Understanding Ultraviolet Spectrophotometers: Principles and Applications
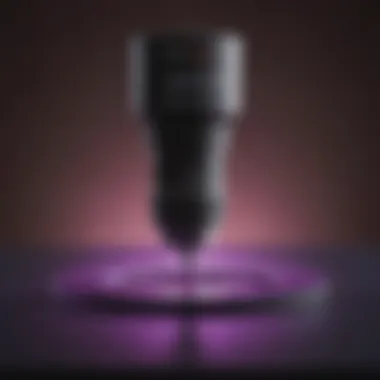
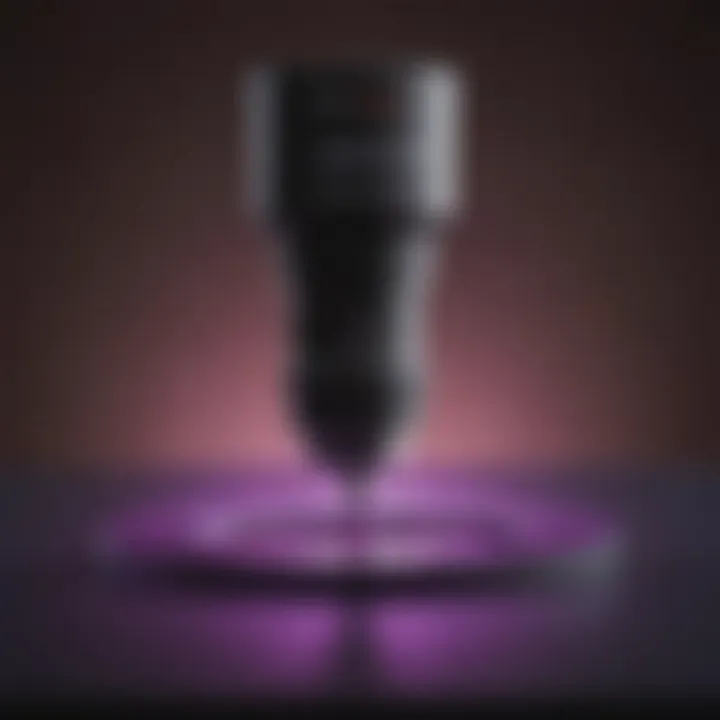
Intro
Ultraviolet spectrophotometry is a powerful technique often employed in various fields of science. By measuring the absorbance of ultraviolet light by a sample, this method provides pivotal insights into the chemical composition and properties of substances. As researchers and educators seek to leverage the capabilities of ultraviolet spectrophotometers, understanding their underlying principles and practical applications becomes critical. This article aims to navigate the terrain of ultraviolet spectrophotometry, shedding light on its multifaceted utility across numerous scientific disciplines.
The rising tide of interest in this technique can be attributed to its versatility. From chemical analysis in pharmaceuticals to quality control in food industries, the applications are as diverse as they are essential. Moreover, with technological advancements, these instruments have become more sophisticated, allowing for better accuracy and broader application ranges. Just as a musician tunes their instrument for optimal performance, calibrating a spectrophotometer ensures that the data obtained is both reliable and reproducible.
In this exploration, we shall delve into the various types of ultraviolet spectrophotometers, highlight significant principles of operation, and examine practical applications. By doing so, we provide a comprehensive framework for both students and seasoned professionals, enabling them to harness the full potential of this technology.
"The beauty of science often lies in its complexities – understanding them is the first step toward innovation."
By providing a clear narrative and organisation, we hope to illuminate the pathway to mastering ultraviolet spectrophotometry, leading to better practices in research and industry.
Prelude to Ultraviolet Spectrophotometry
Ultraviolet (UV) spectrophotometry is a vital tool in the field of analytical chemistry and various scientific disciplines. By using UV light to measure the absorption characteristics of substances, researchers can derive critical information about the chemical composition and concentration of samples. This introductory section serves as a foundation to grasp why understanding UV spectrophotometry is essential for students, educators, and professionals alike.
Definition and Importance
A ultraviolet spectrophotometer is an instrument that measures how much light a chemical sample absorbs at specific ultraviolet wavelengths. The data obtained can offer insights into molecular structures and interactions, which are crucial in applications ranging from environmental monitoring to pharmaceutical development. This technology is often the backbone of qualitative and quantitative analysis in many laboratories.
The importance of UV spectrophotometry lies in its versatility and precision. In a world where accuracy is paramount, the ability to analyze unknown samples swiftly and accurately is invaluable. It’s not just about lab work; it extends to industry standards too, where products must meet specific metrics for safety and efficacy. Moreover, understanding the underlying principles can empower future scientific advancements and innovations.
Historical Context
The journey of UV spectrophotometry began in the early 20th century when scientists, with the advancing knowledge of light and optics, started to explore the interactions of UV light with matter. Initially, it was a novel concept, largely theoretical, until practical applications began to reveal themselves. The first tangible spectrophotometers emerged in the 1940s, designed primarily for scientific research.
Over time, advancements in technology opened the door for more sophisticated equipment, allowing for greater sensitivity and accuracy. The introduction of diode array technology marked a watershed moment, enabling multi-wavelength analysis that saved time and enhanced data quality. Nowadays, UV spectrophotometers are ubiquitous in laboratories around the globe, serving as fundamental instruments for identifying compounds and quantifying concentrations, thereby shaping modern scientific research and industrial practices.
"In every corner of the scientific world, UV spectrophotometers stand as silent sentinels of quality, pushing the boundaries of our understanding one measurement at a time."
By exploring both the vast applications and historical significance, readers set the stage for deeper insights to follow. Each facet of UV spectrophotometry adds another layer to the rich tapestry of knowledge that underpins this powerful technology.
Fundamental Principles of Ultraviolet Spectrophotometry
Understanding the fundamental principles of ultraviolet spectrophotometry is crucial for anyone delving into the realm of analytical chemistry and applied sciences. This section focuses on the mechanisms behind how these instruments operate, their capabilities, and the underlying theories that make them instrumental for research and quality control across various fields. By grasping these concepts, one can effectively interpret data, troubleshoot instruments, and enhance application in practice, which is of utmost importance for students, researchers, and professionals alike.
Light Absorption and Transmission
Light absorption is a pivotal process in ultraviolet spectrophotometry. When ultraviolet light passes through a sample, specific wavelengths are absorbed, and others are transmitted. The degree of absorption is indicative of a sample's concentration of particular compounds or elements. For example, if you shine UV light on a solution of a dye, the amount of light that is absorbed directly corresponds with the concentration of that dye in the solution.
Transmittance, on the other hand, refers to the fraction of light that successfully passes through the sample without being absorbed. Mathematically, transmittance (T) is defined as:
[ T = \fracI_0I ]\
where (I_0) is the intensity of the incoming light and (I) is the intensity of the transmitted light. This relationship highlights an essential concept of spectrophotometry: more concentrated solutions will absorb more light, leading to lower transmittance values.
Utilizing these concepts not only facilitates quantitative analysis but also allows researchers to understand the interaction between light and matter, paving the way for further explorations of chemical properties.
Beer-Lambert Law
A cornerstone of spectrophotometry is the Beer-Lambert Law. This fundamental law establishes a linear relationship between absorbance and concentration of a solution. The equation is given as:
[ A = \varepsilon c l ]\
where:
- (A) is the absorbance,
- (\varepsilon) is the molar absorptivity (a constant defining how strongly a substance absorbs light at a particular wavelength),
- (c) is the concentration of the solution, and
- (l) is the path length of the light through the sample.
The law essentially states that absorbance increases with increasing concentration and path length, assuming that the light is of a specific wavelength that the substance can absorb. This principle not only aids in determining unknown concentrations but also serves crucially in quality control processes and research settings, where precise quantification is necessary. Understanding this relationship is fundamental for interpreting the results obtained from a spectrophotometer effectively.
Wavelength Range and Measurement
Ultraviolet spectrophotometers operate within a specific range of wavelengths, typically from 200 nm to 400 nm. This range is significant because it corresponds to the energy levels of electrons in atoms and molecules, allowing for the analysis of various substances, including organic and inorganic compounds. Different substances absorb UV light at different wavelengths, which means that assessing the wavelengths at which a sample absorbs helps identify its chemical composition.
High-performance spectrophotometers can measure absorbance at selected wavelengths, often employing a monochromator to isolate light. Additionally, scanning spectrophotometers can record absorbance across a range of wavelengths to produce spectra, giving a comprehensive picture of the sample's characteristics.
Understanding the specifics of wavelength range and measurement is pivotal for effectively utilizing spectrophotometry, as it underpins the technique's ability to provide nuanced insights into complex mixtures.
"The principles of ultraviolet spectrophotometry form the bedrock upon which countless analyses are based, driving scientific discovery across domains such as chemistry, biology, and environmental science."
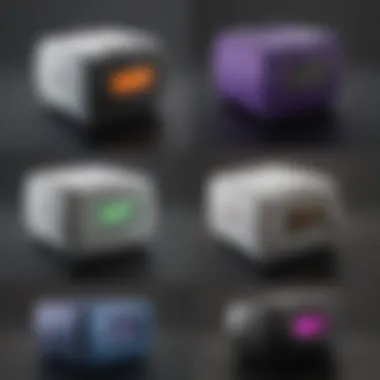
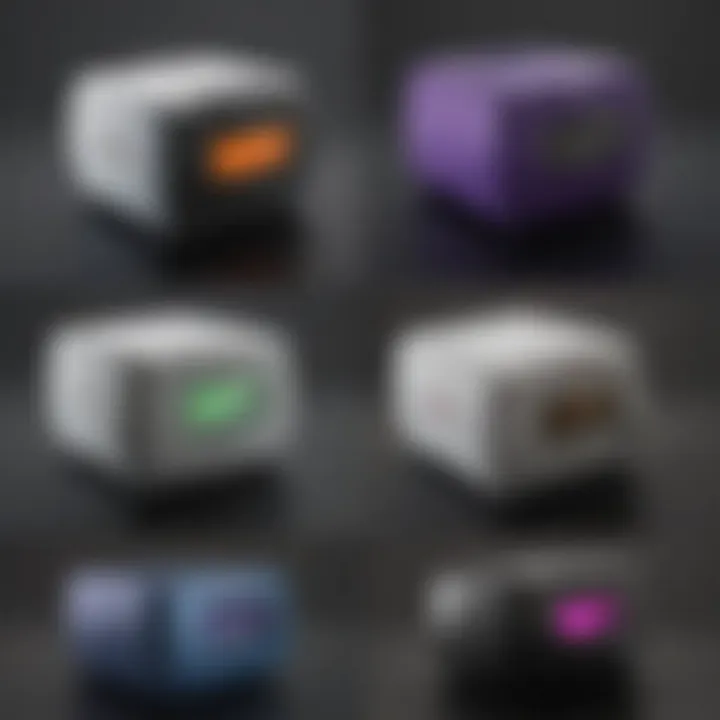
Types of Ultraviolet Spectrophotometers
Understanding the various types of ultraviolet spectrophotometers is crucial in any scientific setting, as they each offer distinct advantages, applications, and precision levels. These devices enable researchers to obtain precise data about the absorbance characteristics of substances, facilitating advancements in fields such as chemistry, biology, and environmental science. By differentiating among the types, users can make informed choices that align with their specific experimental or industrial needs.
Single Beam and Double Beam Spectrophotometers
Single Beam spectrophotometers are known for their simplicity and cost-effectiveness. They function by passing light through a sample, measuring its absorbance, and then comparing it to a blank reference. This method is sufficient for many routine applications but can lead to variability in readings due to fluctuations in the light source.
On the other hand, Double Beam spectrophotometers offer a more sophisticated approach by splitting the light path. One beam goes through the sample, while the other passes through a reference. This design reduces errors linked to source fluctuations, making double beam models highly reliable even in settings demanding strict accuracy.
Diode Array Spectrophotometers
Now, shifting gears to Diode Array Spectrophotometers, these devices are a staple in modern analytical chemistry. Unlike the single or double beam types, the diode array spectrophotometer features an array of photodiodes that allows it to simultaneously read multiple wavelengths. This parallel processing capability enables quicker analyses, which is especially advantageous in high-throughput environments. They are often integrated into laboratories where rapid and accessible spectral data is essential. Their versatility is also noteworthy; they can be employed in diverse fields, from food testing to pharmaceuticals.
Scanning Spectrophotometers
Lastly, we have Scanning Spectrophotometers. These instruments are designed to scan a specified wavelength range, capturing absorbance data across a spectrum. Scanning spectrophotometers are beneficial when examining complex samples with overlapping absorption bands. Researchers rely on these devices for detailed spectral information, helping discern the components within a mixture. However, they may take longer compared to diode arrays, but the richness of data gleaned makes them invaluable for characterizing unknown compounds or verifying substance purity.
"Choosing the right type of ultraviolet spectrophotometer can significantly impact the quality of your analytical results."
In summary, each type of ultraviolet spectrophotometer brings its unique strengths to the table. Whether it’s the cost-efficiency of single beam units, the precision of double beams, the rapid capabilities of diode arrays, or the detailed insights from scanning spectrophotometers, the right choice depends on the specific requirements of your experiments. Being informed about these devices helps maximize the effectiveness of UV spectroscopy in your research or industrial applications.
Components of an Ultraviolet Spectrophotometer
Understanding the components of an ultraviolet spectrophotometer is like pulling back the curtain on a complex yet brilliant mechanism. Each piece plays a critical role in converting light into meaningful data about samples, providing insights necessary for research and industry applications alike. From the light source to the detector, the orchestration of these components creates a cohesive unit that delivers precise measurement of absorbance. This section will delve into these elements, underlining their significance and interdependence in the overall functioning of the device.
Light Source
The light source is the beating heart of a spectrophotometer. Its primary job is to emit ultraviolet light, which is either transmitted through or absorbed by the sample being tested. Common choices for UV light sources include deuterium lamps and mercury vapor lamps. These sources produce stable and consistent output, ensuring that variations in light intensity don't skew the results.
Key considerations when choosing a light source include:
- Output Stability: Fluctuations in light intensity can impact measurement reliability.
- Wavelength Range: Different applications may require specific wavelengths, so the light source should cover the needed range.
- Durability: Given the demand for longevity in laboratory settings, the lifespan of light sources is paramount.
By providing the right quality and range of light, this component ensures that researchers obtain accurate spectrophotometric data for their analyses.
Monochromator
The monochromator’s role is less flashy but equally vital. This component breaks down the light emitted from the source into its constituent wavelengths, selecting a specific wavelength to pass through the sample. Typically, a monochromator uses a system of gratings or prisms to achieve this separation.
In practice, this selection ability is crucial: different compounds absorb UV light at different wavelengths, and the monochromator enables the precise targeting of these absorption peaks. Points to ponder about monochromators include:
- Resolution: Higher resolution lead to more precise measurements; in turn, this benefits both research and quality control processes.
- Speed: Advancements in monochromator technology have led to faster scanning speeds, important for time-sensitive experiments.
Monochromators are critical because they bridge the light source and the detector, making the accurate analysis of samples possible.
Detector
The detector serves as the translator in this entire process. It captures the light that has passed through the sample, measuring the intensity of light that was not absorbed. Detectors can be either photomultiplier tubes (PMTs) or photodiodes, depending on the design of the spectrophotometer.
Considerations regarding detectors include:
- Sensitivity: A highly sensitive detector will pick up even small changes in light intensity, which can be vital for trace analysis in fields like pharmaceuticals and environmental science.
- Response Time: Quick response times are essential for real-time analysis, especially in dynamic experimental setups.
- Dynamic Range: The ability of a detector to measure light intensity across a wide range of values enhances its utility.
A well-functioning detector is essential for ensuring that the data collected is both accurate and meaningful, providing the information researchers need to draw conclusions about their samples.
Cuvette
Last but certainly not least, the cuvette is the container that holds the sample. Usually made from glass or quartz, the material selection depends on the wavelength range of interest, as some materials absorb UV light themselves. The cuvette must also be compatible with the specific application to avoid contamination or interference.
Key factors to consider when selecting cuvettes include:
- Path Length: The distance light travels through the sample. Standard lengths are usually 1 cm, but variations exist for specific applications.
- Material: As noted, the choice of material affects light absorption and must match the spectrophotometer's requirements.
- Cleaning and Handling: Cuvettes must be maintained meticulously to avoid contamination from fingerprints or residues, which can influence measurement outcomes.
In summary, the cuvette is the final frontier where the light meets the sample, making it a pivotal component in the entire spectrophotometric measurement process.
"In spectrophotometry, precision isn't merely a feature; it's the very foundation upon which accurate data and successful research are built."
Each of these components plays a vital role in the functionality of the ultraviolet spectrophotometer as a whole. Understanding how they interconnect enhances the appreciation of this sophisticated tool, ultimately equipping researchers with the knowledge needed to use it effectively.
Applications of Ultraviolet Spectrophotometry
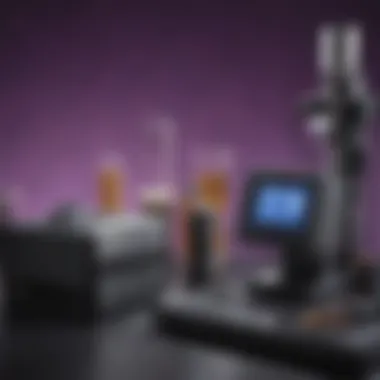
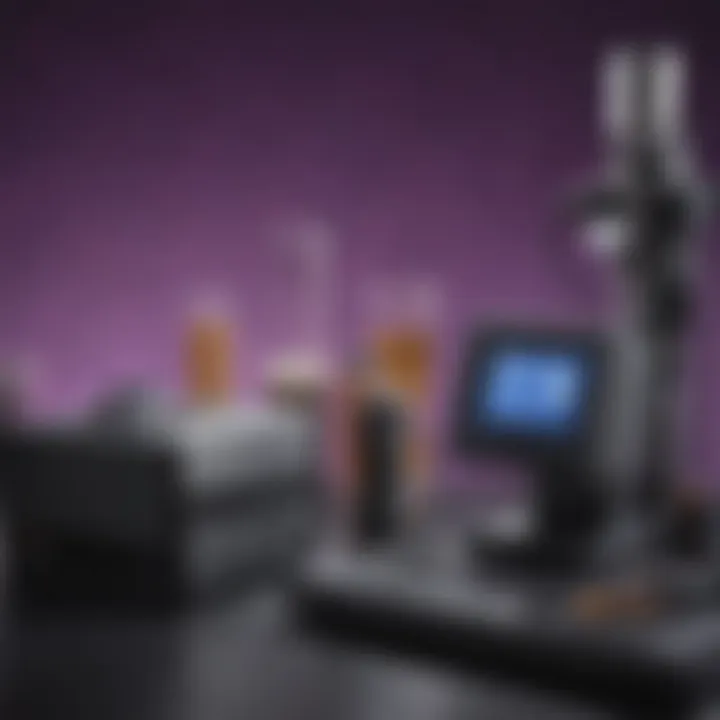
Ultraviolet spectrophotometry stands as a vital tool in various fields, bridging the gap between theoretical knowledge and practical application. The importance of this technique is multifaceted, influencing diverse areas such as chemistry, industry, biological research, and environmental science. By examining how UV spectrophotometry is used, we can appreciate its contribution to science and technology.
Quantitative Analysis in Chemistry
Quantitative analysis in chemistry is where UV spectrophotometers truly shine. This technique allows for precise measurement of concentration in various solutions. Using the Beer-Lambert Law, which relates absorbance to concentration, chemists can determine how much of a particular substance is present in a sample.
For example, a chemist analyzing a solution of phenol can measure its absorbance at a specific wavelength. The data obtained permits the calculation of the phenol concentration, essential in both academic research and practical applications, such as drug formulation.
In practical terms, this capability can significantly influence experimental outcomes, enabling experiments to be conducted with higher confidence. Practical considerations like proper calibration and ensuring the correct cuvette type plays a crucial role in achieving reliable results.
Quality Control in Industry
In industrial settings, UV spectrophotometry is indispensable for quality control. Manufacturers utilize this method to ensure the consistency and purity of products. Industries like pharmaceuticals and food processing rely on these measurements to meet regulatory standards.
With UV spectrophotometers, companies can quickly test raw materials, intermediates, and final products. For instance, a pharmaceutical company might check a batch of antibiotics for purity by analyzing the absorbance peaks corresponding to known impurities. This real-time monitoring can save time and resources, preventing costly product recalls.
A consistent practice of using ultraviolet spectrophotometry in quality control promotes not just reliability but also safety in product consumption, solidifying companies' reputational standing.
Biological and Pharmaceutical Research
In biological and pharmaceutical research, UV spectrophotometry serves as an ally for scientists. Researchers utilize this technique to understand the behavior of biomolecules, including proteins and nucleic acids, revealing intricate details about their structure and function. This understanding can lead to significant advancements in drug development and therapeutic strategies.
For instance, delivering Accurate absorbance measurements of DNA at 260 nm can help researchers evaluate nucleic acid purity, guiding downstream applications in cloning or sequencing.
Moreover, in drug formulation, researchers monitor the stability of pharmaceutical compounds under various conditions. Tracking absorbance changes over time can indicate degradation, guiding necessary adjustments in storage or formulation processes. This insight not only informs laboratory practices but also has real-world implications for patient safety and efficacy.
Environmental Monitoring
Another pivotal application of UV spectrophotometry lies in environmental monitoring. This technique assists scientists in measuring pollutants in water and air, playing a crucial role in assessing environmental health.
For instance, detecting heavy metals in water samples is not just a regulatory requirement; it’s a serious public health concern. By utilizing specific wavelengths, scientists can assess contaminants such as lead or mercury and ensure that levels remain below harmful thresholds. This technology supports ongoing environmental assessments and informs policy-making, emphasizing the technique's relevance beyond the laboratory scope.
Additionally, the application of UV spectrophotometry for monitoring harmful algal blooms in aquatic systems highlights its utility in real-time ecology. By tracking the absorption of specific wavelengths, researchers can gauge the presence of chlorophyll and assess potential risks to marine life and human health.
"The capability of UV spectrophotometry to provide rapid, reliable data is invaluable for making informed decisions in environmental conservation and remediation efforts."
In summary, the applications of ultraviolet spectrophotometry span a broad spectrum of fields. From facilitating quantitative chemical analysis to ensuring product quality in industry, aiding in groundbreaking biological research, and monitoring environmental health, its significance cannot be overstated. As technology continues to evolve, so will the possibilities that accompany this essential analytical tool.
Calibration and Maintenance of Spectrophotometers
Calibration and maintenance of ultraviolet spectrophotometers is pivotal for obtaining accurate and reliable data. In the world of scientific measurements, precision holds the lion's share of importance. A miscalibrated instrument may deliver results that lead researchers down the wrong path. Hence, understanding both calibration procedures and routine maintenance checks is essential for anyone working in laboratories or research facilities.
Regular calibration ensures that the instrument is measuring what it’s really supposed to. Over time, factors like environmental conditions, wear and tear, or component aging can affect the performance of spectrophotometers. A well-calibrated device not only boosts confidence in the data collected but also enhances reproducibility in experiments. Proper maintenance further extends the lifespan of the equipment, ultimately saving costs and minimizing the hassles of unexpected breakdowns.
"An ounce of prevention is worth a pound of cure." This saying rings true in the realm of spectrophotometry, where preventive maintenance practices can significantly mitigate future issues.
Calibration Procedures
Calibration procedures are systematic steps taken to ensure the accuracy of the spectrophotometer. Typically, several key processes are involved:
- Selection of Calibration Standards: Using appropriate standards is crucial. Commonly, potassium dichromate and holmium oxide are employed due to their well-characterized absorption spectra.
- Baseline Adjustment: Ensure that the baseline is correctly aligned with zero absorbance before any measurements. This often requires running a blank sample, usually comprising the solvent without analytes.
- Wavelength Calibration: Verify that the wavelengths delivered by the spectrophotometer match known values. This may be done using spectral lines from a mercury lamp.
- Intensity Calibration: Adjust the instrument for correct absorbance readings across its operational range. Regular checks against standard solutions help in detecting any drift in intensity.
- Documentation: Keeping clear records of calibration runs is not just good practice; it’s often essential for compliance with regulatory standards and audits.
Preventive Maintenance
Preventive maintenance encompasses routine checks and services performed to keep the spectrophotometer in efficient working order. Here are some components that require diligence:
- Regular Cleaning: Dust and residues can accumulate, impacting light paths. Regularly clean optical components, such as cuvettes and mirrors, with appropriate solvents.
- Condition Checks: Frequent inspection of cables, connections, and control panels ensures that any wear or corrosion is promptly identified.
- Component Replacement: Light sources and detectors have finite lifespans. It’s crucial to replace these components according to their usage cycles to ensure consistent performance.
- Software Updates: If the spectrophotometer employs software for running analyses, keeping this updated ensures access to the latest features and bug fixes.
- Performance Audits: Periodic reviews of data outputs compared to expected results can help trace back to potential issues early on, allowing for timely corrective action.
In summary, calibration and preventive maintenance are not just procedural checkboxes; they serve as the backbone of quality and reliability in spectrophotometric data. The initial investment of time and resources pays off significantly in the form of trustable results and sustained equipment longevity.
Interpreting Spectrophotometric Data
Interpreting spectrophotometric data is a crucial aspect of utilizing ultraviolet spectrophotometers. This process helps researchers make sense of the measurements obtained from their samples, translating raw data into insights that can guide further investigations or applications. Each factor involved plays a significant role in comprehending results and their implications. A clear understanding of how to interpret these outputs not only enhances research validity but also ensures the derived conclusions are sound and reproducible.
Understanding Absorbance and Transmittance
When we talk about absorbance and transmittance, we step into the heart of spectrophotometry. Absorbance, denoted as A, quantifies how much light a sample absorbs at a specific wavelength. The formula involves the intensity of light before entering the sample (I0) and the intensity of light after passing through the sample (I):
A = log(I0/I)
The greater the absorbance value, the less light exits the sample, indicating that the sample has absorbed more light.
Conversely, transmittance (T) is the fraction of light that passes through the sample. It's often expressed as a percentage:
T = (I/I0) x 100%
Understanding these two concepts allows researchers and professionals to gauge sample concentration, evaluate purity, and check the performance of chemical reactions. This duality serves as the backbone for quantitative analysis and can be influential in various fields, from pharmaceuticals to environmental science.
Analyzing Spectra
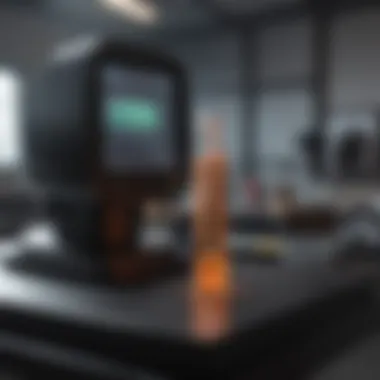
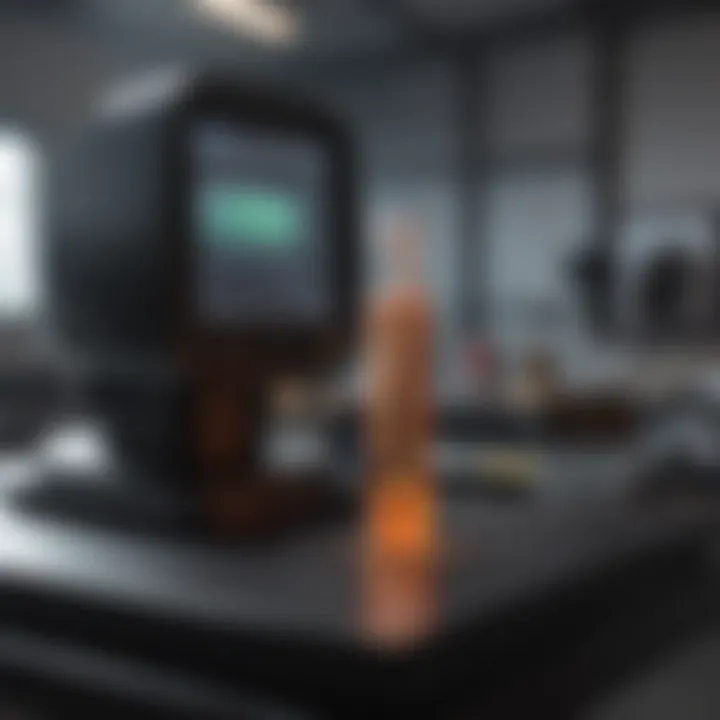
Spectral analysis involves interpreting graphical representations of the absorbance or transmittance data across a range of wavelengths. When a spectrum is generated, each peak and trough depicts certain characteristics of the sample being examined. A good practice is to first identify the wavelengths where absorbance peaks appear, as these typically correspond to specific electronic transitions in the molecules.
For instance, a sharp peak in the ultraviolet region may indicate the presence of a particular functional group or chromophore within the compound. On the flip side, broad peaks can suggest complex interactions or mixtures of compounds. When interpreting these patterns, it’s essential to consider context: factors like solvent choice or molecular concentration can significantly affect the spectra.
"A spectrum tells a story about the interactions of light with matter; the peaks are the narrative threads."
Common Data Analysis Techniques
Once the data has been captured and the spectra analyzed, using data analysis techniques becomes essential to derive meaningful conclusions. Several methods are employed in this context:
- Baseline Correction: This removes background noise and ensures the extracted data truly reflects the sample.
- Peak Integration: This quantifies the area under the peaks in the spectra, allowing for assessments of concentration.
- Deriving Concentration: This utilizes established calibration curves to convert absorbance values into actual concentrations of species present in the sample.
- Statistical Methods: Techniques such as regression analysis help understand relationships within data, providing a basis for predictions and further research validation.
By engaging these methods, researchers not only improve accuracy but also enhance the robustness of their findings. This analytical depth is paramount across diverse scientific fields, including biochemistry, environmental science, and materials analysis, making it indispensable for anyone looking to wield a spectrophotometer effectively.
Limitations of Ultraviolet Spectrophotometry
Ultraviolet spectrophotometry, while a powerful tool in scientific analysis, is not without its drawbacks. The limitations presented by this technique can influence data accuracy, operational efficiency, and overall research outcomes. It's crucial for researchers and educators to be aware of these hurdles, as understanding them can lead to improved methods of application and better interpretation of results.
Interference from Other Compounds
In the realm of UV spectrophotometry, interference from other substances can be a significant roadblock. Often, samples contain various compounds that absorb light within the same wavelength range. This overlap can obscure the signals from the target analyte, leading to misleading interpretations.
For example, consider a scenario where a chemist is analyzing a solution that contains both a desired drug compound and several excipients, which are inactive substances used as a carrier. If one of those excipients absorbs UV light at the same wavelength as the drug, it might lead to an inflated absorbance reading. This makes it difficult to discern the actual concentration of the analyte.
To tackle this issue, employing techniques like chromatography before the spectrophotometric analysis can help isolate the target analyte. This extra step adds time and complexity but affords a clearer picture in the end.
Instrumental Limitations
Instrumental limitations also play a crucial role in the efficacy of UV spectrophotometric measurements. Various factors contribute here, including stray light, wavelength accuracy, and resolution. Stray light refers to light that reaches the detector without passing through the sample, which can lead to inaccurate absorbance readings. It's akin to trying to listen to music in a busy café; the background noise can drown out the melody you're interested in.
Moreover, the resolution of the instrument may affect the ability to discern between closely spaced absorption peaks. If an instrument is not finely tuned, two compounds that absorb light at adjacent wavelengths might not be properly distinguished. This can seriously compromise the data quality.
Aside from the technical aspects, user handling can also introduce errors. Operators must be adept with the instruments and understand their limitations. Regular maintenance and calibration are essential to ensure consistent performance. A poorly maintained spectrophotometer can behave like an unreliable friend—one day it’s solid, and the next, it’s completely off the mark.
"Understanding the limitations of any analytical instrument is as crucial as mastering its methodologies. The two go hand-in-hand for effective application."
In summary, recognizing interference from other compounds and instrumental limitations helps refine the approach to spectrophotometric analysis, paving the path for more robust results.
Future Trends in Ultraviolet Spectrophotometry
The realm of ultraviolet spectrophotometry is continuously evolving, driven by advancements in technology and the ever-increasing demands of various scientific fields. Recognizing the future trends in this area is essential for researchers and practitioners who aim to stay ahead of the curve. From enhanced precision to better integration with other technologies, these trends signal a transformative phase for ultraviolet spectrophotometers that can significantly amplify their application and reliability.
Innovations in Spectrophotometer Design
Spectrophotometer design is experiencing notable innovations that can reshape their functionality and user experience. Modern designs aim to incorporate user-friendly interfaces, allowing even novices to navigate the complexities of spectral analysis. For example, the introduction of touch screens and intuitive software simplifies procedures, lowering the barrier for usage. Moreover, lightweight materials and compact designs are making it easier for laboratories to manage their workspace efficiently.
Consider the integration of microfluidics in spectrophotometers, which enhances the speed and accuracy of measurements by allowing for precise sample handling. This methodology is especially beneficial in high-throughput environments where efficiency is paramount.
Additionally, the possibility of 2D imaging in spectroscopy is on the rise. This allows for faster analysis of samples, and greater detail can be obtained from complex mixtures. The instruments of the future might not just tell you what is present but also visualize how the components interact on a micro-level.
Integration with Advanced Technologies
Another key trend lies in the integration of ultraviolet spectrophotometers with advanced technologies such as artificial intelligence and machine learning. These powerful tools can assist in data analysis, predictive modeling, and even real-time monitoring of experiments. Imagine an AI-driven spectrophotometer that can learn from past experiments and optimize the analysis in future tests automatically.
Moreover, the convergence of ultraviolet spectrophotometry with nanotechnology opens new avenues for research and applications, particularly in fields like biomedicine and environmental science. For instance, nanomaterials enhance light interactions, potentially leading to unprecedented sensitivity and specificity in detection.
Emerging online platforms are also playing a crucial role. By linking spectrophotometers to comprehensive databases, researchers can tap into a wealth of real-time data, improving accuracy in qualitative and quantitative assessments. Collaboration tools, paired with spectrophotometric data, may soon allow remote teams to conduct experiments collectively and effectively.
"The integration of spectrophotometric methods with new technologies not only enhances analytical capabilities but also streamlines research processes, making them quicker and more efficient."
The End
Understanding ultraviolet spectrophotometers is not just for the specialists in the lab; their relevance permeates various scientific sectors. This article has highlighted how this technology plays a pivotal role by aiding in precise measurements and analyses that can lead to groundbreaking discoveries.
Summary of Key Points
- Fundamental Principles: The principles of light absorption and the Beer-Lambert law are central to the understanding of how these instruments function.
- Types of Spectrophotometers: Knowing the distinctions between single beam and double beam instruments, as well as diode array spectrophotometers, helps in choosing the right tool for specific tasks.
- Applications: Ultraviolet spectrophotometers find utility in numerous fields like chemistry, pharmaceuticals, and environmental science. Each application underscores the technology's impact on quality control and research.
- Calibration and Maintenance: Regular calibration and maintenance are essential. This ensures accuracy and reliability in measurements, which are crucial for high-stakes analysis.
- Limitations: Awareness of the potential limitations—like interference from other compounds—allows users to interpret data with a more critical perspective.
- Future Trends: Innovations in design and integration with advanced technologies signal a promising evolution in the functionality of these spectrophotometers.
Final Thoughts on Application and Impact
The significance of ultraviolet spectrophotometry is profound in modern research and industry practices. It not only facilitates essential quantitative analysis but also enhances our ability to monitor and maintain quality across various sectors. For anyone involved in scientific research—from students to seasoned professionals—grasping the implications and applications of this tool can lead not just to better studies but also to more robust findings.
In a world where precision and accuracy are paramount, investing time in understanding these spectrophotometers pays off. Innovating with this technology can pave the way for future discoveries, ensuring that scientific inquiry continues its trajectory towards deeper insights and the intersection of disciplines.
"The right tools can transform ideas into reality; understanding their nuances is what enables innovation."
Hence, this exploration not only emphasizes the existing capabilities of ultraviolet spectrophotometers but also invites readers to consider their implications and future possibilities in diverse scientific endeavors.