Understanding the Complexities of Complete DNA
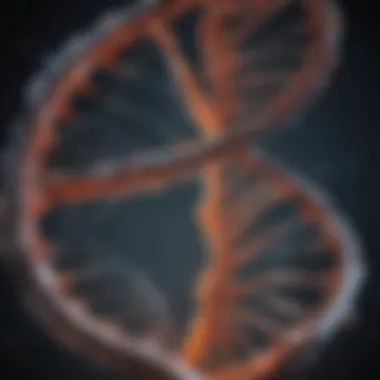
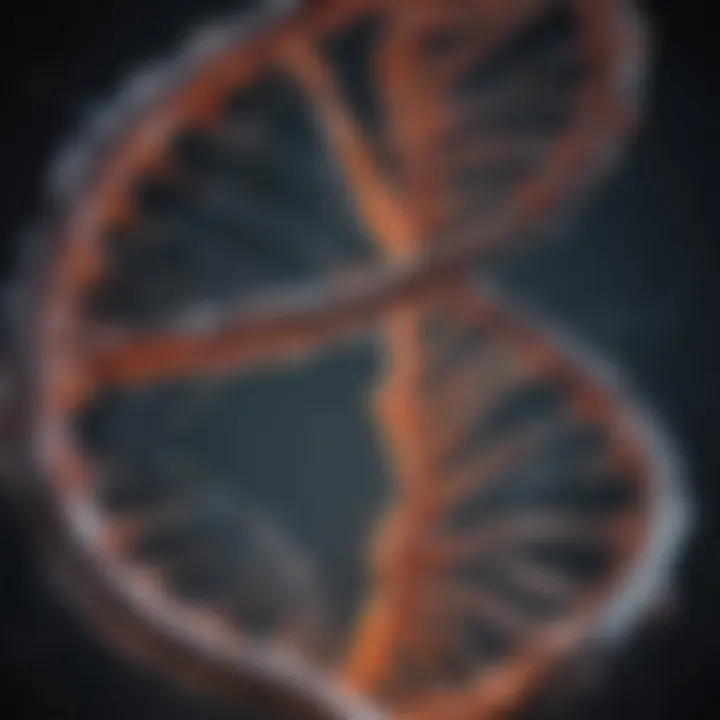
Intro
The realm of complete DNA is often perceived as a labyrinth—filled with twists, turns, and many hidden wonders. It’s not just a simple sequence of genetic information; it encompasses a complex interplay of structures and functions that underpin life itself. Understanding complete DNA goes beyond memorizing sequences; it’s about grasping its implications across various scientific fields, especially in genetics, medicine, and biotechnology.
As we journey through this intricate landscape, we will navigate through key concepts that define the essence of complete DNA. From structural elements like nucleotides and chromatin to their roles in cellular processes, we aim to shed light on the multifaceted nature of this central biological molecule. This exploration also highlights how advancements in DNA analysis techniques have changed the way researchers view genetics and biology.
Moreover, the discussions will extend to how complete DNA influences evolutionary biology and the burgeoning field of personalized medicine. As we unravel these threads, we will provide insight into methodologies employed in DNA research, potential future directions for study, and the profound impact DNA has on science and society.
Buckle up—you’re in for a deep dive into the world of complete DNA!
Foreword to DNA
In the landscape of molecular biology, the understanding of DNA emerges as a cornerstone for numerous scientific inquiries. DNA, or deoxyribonucleic acid, is not merely a biological molecule; it serves as the fundamental blueprint of life. It carries the genetic instructions vital for the growth, development, functioning, and reproduction of all known organisms and many viruses.
The significance of DNA to this article cannot be overstated. The intricate lattice of nucleotides, each corresponding to specific traits and characteristics, invites a deeper exploration into the implications of complete DNA, setting the stage for discussions about genetic research, medicine, and more.
Definition and Structure
At its core, DNA is a macromolecule composed of two long strands that coil around each other to form a double helix. Each strand consists of a sequence of nucleotides, which are the building blocks of DNA. These nucleotides contain three components: a phosphate group, a sugar molecule (deoxyribose), and a nitrogenous base. The four bases—adenine, thymine, cytosine, and guanine—pair specifically (adenine with thymine and cytosine with guanine) through hydrogen bonds, ensuring the stability of the structure.
Understanding the structure of DNA is essential, as it not only elucidates how genetic information is stored and passed on but also opens doors to various biotechnological applications. The ability to replicate itself is an unprecedented feat that allows for genetic continuity across generations.
Historical Context
The journey to our present understanding of DNA has been paved by the efforts of many brilliant minds. In the 1920s, discoveries by scientists like Oswald Avery and his contemporaries pointed to DNA as the carrier of genetic information. However, it wasn’t until 1953, when James Watson and Francis Crick introduced their double-helix model, that DNA truly entered the spotlight.
Their groundbreaking work, guided by Rosalind Franklin’s X-ray diffraction images, unveiled the structure of DNA, laying the groundwork for modern genetics. This discovery not only changed biology but also ignited countless avenues of research, leading to advancements in areas like genetic engineering, forensic science, and personalized medicine. The implications of these historical developments continue to reverberate today, making the study of DNA as relevant as ever.
The Concept of Complete DNA
The concept of complete DNA goes beyond mere sequences of nucleotides; it encompasses a comprehensive understanding of the organism's genetic blueprint. This is not just an academic exercise. Grasping the full extent of what complete DNA entails has profound implications for several fields, such as genetics, medicine, and even evolutionary theory.
Understanding complete DNA means recognizing how every gene and regulatory element contributes to an organism's traits and behaviors. It serves as the foundation upon which the complexities of biology are built. By studying complete DNA, researchers unlock the mysteries of hereditary traits, genetic diseases, and potential therapeutic avenues. Also, knowledge of complete DNA facilitates the development of personalized medicine, enabling tailored treatments that correspond to an individual's unique genetic makeup.
Understanding Complete DNA
Complete DNA refers to the entire set of genetic material present in an organism, often represented by the genome. This includes not only the coding regions, where the genes reside, but also the non-coding regions that play crucial roles in regulating gene expression. To put it simply, complete DNA is like a detailed map that guides the biological processes vital for survival and reproduction.
- Components of Complete DNA:
- Coding Regions: These are sequences that code for proteins. They determine traits by directing the synthesis of proteins that perform various functions in the cell.
- Non-Coding Regions: These regions do not code for proteins but are involved in the regulation and expression of genes. Understanding these regions is crucial in discerning how genes are turned on and off.
- Control Elements: These include enhancers and silencers, which regulate the timing and amount of gene expression.
Scientists use technologies such as whole genome sequencing to map out complete DNA. The capacity to analyze entire genomes allows researchers to identify mutations and variations that might lead to diseases or contribute to evolutionary changes. However, complete DNA isn't entirely straightforward. It comes with challenges, such as understanding the functional significance of every single region and its interactions within the genome.
Differences Between Complete and Partial DNA
To understand the concept of complete DNA fully, one must differentiate it from partial DNA. Partial DNA refers to subsets of the genome that might reflect only specific genes or regions, often used in targeted studies.
- Scope of Analysis:
- Applications in Research:
- Implications for Medicine:
- Complete DNA: Encompasses the total genetic information.
- Partial DNA: Focuses on selected genes or regions, which may lead to incomplete conclusions about an organism's biology.
- Complete DNA: Provides insights into comprehensive genomic interactions and overall organism health.
- Partial DNA: Useful for specific research questions but can miss crucial regulatory elements.
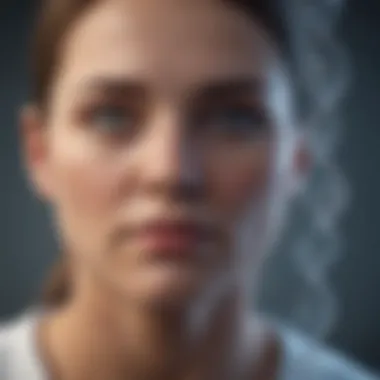
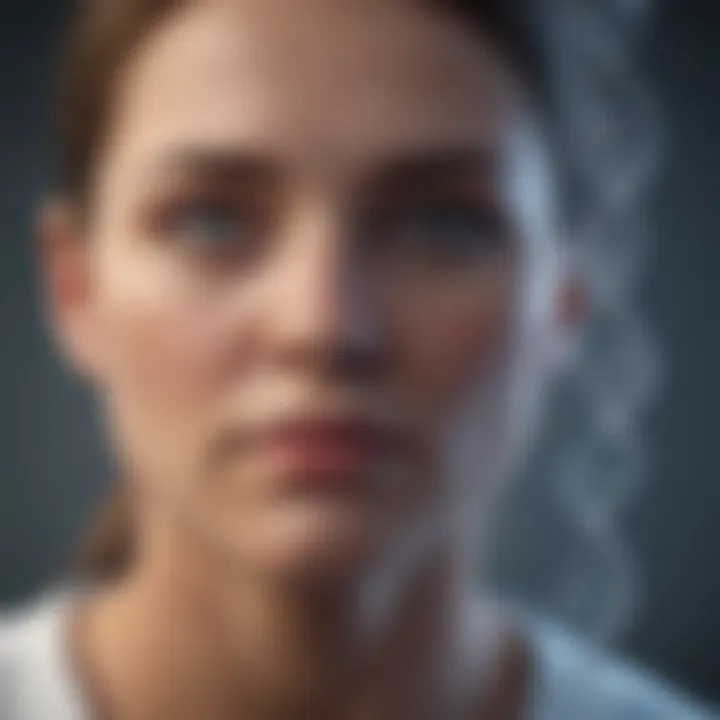
- Complete DNA: Facilitates understanding of genetic predispositions, leading to preventative and personalized healthcare.
- Partial DNA: May inform treatment for specific conditions but lacks a holistic view of patient genetics.
"To uncover the secrets of life itself, knowing the whole story is paramount," as echoed by many leading geneticists in the field.
Methods of DNA Sequencing
Understanding DNA sequencing methods is crucial, as they serve as the backbone for exploring genetic blueprints. Each technique comes with its own unique advantages and challenges. It's not just about knowing the sequence; it's also about recognizing how these methods shape our approaches in genetic research, medicine, and beyond. The significance of these methods cannot be overstated, as they pave the way for innovations in biotechnology, allow personalized treatments for patients, and even help us trace our ancestral roots.
Traditional Sequencing Techniques
Traditional sequencing techniques, like Sanger sequencing, have laid the groundwork for DNA analysis as we know it today. Developed by Frederick Sanger in the 1970s, this method involves the selective incorporation of chain-terminating dideoxynucleotides during DNA replication. While it has served researchers well over the decades, it’s often considered time-consuming and less efficient for large-scale projects.
One notable characteristic of Sanger sequencing is its accuracy, often reaching a fidelity of over 99%. However, it comes with a price; the process can only analyze short sequences, typically up to 1000 base pairs. Despite these limitations, it's still a favorite for smaller-scale studies or for verifying results obtained through other methods.
In essence, traditional sequencing can be viewed as the reliable old workhorse of genetic research. Many labs still employ it, especially when high accuracy is crucial. It's important to understand how the foundational techniques like Sanger play a role in validating the results obtained from newer technologies.
- Advantages of Traditional Sequencing:
- Limitations of Traditional Sequencing:
- High accuracy
- Ideal for small-scale projects
- Well-established protocols
- Time-consuming
- Limited to short sequencing lengths
"While newer technologies have burst onto the scene, traditional methods still hold significant weight in the landscape of DNA analysis."
Next-Generation Sequencing
Next-generation sequencing (NGS) revolutionizes the field of genetics by enabling the rapid sequencing of entire genomes. Unlike traditional methods, NGS allows researchers to decipher vast amounts of data quickly and cost-effectively. With NGS, simultaneous sequencing of multiple DNA fragments occurs, leading to an unprecedented increase in throughput.
The implications of NGS are profound. It empowers researchers to undertake population-level studies, unveiling genetic variances that can lead to deeper insights in evolutionary biology and personalized medicine. Through techniques like Illumina sequencing or Ion Torrent, large sets of DNA data can be processed in a fraction of the time it would take with traditional methods.
Yet, this rapid capability doesn't come without challenges. NGS generates a wealth of data, which necessitates sophisticated bioinformatics tools to analyze and interpret the results effectively. Moreover, while it offers spectacular coverage, the accuracy can dip in complex genomic regions.
- Advantages of Next-Generation Sequencing:
- Challenges of Next-Generation Sequencing:
- High throughput
- Cost-effective for large-scale projects
- Capability to sequence entire genomes
- Data complexity demands advanced analysis
- Potential drop in accuracy in certain genomic areas
Ultimately, both traditional and next-generation sequencing techniques play collaborative roles in advancing genetic research. Understanding their strengths and weaknesses helps the scientific community make informed decisions about which methodologies to employ for specific research questions.
Applications in Genetic Research
Understanding the complexities of complete DNA is crucial for various applications in genetic research. By analyzing complete DNA sequences, researchers can uncover insights that expand our understanding of biological systems, leading to advances in health, agriculture, and conservation. The relevance of exploring DNA isn’t just academic; it impacts real-world applications that shape medicine and environmental practices.
Genomic Studies
Genomic studies harness the power of complete DNA to analyze entire genomes, revealing how genes interact and influence traits. This approach is fundamental for several reasons:
- Disease Association: By mapping entire genomes, scientists identify genetic variations that may predispose individuals to certain diseases. For instance, understanding the complete DNA of individuals with cancer helps pinpoint mutations linked to tumor growth. Researchers can then follow up with targeted therapies, modifying treatment plans based on individual genetic profiles.
- Population Genetics: Complete DNA analysis also provides a window into human evolution and migration patterns. By comparing the genomes of different populations, researchers gain insight into how environmental factors have shaped genetic diversity. This information can be essential for understanding past human behavior and migration, lending context to current health disparities.
- Functional Genomics: Scholars can utilize complete DNA to detail the roles of various genes and understand their functions within the larger biological context. This knowledge contributes to breakthroughs in fields like biotechnology, enabling the development of genetically engineered crops that can withstand diseases, pests, or extreme weather.
Role in Evolutionary Biology
The importance of complete DNA extends into the realm of evolutionary biology, where it serves as a key to unlocking nature's intricate puzzles. Recognizing how organisms evolve through their DNA is essential for multiple reasons:
- Phylogenetics: Analyzing complete DNA equips scientists to construct phylogenetic trees, visualizing the relationships between species based on genetic similarities and differences. This clarity enhances our understanding of common ancestry and the evolutionary processes that shape life.
- Adaptation Studies: Evolutionary biologists can observe how specific genes react to environmental pressures, helping to explain how species adapt over generations. For example, studying the DNA of polar bears provides insights into the adaptive traits that have allowed them to thrive in harsh, cold environments.
- Conservation Genetics: Complete DNA supports biodiverse conservation efforts, allowing researchers to understand the genetic health of endangered species. By identifying genetic bottlenecks, scientists can develop strategies to bolster populations, ensuring species resilience against external pressures such as habitat loss or climate change.
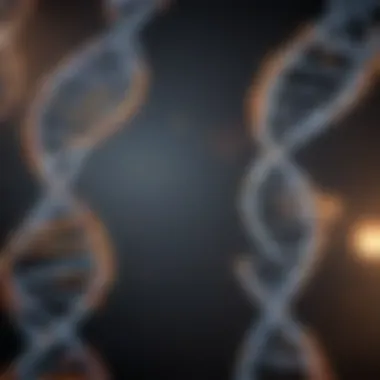
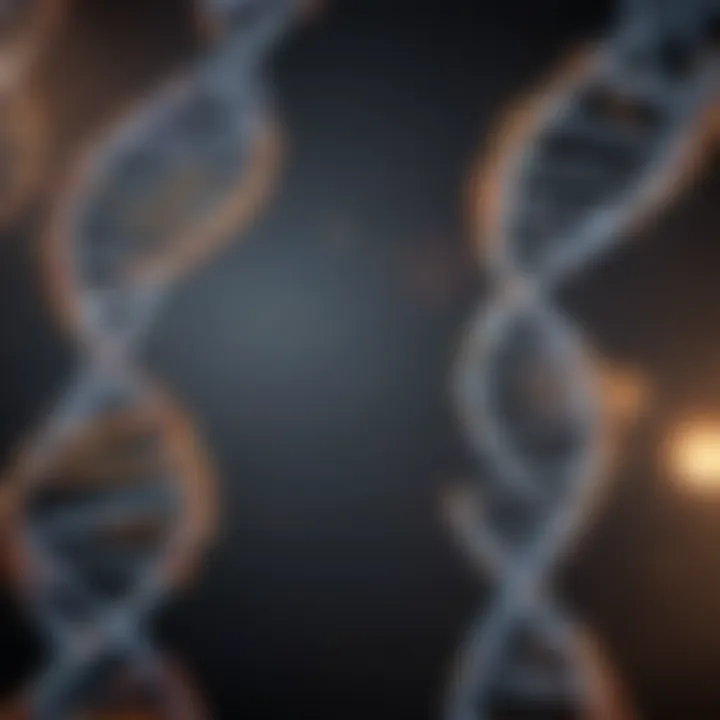
"Understanding the role of complete DNA in evolutionary pathways allows us to grasp not just where we come from, but where we might be headed."
In summary, the applications of complete DNA in genetic research are expansive, touching on everything from personalized medicine to conservation efforts. Through genomic studies and evolutionary insights, we garner knowledge essential for addressing contemporary challenges in health and biodiversity. This intricate dance of genes, based on complete DNA analysis, ultimately shapes the future of life sciences and beyond.
Biotechnology and Complete DNA
Biotechnology has revolutionized the way we understand and manipulate life at the molecular scale. At the center of this revolution lies complete DNA, which provides a comprehensive blueprint for all living organisms. This section unpacks the vital relationship between biotechnology and complete DNA, revealing the innovative techniques and potential applications that arise from this connection.
Importance of Complete DNA in Biotechnology
The central role of complete DNA in biotechnology cannot be overstated. It serves as the foundational element from which a myriad of biotechnological applications emerge. By harnessing complete DNA sequences, scientists can engineer living systems to serve various purposes in medicine, agriculture, and environmental science. Here are some specific elements where complete DNA plays a paramount role:
- Precision: Complete DNA sequences allow for more accurate genetic modifications, leading to effective outcomes in genetic engineering and synthetic biology.
- Diversity: With a full genome, researchers can identify and utilize genes from diverse organisms, expanding the toolbox available for biotechnological applications.
- Analysis: Whole genome sequencing facilitates understanding genetic variations among populations, which is essential for both therapeutic areas and ecological studies.
Benefits of Biotechnology Using Complete DNA
The integration of complete DNA in biotechnology brings notable benefits, including:
- Enhanced Crop Yields: Through genetic modifications based on complete DNA information, crops can be engineered to withstand pests and diseases, thereby improving food security.
- Personalized Medicine: In healthcare, understanding the complete DNA sequences of individuals can lead to tailored treatments based on genetic predispositions, something traditional medicine struggles to achieve.
- Disease Prevention: Advanced methods of genetic engineering can lead to the creation of vaccines and therapies that are tailored to effectively combat specific pathogens.
"The future of biotechnology is deeply intertwined with advancements in our understanding of complete DNA."
Genetic Engineering
Genetic engineering is a cornerstone of biotechnology, enabling the direct manipulation of an organism’s genes. With complete DNA sequences at their disposal, scientists can interact with the genetic architecture of organisms in unprecedented ways.
In practice, this means researchers can:
- Insert Genes: Desired genes can be inserted into an organism’s genome, giving it new characteristics, like drought resistance in plants or increased insulin production in bacteria.
- Edit Genes: Techniques such as CRISPR allow for precise cuts to an organism's DNA, enabling modification of specific traits. This leads to innovations such as gene therapies for genetic diseases.
- Remove Genes: Unwanted or harmful genes can be deleted, potentially eradicating negative traits that could affect survival.
The implications of these manipulations are profound and far-reaching. Genetic engineering shapes industries from agriculture to pharmaceuticals, paving the way for sustainable practices and medical advancements.
Synthetic Biology
Synthetic biology encompasses a broader spectrum of innovation, combining principles from biology and engineering to create new biological systems that are not found in nature. This is where complete DNA becomes even more pivotal.
Through synthetic biology, scientists can:
- Design New Organisms: By utilizing complete DNA sequences as templates, researchers can construct organisms that perform specific functions, such as bacteria that produce biofuels or algae that capture carbon dioxide from the atmosphere.
- Create Genetic Circuits: Complete DNA information enables the engineering of genetic circuits that can respond to environmental stimuli, opening doors to smart biological devices that can produce medicine on-demand or respond to pollution levels.
- Synthesize Essential Compounds: From vitamins to pharmaceuticals, synthetic biology aims to manufacture complex compounds by reprogramming microorganisms, making the production process more efficient and cost-effective.
As synthetic biology continues to evolve, the implications for healthcare, environmental sustainability, and industrial processes are profound. The prospect of designing living systems to address global challenges—like disease, food shortages, and climate change—is not just exciting but necessary in our rapidly changing world.
Personalized Medicine and Complete DNA
Personalized medicine has become a buzzword in the medical field, but what does it really mean in connection with complete DNA? This approach aims to tailor medical treatment to the individual characteristics of each patient, moving away from the one-size-fits-all model of healthcare. By leveraging complete DNA analysis, healthcare professionals can finely tune therapeutic strategies to optimize their effectiveness. The intertwining of personalized medicine and complete DNA is crucial due to its ability to enhance patient outcomes, reduce adverse effects, and better address the diverse genetic makeup of individuals.
The role of complete DNA in personalized medicine is multifaceted. When doctors have complete access to a patient's DNA, they can identify specific genetic mutations that may influence how a patient metabolizes medication. For example, in cancer treatment, a physician may analyze tumor DNA to determine which targeted therapies are likely to work best. This not only increases the chance of a successful outcome but also minimizes unnecessary treatments that may harm the patient.
Tailored Treatment Approaches
Tailoring treatment approaches is where personalized medicine shines brightest. With complete DNA sequencing, physicians can develop customized therapies based on a patient's unique genetic profile. This practice has been particularly transformative in oncology. Consider the example of using genetic markers to guide the choice of chemotherapy drugs; some drugs work effectively for certain mutations but can be entirely ineffective for others. By focusing on the complete genetic landscape, practitioners can pinpoint therapies that align specifically with the biological characteristics of the tumor.
Another area that benefits from this individualized approach is pharmacogenomics—the study of how genes affect a person's response to drugs. By analyzing the genomic data, doctors can predict how well a patient will respond to a medication. For instance, a patient with a specific genetic variant may metabolize a drug more slowly, leading to a higher risk for side effects. In these cases, adjustments can be made to either the dosage or the drug type being administered, enhancing both safety and efficacy.
Ethical Considerations
As with any innovation in medicine, the embrace of personalized approaches rooted in complete DNA sequencing raises vital ethical questions. Patient privacy is a significant concern. With the potential for sensitive genetic data to be misused or mishandled, establishing strong frameworks for data protection is paramount. Patients must feel confident that their genetic information is kept secure and used strictly for appropriate medical purposes.
Moreover, there's the matter of consent. Patients should be fully informed about how their data will be used and the implications of their genetic information. This responsibility falls on healthcare providers and genetic counselors to ensure that patients understand the breadth of genetic testing and its consequences, both positive and negative.

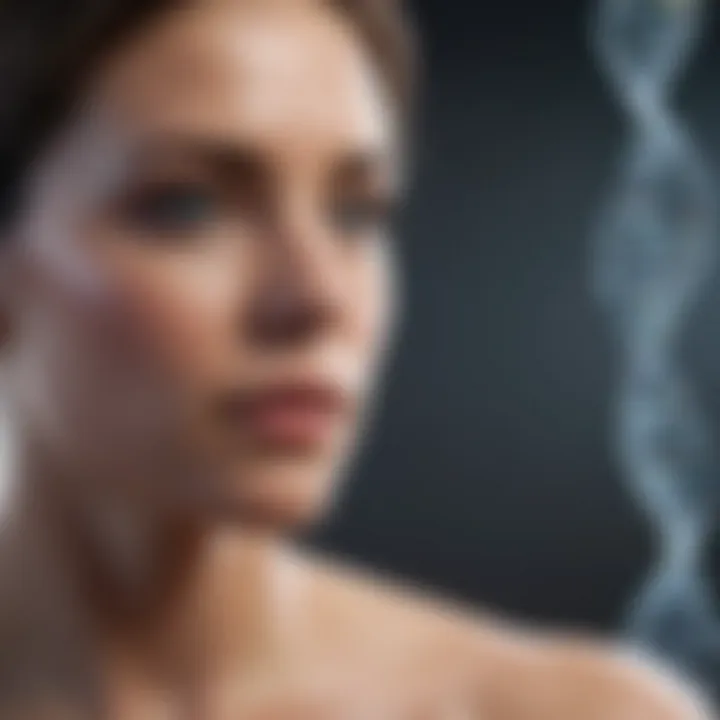
There's also the risk of creating a divide between those who can afford genetic testing and personalized treatments, and those who cannot. As this field progresses, it's crucial for stakeholders to advocate for equitable access to services and ensure that the benefits of personalized medicine are available to all, not just a select few.
With the landscape of healthcare rapidly evolving, personalized medicine, powered by complete DNA analysis, holds immense promise. However, its successful integration into practice depends not just on scientific advancements but also on addressing these ethical and social dimensions.
Challenges in DNA Research
The journey into the depths of DNA research is not without its own set of hurdles. As we explore the realms of complete DNA, it becomes clear that addressing the challenges in this field is crucial for driving progress and understanding the multifaceted nature of genetics. From technical limitations to societal impacts, these challenges pose significant considerations that could either propel or hinder advancements.
Technical Limitations
Despite remarkable strides in technology, researchers often find themselves grappling with technical limitations that can complicate the analysis of complete DNA.
- One of the major issues is the complexity of the human genome. At over three billion base pairs, analyzing and effectively interpreting this colossal data set can feel like looking for a needle in a haystack. The sheer volume of data not only places a strain on computational resources but also limits the pace at which researchers can draw conclusions.
- Additionally, current sequencing methods, while advanced, still face accuracy issues. Errors during sequencing can lead to misinterpretations, which in turn can affect downstream applications such as genetic counseling and precision medicine. Such inaccuracies can potentially result in misguided treatments, emphasizing the need for reliable techniques.
- Many researchers also contend with the limitations of existing bioinformatics tools, which can be inadequate for the expansive datasets generated by next-generation sequencing. Without appropriate analytical tools, extracting meaningful insights becomes a daunting task, often leading to lost opportunities for understanding disease mechanisms.
Societal Impacts
While technical challenges are at the forefront, we cannot ignore the societal implications intertwined with DNA research.
- One major impact stems from ethical concerns regarding genetic data. As researchers delve deeper into complete DNA studies, issues related to data privacy and informed consent become paramount. People are understandably apprehensive about how their genetic information might be used or misused, particularly in light of rising genetic testing services. Trust is essential in the research landscape, and any breach could have far-reaching consequences.
- The societal impact of genetic information extends beyond individuals, affecting entire communities. Disparities in access to DNA testing and treatment can exacerbate existing health inequalities. Those with limited resources may find themselves at a disadvantage, unable to access cutting-edge treatments that depend on complete DNA analysis. This should stir a conversation on how to ensure equitable access to beneficial discoveries in genomics.
- Furthermore, there’s a growing concern about genetic discrimination. Whether in employment or insurance, the potential for individuals to be treated differently based on their genetic information is a pressing issue. Laws and regulations surrounding genetic discrimination must evolve, providing adequate protection without stifling the innovative spirit of DNA research.
"The power of DNA research lies not just in its scientific potential but also in the ethical and social responsibilities it entails."
In closing, addressing the challenges in DNA research is a multifaceted endeavor that requires both technological advancements and a sensitivity to societal implications. As we continue to push the envelope in our understanding of complete DNA, it’s critical to remain aware of these challenges. With a proactive and informed approach, we can turn hurdles into opportunities, guiding the future of genetic research towards a more insightful and inclusive path.
Future Prospects in DNA Studies
The trajectory of DNA research is shaping an exciting horizon for genetic understanding and application. As we unravel the intricacies of complete DNA, numerous pathways unveil a wealth of knowledge that stands to revolutionize various fields, especially genetics, biotechnology, and personalized medicine. Exploring these prospects not only highlights the scientific benefits but also raises questions about ethics, accessibility, and implications for society as a whole.
Advancements in Sequencing Technologies
The landscape of DNA sequencing technologies is changing faster than a cat on a hot tin roof. With each passing year, innovative methods give researchers new tools to explore DNA. One of the most significant advancements is the refinement of nanopore sequencing. Unlike traditional methods, which mostly rely on predetermined fragments, nanopore technology allows for the reading of long strands sequentially. This means that we can now stitch together a more complete picture of the genetic code more efficiently than ever.
Moreover, advancements in computational biology have amplified the impact of sequencing. Algorithms have become increasingly sophisticated, enabling researchers to sift through massive datasets swiftly. Harnessing machine learning tools, scientists can now identify patterns and anomalies in genetic information that were previously invisible. This dual-layered approach—combining real-time sequencing with advanced data analysis—offers the potential for breakthroughs that can change our understanding of various diseases.
"The future of DNA studies is not just in sequencing, but in making sense of the data that comes from it."
Potential Discoveries in Genetics
The promise of complete DNA goes far beyond mere mapping; the potential discoveries stretch from the depths of personalized medicine to flickers of insights into human evolution. As we dive deeper, one of the most pressing questions includes how genetic variations affect individual responses to treatments. For instance, fully sequenced genomes can allow for tailored therapies, minimizing side effects and enhancing efficacies based on one’s genetic makeup.
Equally, understanding genomic differences can provide clues into the mechanics of inherited conditions. Think of it as a treasure hunt, where each piece of DNA may hold the secret to unlocking better management strategies and potential cures for genetic disorders. Furthermore, as scientists gain access to diverse populations and unique genetic backgrounds, we might start to unravel the environmental interactions that shape health outcomes, shedding light on subjects like epigenetics.
In summation, the future is not just bright; it’s illuminating pathways full of possibilities. Whether through enhanced sequencing technologies or groundbreaking discoveries, the implications of complete DNA stretch across multiple domains, blending science with real-world applications. Embracing this journey means recognizing both the intricate specifics and the broader societal impacts as we tread carefully into uncharted territory.
Ending
In the tapestry of genetic sciences, the concept of complete DNA emerges as a focal point, weaving together various strands of inquiry and discovery. Its significance cannot be understated, especially in an era where the implications of genetic research are paramount. Complete DNA is not merely a collection of nucleotides; it's the very blueprint that guides the development and functioning of life. Understanding its structure, function, and potential applications is vital for numerous stakeholders, ranging from researchers to medical professionals.
Summarizing the Importance of Complete DNA
The importance of complete DNA lies in several critical areas:
- Foundation for Genetic Research: It serves as an essential reference point in understanding genetic variation, disease mechanisms, and inheritance patterns.
- Advancements in Medicine: The influence of complete DNA in personalized medicine cannot be overlooked. This approach offers tailored treatment plans based on an individual's genetic makeup, optimizing efficacy and minimizing side effects.
- Biotechnological Innovations: In the realm of biotechnology, complete DNA is instrumental in genetic engineering and synthetic biology. These fields are at the forefront of creating solutions to global challenges, from food security to disease resistance.
Moreover, the advancements in sequencing technologies continually enhance our ability to decode complete DNA more efficiently and accurately. Resources such as Wikipedia, Britannica, and forums like Reddit provide a wealth of information that can deepen our understanding of these developments.
Final Thoughts on Future Research
As we stand on the precipice of genetic exploration, the future of research surrounding complete DNA looks promising yet complex. Key considerations include:
- Ethical Implications: With power comes responsibility. The ability to manipulate complete DNA raises ethical questions about privacy, consent, and the potential for misuse in areas such as gene editing.
- Continuous Learning: The field of genomics is ever-evolving. Future discoveries could reshape our understanding of genetics, evolution, and even disease.
- Collaborative Efforts: Interdisciplinary collaboration will be crucial in unlocking the mysteries held within complete DNA. Partnerships among biologists, bioinformaticians, and ethicists will pave the way for holistic approaches to genetic research.