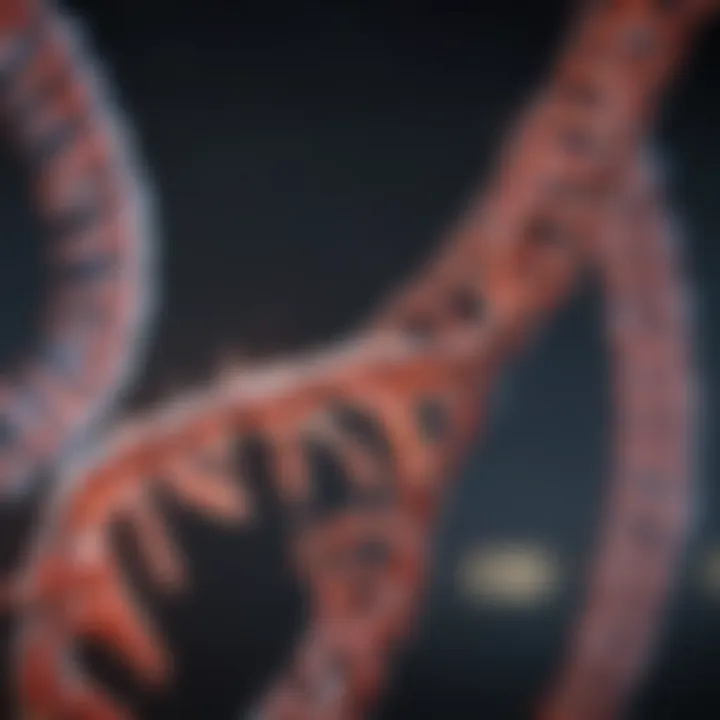
Advancements in Biotechnology
RNA research has led to crucial advancements in biotechnology. For instance, mRNA technology has skyrocketed in popularity due to its role in the development of vaccines. The mRNA vaccines designed to combat COVID-19, such as the Pfizer-BioNTech and Moderna vaccines, exemplify how RNA can be harnessed to offer rapid responses to emerging infectious diseases.
The benefits of mRNA-based therapeutics extend beyond vaccines. They possess the potential to treat genetic disorders through the delivery of correct mRNA sequences to synthesize deficient proteins. Such innovations could reshape targeted treatment strategies, enhancing the precision of therapies against diseases that were previously difficult to treat.
Clinical Applications and Therapeutics
In clinical settings, the implications of RNA research are becoming increasingly apparent. Therapeutics that target RNA molecules, such as antisense oligonucleotides, are gaining traction. These compounds can modulate splicing or promote the degradation of specific RNA molecules, which can be particularly useful in cases of genetic diseases caused by mutations.
Furthermore, the exploration of RNA interference technologies has facilitated the development of novel treatments for various conditions, including cancer and viral infections. Researchers are focusing on small interfering RNA (siRNA) and microRNA (miRNA) as potential therapeutic agents. By manipulating these RNA types, scientists can turn down or silence the expression of harmful genes, offering a new avenue for disease management.
"As RNA research continues to expand, it is likely to unlock further therapeutic avenues that could redefine standard care practices across multiple disciplines."
Overall, the implications of RNA research are profound, providing insights into fundamental biological processes and translating into practical applications that can improve human health. The continuous evolution in this field will further illuminate the path towards innovative treatments and therapies.
Current Trends in RNA Research
RNA research is at the forefront of molecular biology today. It plays a vital role not only in basic biology but also in medical science. Understanding the current trends in RNA research signifies a deeper comprehension of how RNA influences various cellular processes and its potential in developing novel strategies for therapies.
CRISPR and RNA Editing
CRISPR technology has revolutionized genetic engineering, and its application in the realm of RNA editing has opened new pathways for targeted therapies. The ability to edit RNA, rather than DNA, minimizes the risk of permanent genetic changes while allowing for precise alterations in gene expression. This aspect is particularly essential for research aimed at addressing specific diseases, including genetic disorders. CRISPR-based RNA editing holds promise for correcting mutations that affect RNA function without altering the underlying DNA sequence.
- Targeted Modifications: CRISPR enables the targeting of specific RNA molecules for editing.
- Versatility: It has applications across various species, making it widely usable in research.
- Rapid Development: The technology allows for quick iterations, leading to faster advancements in therapy development.
The implications of CRISPR in RNA editing extend to decreasing off-target effects, a concern in traditional genetic alteration techniques.
Exploration of RNA Vaccines
The global focus on RNA vaccines surged during the COVID-19 pandemic. Messenger RNA vaccines, like those developed by Pfizer-BioNTech and Moderna, demonstrate how RNA can instruct cells to produce proteins that trigger an immune response. This platform's adaptability ensures rapid development against new pathogens.
Benefits of RNA vaccines include:
- Speed of Development: Creating RNA vaccines takes less time than traditional vaccine methods.
- Strong Immune Response: They elicit robust immunity, often leading to strong protective responses against diseases.
- Flexibility: RNA vaccine technology can be tailored for various infectious diseases.
The exploration doesn't just stop at infectious diseases; researchers are investigating the potential of RNA vaccines for cancer treatment.
RNA has become a pivotal player in our modern approach to disease prevention and treatment, showcasing its versatility in both therapeutic and preventive realms.
Challenges in RNA Studies
RNA research is crucial to the understanding of many biological processes. However, the study of RNA poses several significant challenges. Addressing these obstacles is essential for advancing our knowledge in molecular biology. The technical limitations and ethical considerations involved in RNA research both play a pivotal role in shaping the current landscape of this field.
Technical Limitations
One of the main obstacles in RNA studies is the technical limitations pertaining to RNA detection and quantification. Unlike DNA, RNA is often present in lower concentrations, making it more challenging to analyze. Traditional methods such as Northern blotting are less sensitive and require large amounts of RNA. Therefore, researchers have increasingly turned to techniques like quantitative reverse transcription PCR (qRT-PCR) and RNA sequencing. These methods offer higher sensitivity, but they also require specialized equipment and expertise, limiting accessibility for some laboratories.
The instability of RNA molecules adds another layer of complexity. RNA is more prone to degradation compared to DNA due to the presence of ribonucleases, enzymes that break down RNA. This necessitates strict sample handling protocols and immediate processing after extraction to prevent loss of material. The complexity of RNA modifications further complicates analyses, as these changes can affect stability and function. Advanced techniques in RNA editing and sequencing must be employed to accurately study these modifications and their implications.
Key Points on Technical Limitations:
- Low abundance of RNA makes detection challenging.
- Traditional methods have limitations in sensitivity and require considerable amounts of RNA.
- RNA molecules are less stable and require careful handling to prevent degradation.
- Advanced techniques can be costly and resource-intensive.
Ethical Considerations in RNA Research
The ethical implications of RNA research must also be carefully considered. As RNA studies often involve human samples, issues related to consent and privacy are paramount. Ensuring that participants fully understand the potential implications of their genetic material being used in research is necessary. Informed consent protocols must be transparent and comprehensive, respecting the rights of individuals who contribute to scientific advancement.
Moreover, the use of CRISPR technology in RNA editing raises ethical concerns about potential misuse for genetic modifications. The ability to alter RNA sequences can lead to significant changes in gene expression, raising questions about long-term impacts and unintended consequences. This includes considerations related to gene therapy and its implications for future generations.
"With great power comes great responsibility." This principle holds especially true in RNA research, as the capability to modify genetic material demands careful ethical considerations.
Important Ethical Considerations:
- Need for transparent informed consent protocols.
- Risks of genetic modifications through RNA editing techniques.
- Potential long-term effects on individuals and populations.
Closure
The conclusion of this article highlights the essential aspects of understanding how DNA is transcribed into RNA. It underscores the complexity of the transcription process, where genetic information is transformed into a medium that can be utilized for protein synthesis. Understanding this transition is not merely academic; it has profound implications for fields such as genetics, molecular biology, and biotechnology.
One specific element to consider is the role of RNA in various cellular processes. The mechanisms of gene regulation, as well as the involvement of non-coding RNAs, are pivotal in understanding cellular behavior. This knowledge can lead to innovative therapeutic strategies for diseases where these processes are disrupted.
The benefits of grasping these concepts extend beyond the laboratory. For students, researchers, and educators, having a firm grasp of transcription enriches their comprehension of modern biology. It adds a layer of depth to how we interpret genetic information and its expression in living organisms.
Moreover, as we stand on the brink of new advancements in RNA technology, from CRISPR applications to RNA vaccines, appreciating the foundational principles of transcription allows each stakeholder to engage intelligently with ongoing research. In essence, the conclusion summarizes not only the key points of the discussion but also the significance of the transition from DNA to RNA in an era of rapid scientific advancement.
Summary of Key Points
- Transcription is a fundamental biological process, converting DNA sequences into RNA.
- RNA serves various functions:** messenger RNA (mRNA)** directs protein synthesis, while ribosomal RNA (rRNA) and transfer RNA (tRNA) play critical roles in translation.
- The transcription process is regulated by numerous factors, including transcription factors and enhancers, which ensure genes are expressed at the right time and place.
- Post-transcriptional modifications such as capping, polyadenylation, and splicing, greatly impact RNA functionality and stability.
- Recent advancements, such as the development of RNA vaccines, underscore the relevance of RNA in modern medicine.
Future Directions in RNA Research
Moving forward, RNA research will continue to expand in several key areas:
- CRISPR and genome editing: Innovations in RNA editing will enhance our ability to modify gene sequences, offering potential treatments for genetic disorders.
- Exploring RNA vaccines: The success of mRNA vaccines for COVID-19 has opened new avenues for vaccine development across various pathogens, including cancer.
- Non-coding RNAs: Ongoing investigations into the roles of non-coding RNAs in gene regulation could reveal more about cellular mechanisms and disease pathways.
- Synthetic biology: The ability to design synthetic RNA molecules may lead to advanced therapeutic techniques in treating complex diseases.
Understanding the transition from DNA to RNA is not just about biological processes; it's about the potential for transformative applications that can impact healthcare and biotechnology. The future of RNA research holds great promise, and staying informed on these developments will be crucial for all involved in the life sciences.