Exploring Post-Translational Modifications Using Mass Spectrometry
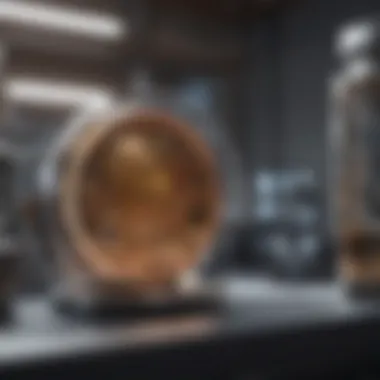
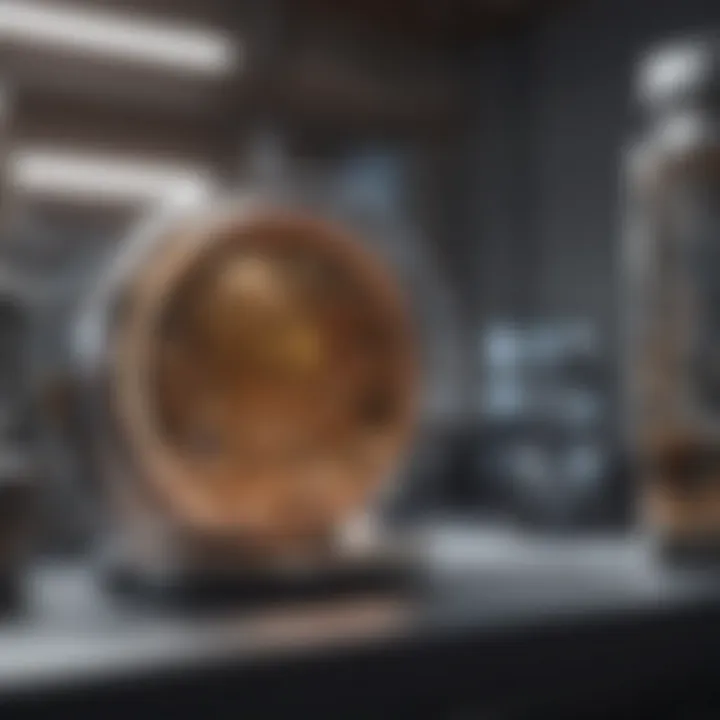
Intro
Post-translational modifications (PTMs) represent an essential aspect of protein biochemistry. They affect protein function, stability, localization, and interaction with other molecules. Identifying these modifications is fundamental for understanding cellular mechanisms and conducting biomedical research. Mass spectrometry (MS) has emerged as a powerful tool for this purpose, facilitating the analysis of PTMs at both qualitative and quantitative levels. Through this article, we will explore the methodologies used in mass spectrometry to examine PTMs, the technological advancements in the field, and the challenges faced by researchers.
Methodology
Overview of Research Methods Used
Research methods for analyzing PTMs through mass spectrometry encompass several stages. Firstly, sample preparation is critical. This involves protein extraction, purification, and sometimes enrichment of PTMs. Following this, specific mass spectrometric techniques are employed to identify and characterize modifications. These include tandem mass spectrometry (MS/MS) for sequencing peptides and detecting specific modifications, as well as matrix-assisted laser desorption ionization (MALDI) and electrospray ionization (ESI) for ion generation.
Data Collection Techniques
Data collection in mass spectrometry involves rigorous protocols to ensure accuracy. The mass spectrometer records the mass-to-charge ratios of ions that are generated from the analyte. These data points are then interpreted to identify PTMs. The common techniques include:
- Peptide mass fingerprinting: This allows the identification of proteins following digestion with specific enzymes.
- Labeling techniques: Isobaric tags for relative and absolute quantitation (iTRAQ) enable relative quantification of proteins across different samples.
- Bioinformatics: Software tools are essential for analyzing raw mass spectrometric data, providing insights into modification sites and quantification.
"Mass spectrometry continues to evolve, providing deeper insights into the complex world of post-translational modifications and increasingly reliable data for researchers."
Future Directions
Upcoming Trends in Research
The future of PTM analysis through mass spectrometry is promising. Emerging trends include:
- Single-cell proteomics: This enables the analysis of PTMs at the single-cell level, offering insights into cellular heterogeneity.
- Integrated omics approaches: Combining mass spectrometry with genomic and transcriptomic data improves understanding of how PTMs influence cellular pathways.
Areas Requiring Further Investigation
Despite progress, challenges remain. Areas that require further inquiry include:
- Standardization of protocols: Variations in methods can lead to inconsistencies in results. Standardized protocols will enhance reliability.
- Biological significance of modifications: More work is necessary to link specific PTMs to their functional consequences in health and disease.
In summary, mass spectrometry is a vital technology for exploring post-translational modifications. By refining methodologies and addressing existing gaps, researchers can continue to gain valuable insights into protein function and regulation.
Prelude to Post-Translational Modifications
Post-translational modifications (PTMs) are critical processes that occur after a protein has been synthesized. They play crucial roles in regulating diverse cellular functions and pathways. Understanding PTMs is vital for researchers in various fields, including biochemistry, molecular biology, and medicine. Improved insights into these modifications can lead to significant advancements in disease understanding and therapy development.
Definition and Importance
Post-translational modifications refer to the chemical modifications that proteins undergo after their initial translation from RNA. These modifications can alter the protein's function, localization, stability, and interaction with other molecules. PTMs are essential for regulating a wide range of biological processes such as signal transduction, immune responses, and gene expression. Their importance is underscored by their roles in various diseases, including cancer and neurodegenerative disorders.
Understanding the complex landscape of PTMs is essential for developing precise drugs, identifying biomarkers, and enhancing diagnostic tools in clinical settings. Moreover, the identification and characterization of these modifications continue to push forward the boundaries of proteomics and systems biology.
Types of Post-Translational Modifications
There are several key categories of post-translational modifications, each with unique characteristics and functions:
- Phosphorylation: This modification involves the addition of a phosphate group, typically to serine, threonine, or tyrosine residues. Phosphorylation can activate or deactivate enzymes and receptors, thus playing a pivotal role in signal transduction pathways. Its dynamic nature makes phosphorylation a fundamental aspect in the study of PTMs.
- Acetylation: This process includes the transfer of an acetyl group to amino acids, primarily lysine. Acetylation can influence protein stability, activity, and interaction with DNA. It is particularly important in gene regulation and metabolic pathways, linking cellular signals to transcriptional outcomes.
- Methylation: This modification primarily occurs on lysine and arginine residues. Methylation affects protein-protein interactions and is crucial in epigenetics, impacting chromatin structure and gene expression. Different methylation states can result in distinct functional outcomes for the modified proteins.
- Glycosylation: The attachment of carbohydrate groups to proteins characterizes this modification. Glycosylation plays a vital role in protein folding, stability, and recognition processes. It can also influence immune responses and cell signaling, making it a significant area of study in various diseases.
- Ubiquitination: This involves attaching ubiquitin molecules to proteins, often signaling them for degradation via the proteasome. Ubiquitination is central to regulating many cellular processes, including the cell cycle, DNA repair, and stress responses. It serves as a vital mechanism for maintaining cellular homeostasis.
Each of these modifications contributes to the diverse functional repertoire of proteins, making them essential to biological systems. By studying these PTMs, scientists can uncover new insights into cellular mechanisms and potentially exploitable therapeutic targets.
Fundamentals of Mass Spectrometry
Mass spectrometry is a powerful analytical technique that plays a crucial role in identifying and quantifying post-translational modifications (PTMs) in proteins. It allows researchers to analyze the mass-to-charge ratio of ions, which offers insights into molecular structure and composition. Understanding the fundamentals of mass spectrometry is essential for navigating its capabilities, especially in the context of PTM analysis. This knowledge enables scientists to strategically choose methods that best suit their research needs, ultimately enhancing the reliability of their findings.
Principle of Mass Spectrometry
The principle of mass spectrometry is based on the analysis of ions. In this process, molecules are ionized, resulting in charged particles that can be manipulated in the presence of electric and magnetic fields. The fundamental idea is to measure the mass of these ions relative to their charge. This measurement allows scientists to determine the mass of different components within a sample. Accurate quantification and structural information are also achievable when interpreting the data from mass spectrometry. The accuracy of these measurements is pivotal when it comes to identifying specific post-translational modifications in proteins.
Mass Spectrometry Components
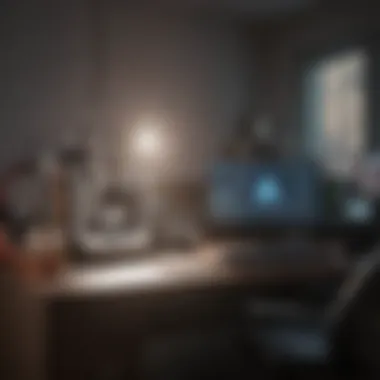
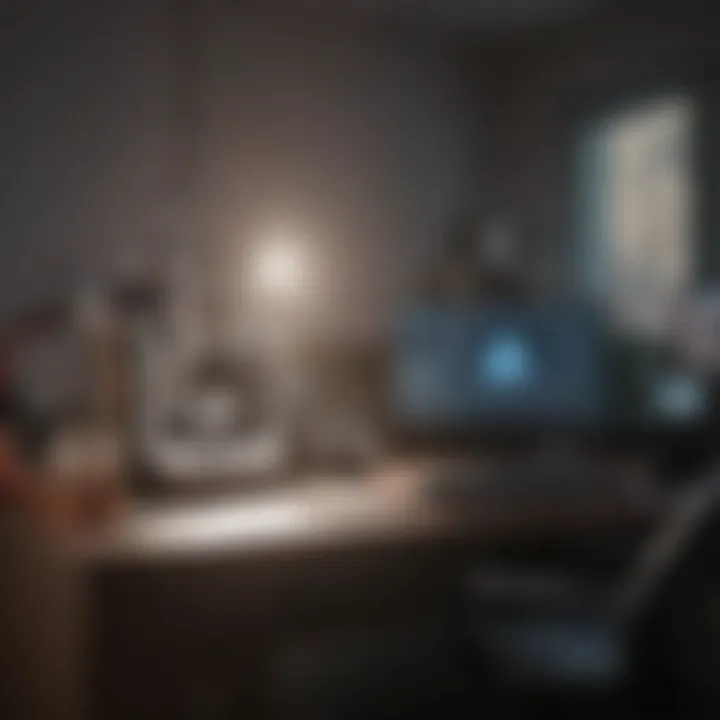
Mass spectrometry consists of several essential components that work together to facilitate the analysis of samples. Each component serves a unique purpose and contributes to the overall effectiveness of the technique in discussing PTM analysis.
Ion Source
The ion source is where the process begins, as it is responsible for converting the sample into ions. Different methods exist for ion generation, such as electrospray ionization and matrix-assisted laser desorption ionization. The key characteristic of an ion source is its ability to create ions efficiently with minimal fragmentation. This efficiency is particularly beneficial in PTM analysis since it preserves the integrity of the protein’s structure. One of the unique features of the electrospray ionization method is its capacity to handle complex mixtures. A disadvantage, however, might be the requirement for specific solvents, which can sometimes complicate the preparation of samples.
Mass Analyzer
Next in the sequence is the mass analyzer, which separates the ions based on their mass-to-charge ratios. There are different types of mass analyzers, such as time-of-flight and quadrupole analyzers. The major benefit of using a time-of-flight analyzer is its ability to provide high-resolution data, which is critical for differentiating between similar masses of modified proteins. However, this high resolution can come at the cost of increased analysis time. Understanding the various options for mass analyzers is fundamental to determining the best approach for accurate PTM identification.
Detector
The final component is the detector, which measures the abundance of ions reaching it after they have been analyzed. Various detectors, such as ion trap and electron multiplier detectors, provide the output data critical for interpretation. A notable characteristic of these detectors is their sensitivity, which allows for the detection of low-abundance ions that may represent rare post-translational modifications. Detecting these rare modifications is vital for understanding their biological significance. One challenge with some detectors may be their dynamic range, which could affect the accuracy of quantification for abundant versus low-abundance molecules.
"The interplay of these components defines the effectiveness of mass spectrometry in revealing the complexities of post-translational modifications."
Through the integration of these key components, mass spectrometry becomes a reliable tool for scientific inquiries into post-translational modifications. Understanding how each function contributes to the whole enhances the validity of research findings in proteomics.
Mass Spectrometry Techniques for PTM Analysis
Mass spectrometry has emerged as a pivotal tool in studying post-translational modifications (PTMs). The techniques used in this field are essential for advancing our understanding of cellular mechanisms. They enable researchers to identify and quantify PTMs with remarkable precision. The integration of mass spectrometry techniques in PTM analysis offers several advantages. These include heightened sensitivity, specificity, and the ability to process complex biological samples.
Shotgun Proteomics
Shotgun proteomics represents a comprehensive approach in mass spectrometry for analyzing proteins and their modifications. In this technique, proteins are digested into smaller peptides using enzymes like trypsin. The resulting peptide mixture is then analyzed by mass spectrometry. The core benefit of this method lies in its ability to capture a broad spectrum of proteins from complex samples, providing insight into the entire proteome.
One significant aspect of shotgun proteomics is its capacity to discover novel PTMs that might be overlooked in other methods. However, it also comes with challenges, particularly in data analysis due to the vast number of peptides generated. The interpretative complexity requires advanced software tools and databases for accurate identification.
Targeted Mass Spectrometry
Targeted mass spectrometry offers a focused approach to analyzing specific proteins and their PTMs. This technique typically involves pre-selecting peptides of interest and monitoring them precisely during the mass spectrometric analysis. Platforms such as Selected Reaction Monitoring (SRM) and Parallel Reaction Monitoring (PRM) are commonly utilized in targeted mass spectrometry.
The advantage of this approach is its increased sensitivity and quantification ability for low-abundance PTMs. Targeted mass spectrometry reduces background noise and enhances detection limits, making it particularly useful in clinical applications where specific PTMs may indicate diseases. Despite the high specificity, the need for prior knowledge about the proteins of interest can limit the scope of discovery in untargeted settings.
Top-Down and Bottom-Up Approaches
Both top-down and bottom-up approaches provide distinct paths to analyzing proteins and their PTMs. The top-down approach focuses on analyzing intact proteins, maintaining their native form, which is advantageous for studying modifications that may affect the protein’s structure or function. This offers a holistic view but can be technically challenging, as the intact proteins need to be ionized and analyzed in a single run.
Conversely, the bottom-up approach dissects proteins into smaller peptides, making it easier to analyze them with current mass spectrometry technology. This method provides a detailed view of PTMs across many proteins but may miss modifications that only occur in the context of the full protein structure.
Both strategies have their pros and cons. Choosing between them typically depends on the specific goals of the analysis, the complexity of the sample, and the desired depth of information about the PTMs present.
The selection of appropriate mass spectrometry techniques significantly influences the analysis and interpretation of post-translational modifications in biological research.
In summary, mass spectrometry techniques form the backbone of PTM analysis. Each technique presents unique strengths and challenges. The choice of method has profound implications on the experimental outcomes and the insights gained into the biological roles of PTMs.
Sample Preparation for Mass Spectrometry
Sample preparation is a critical step in the mass spectrometry analytical process. It directly influences the quality and accuracy of the results. The objective is to effectively extract proteins, digest them, and enrich for specific post-translational modifications (PTMs) prior to analysis. Inadequate sample preparation can lead to loss of information or faulty data, which may compromise the entire study.
Protein Extraction Techniques
The first stage in sample preparation involves extracting proteins from biological samples. The choice of extraction method is crucial. Various techniques exist, including precipitation, salting out, and buffer extraction. Precipitation techniques often involve solvents like acetone or ethanol. These solvents can remove lipids and other contaminants, facilitating clearer analysis. However, they may also precipitate some PTMs, potentially skewing results. Thus, selecting an appropriate extraction method is essential for preserving the integrity of PTMs.
Moreover, the choice of buffer can significantly influence extraction efficiency. Buffers need to be compatible with subsequent mass spectrometry procedures. If not, they can interfere with ionization and detection. A common buffer used is phosphate-buffered saline (PBS), known for maintaining protein stability. Factors like pH, temperature, and duration of extraction also must be optimized to ensure maximum yield.
Digestion Protocols
Once proteins are extracted, digestion is necessary to break them down into peptide fragments. This step is key for mass spectrometry, which analyzes peptides rather than whole proteins. Trypsin is the enzyme frequently used in these protocols. It cleaves at specific amino acid residues, resulting in a predictable pattern of peptides. This predictability facilitates easier identification during analysis.
There are different approaches for digestion, including in-solution digestion and in-gel digestion. In-solution is typically faster but may introduce variability. Conversely, in-gel digestion tends to offer more homogeneity, but is more time-consuming. The choice between these approaches often depends on the sample context and research goals.
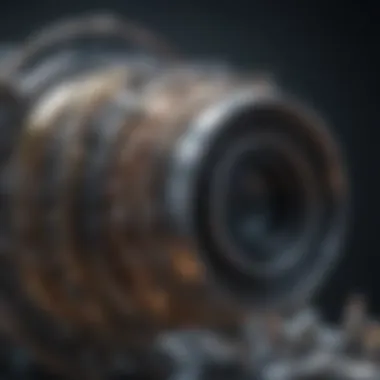
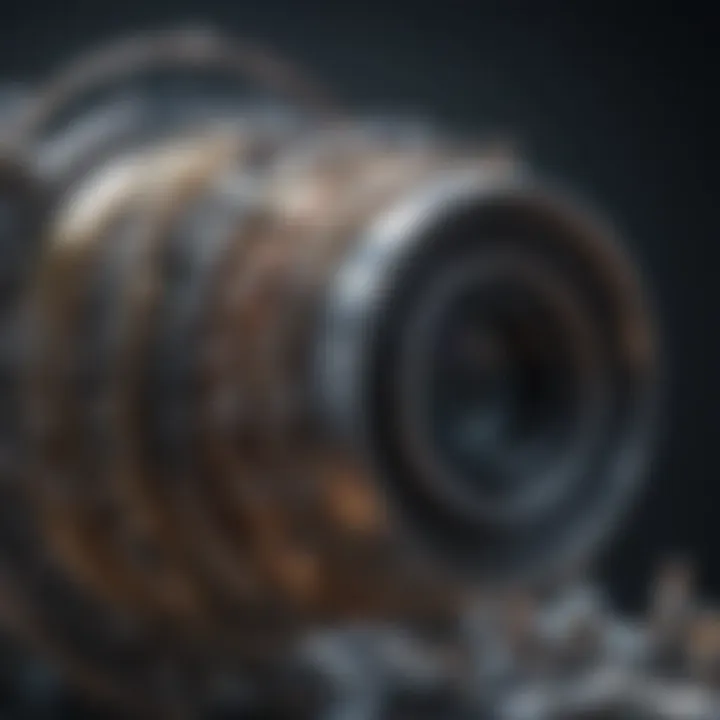
Maintaining proper conditions during digestion is also important. Optimal temperature and incubation time can significantly affect peptide yield and quality. Proper digestion ensures better resolution in mass spectrometry, leading to more reliable identification of PTMs.
Enrichment Methods for PTMs
Enrichment is a strategic step focusing on isolating specific PTMs from the peptide library generated during digestion. Techniques such as affinity enrichment and chromatographic methods (e.g., HILIC, strong cation exchange) are commonly implemented. These techniques exploit the unique properties of modified peptides. For example, immobile phase resin can selectively bind to phosphorylated peptides, allowing others to wash away. This targeted approach enhances the chances of detecting lower abundance modifications, which often go unnoticed.
In addition, careful optimization of enrichment conditions can yield improved results. Factors like temperature, eccentricity, or time should be tailored to each specific type of modification. Researchers need to remain aware of the limitations posed by enrichment methods. For instance, certain procedures could inadvertently eliminate some PTMs or introduce bias by favoring more abundant ones.
Effective sample preparation is not merely a procedural formality; it is an indispensable element that dictates the reliability of mass spectrometric data.
Data Analysis in Mass Spectrometry
The analysis of data in mass spectrometry is crucial for the interpretation of the complex molecular information generated during experiments. It transforms raw spectral data into meaningful biological insights. This section will focus on various key elements including the techniques of data interpretation, software tools, and the overall benefits and considerations involved in the analytical process.
Understanding the mass spectrum is essential. Mass spectrometry generates a graph plotting the intensity of detected ions against their mass-to-charge ratios. Each peak corresponds to a different ion, reflecting its abundance in the sample. Proper interpretation of this data is necessary to identify the presence of post-translational modifications (PTMs). These modifications play significant roles in cellular processes and can influence protein functions. Analyzing spectral data not only helps in recognizing the types of PTMs present but also aids in quantifying their levels in different biological conditions.
The approach to data analysis must consider the complexity of biological samples. Samples often contain a mixture of proteins, each with multiple possible modifications. Thus, robust analysis techniques and tools are needed to filter noise and correctly identify features of interest across varied conditions. It is a challenging but vital aspect of mass spectrometry that strives to bridge the gap between raw data and biological significance.
Mass Spectral Interpretation
Interpreting mass spectral data is a multi-step process that requires both experience and appropriate methodologies. Initially, understanding the mass spectrum is crucial. Factors such as peak identification and comparison to known standards are foundational elements. Researchers must be familiar with various types of PTMs that can occur in proteins and how they influence the mass spectrum. Different PTMs alter the mass of proteins in specific ways, making it imperative to account for these changes when assessing data.
The resolution of mass spectral data significantly affects interpretation. Higher resolution instruments can distinguish closely related ions more effectively, providing clearer insights into specific modifications. Moreover, qualitative analysis, such as identifying the types of PTMs, is complemented by quantitative analysis, which measures the relative abundances of these modifications across samples. Together, these aspects yield insights into the biological context of the proteins being examined.
Software Tools for Data Analysis
Data analysis in mass spectrometry relies heavily on specialized software tools. These tools can streamline the process from raw data acquisition to detailed analysis. Below are some prominent software tools used in this domain.
Proteome Discoverer
Proteome Discoverer is a widely adopted software for the analysis of mass spectrometry data. Its primary contribution lies in its ability to integrate various workflows for analyzing complex samples. It simplifies the identification of proteins and PTMs, making it suitable for researchers in this field.
A key characteristic of Proteome Discoverer is its user-friendly interface. This aspect encourages even less experienced users to conduct their analyses effectively. A unique feature is the ability to perform multi-source analysis, allowing users to integrate results from different experimental setups. However, its reliance on high-quality input data means that it is not suitable for all types of samples, potentially limiting its application in some circumstances.
MaxQuant
MaxQuant is another significant tool designed for quantitative proteomics. Its role in enhancing data analysis capabilities greatly contributes to the field of mass spectrometry. With its ability to manage large datasets efficiently, it helps researchers to identify proteins and their modifications with high accuracy.
MaxQuant’s defining feature is its powerful algorithms that adjust for isotopic labeling, leading to more precise quantification. It is beneficial due to its cost-effective nature, especially in academia. One of its disadvantages, however, lies in its learning curve; new users may find it complex to navigate initially, which can delay research projects.
OpenMS
OpenMS offers an open-source platform for mass spectrometry data analysis. It is particularly appreciated for its flexibility and comprehensive functionalities. Its contributions lie in providing tools for both qualitative and quantitative analysis of mass spectrometric data.
What sets OpenMS apart is its modular design, allowing users to select specific tools based on their needs. This adaptability has made it popular within the scientific community, especially among those who favor open-source solutions. However, a downside could be the necessity of programming skills for advanced usage, which may pose a barrier for some users.
Challenges in PTM Analysis
Analyzing post-translational modifications (PTMs) is a complex and evolving field within proteomics. An understanding of the challenges encountered in this area is fundamental to advancing research and applying findings in practical settings. The intricacies of biological systems can obscure the presence and function of various PTMs. Additionally, the detection of low abundance modifications and issues related to microheterogeneity are common obstacles. Addressing these challenges is not only vital for accurate analysis but also crucial for the interpretation of results in a meaningful biological context.
Complexity of Biological Systems
The biological systems in which proteins operate are inherently complex. Each cell type exhibits unique expression patterns of proteins and PTMs that can vary by diseases, tissues, or environmental conditions. This variability can make it difficult to establish standard protocols for PTM analysis. The multitude of interacting pathways and networks further complicates the identification of specific PTMs and their functional roles.
Moreover, many PTMs occur in response to signals that are transient or variable. For instance, phosphorylation can change rapidly based on external stimuli, making it hard to capture and analyze these modifications at a single time point. This dynamism requires sophisticated experimental designs and technology capable of high-resolution, real-time analyses. Only then can researchers begin to unravel the roles these modifications play in cellular processes.
Low Abundance PTMs
Some PTMs exist in very low abundance, creating significant challenges for detection and quantification. Modifications like methylation or certain ubiquitination types may occur on only a small fraction of total protein molecules. This low prevalence means that they can be easily overlooked in mass spectrometry analyses, especially when using traditional or less sensitive methods.
To combat this issue, researchers often employ enrichment techniques. These include affinity purification strategies utilizing specific antibodies or chemical tagging approaches that allow for the isolation of low-abundance PTMs from a complex background. Such methods help enhance the sensitivity of downstream mass spectrometry analysis. However, the introduction of additional steps in sample processing can also increase the potential for artifacts or variations in results, necessitating careful optimization of each stage.
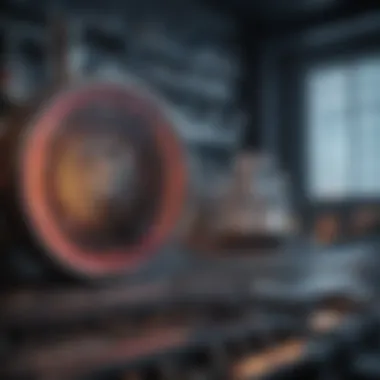
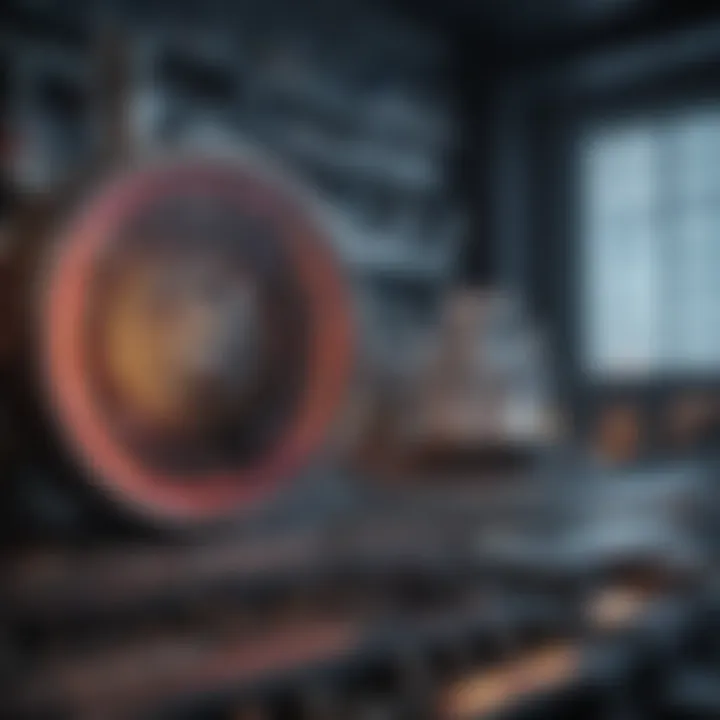
Microheterogeneity
Microheterogeneity refers to the existence of different forms of a protein that arise due to variations in PTMs across molecules. Two identical proteins can end up having different PTM patterns based on factors like cellular context, biological stimuli, or even individual differences among biological samples. This heterogeneity presents a significant challenge when analyzing mass spectrometric data, as it can lead to the generation of a complex spectrum that is hard to interpret.
Researchers need to apply advanced data analysis techniques to differentiate between these forms. High-resolution mass spectrometry is often required to resolve closely spaced peaks that represent microheterogeneous species. Furthermore, integrating various analytical methods such as liquid chromatography or ion mobility spectrometry can be beneficial in distinguishing these variants.
Understanding the challenges in PTM analysis opens pathways to improve methodologies and analytical capabilities, leading to more accurate insights into protein function and regulation.
Applications of PTM Analysis
Post-translational modifications (PTMs) play a critical role in the regulation of protein function within cells. The analysis of PTMs through mass spectrometry has opened new avenues in various fields of research and application. The ability to identify and quantify these modifications can lead to significant advances in understanding biological pathways, disease mechanisms, and therapeutic potentials.
Clinical Applications
In clinical settings, the detection and analysis of PTMs are paramount. They can reveal critical insights into disease states. For example, aberrations in phosphorylation patterns may signal cancer or diabetes. These modifications can affect how a protein behaves, influencing disease progression. Clinicians use PTM analysis to better diagnose, monitor, and personalize treatment strategies. Personalized medicine can benefit greatly as specific PTMs may indicate the best therapeutic approach for an individual patient.
Biomarker Discovery
Biomarkers provide vital information regarding a biological state. PTMs can serve as effective biomarkers. For instance, glycosylation changes are often associated with specific diseases, including various cancers. Identifying these alterations can help researchers and clinicians screen for diseases earlier or more accurately. Mass spectrometry facilitates the discovery and validation of these protein biomarkers, making it an essential tool in clinical diagnostics and research.
Drug Development
The pharmaceutical industry is increasingly recognizing the significance of PTMs in drug development. Drug targets often undergo modifications that alter their function or activity. Understanding these modifications can inform drug design. For example, if a drug is designed to inhibit a specific protein, researchers must consider how PTMs influence the protein’s function. By integrating PTM analysis early in the drug development process, scientists can develop more effective therapeutic agents.
"Understanding PTMs is key to unlocking the complexities of cellular functions and disease processes."
Future Directions in PTM Research
Post-translational modifications (PTMs) have emerged as critical players in the regulation of cellular activities. With ongoing advancements in analytical techniques, especially mass spectrometry, the future of PTM research looks promising. Expanding knowledge in this area allows for a deeper understanding of biological processes and disease states. This section explores the key future directions for PTM research, emphasizing the integration of various methodologies and technological advancements.
Integrating Omics Approaches
The integration of omics approaches is particularly significant in PTM research. The concept of omics refers to the comprehensive study of various biological disciplines like genomics, proteomics, and metabolomics. Each of these domains contributes valuable insights, enabling a more complete view of cellular functions.
When applied to PTM research, integrating omics can reveal how PTMs impact gene expression, protein interactions, and metabolic pathways. By analyzing large datasets obtained from genomics and proteomics, researchers can identify patterns that specific PTMs might influence.
Some benefits of this integration include:
- Holistic Analysis: Researchers can understand how different PTMs interact within the context of overall cellular metabolism.
- Biomarker Discovery: A comprehensive approach may unveil novel biomarkers for diseases based on intricate PTM patterns.
- Personalized Medicine: Understanding PTMs in relation to genomic data can lead to more targeted therapies based on individual patient profiles.
However, researchers must also consider various challenges. Standardization of methodologies and ensuring data interoperability can be complex. Sufficient training in bioinformatics tools is also vital for effective data analysis. Thus, while the potential is large, careful consideration in executing these integrated approaches is crucial.
Advancements in Mass Spectrometry Technology
As technology evolves, mass spectrometry continues to be at the forefront of PTM analysis. Future developments promise to enhance both sensitivity and resolution, allowing for the detailed exploration of PTMs.
Key advancements in mass spectrometry technology could include:
- Increased Sensitivity: New detectors and ionization methods can significantly improve the sensitivity of mass spectrometers, enabling the detection of low-abundance PTMs.
- High-Resolution Mass Spectrometry: Enhanced resolution will facilitate the differentiation between closely related PTMs, which is essential for understanding complex signaling pathways.
- Real-Time Analysis: Future mass spectrometry setups may enable real-time monitoring of PTMs during biochemical reactions, providing insights into dynamic cellular processes.
Moreover, advances such as the utilization of artificial intelligence could revolutionize how data is processed. AI can automate data interpretation, which may ultimately reduce the time needed for analysis and increase the throughput of experiments.
Epilogue
Understanding post-translational modifications (PTMs) is paramount for comprehending the complex mechanisms that operate within biological systems. This article provides a comprehensive overview of the crucial role PTMs play in regulating cellular functions, which is a subject of great importance for researchers and educators alike. The exploration of PTMs through mass spectrometry not only sheds light on their intricate nature but also highlights the methodologies developed for their identification and quantification.
Summary of Key Points
The article discusses several key aspects concerning PTM analysis through mass spectrometry:
- Importance of PTMs: They influence various biological processes, including signal transduction and protein function.
- Mass Spectrometry Techniques: Various techniques, such as shotgun proteomics and targeted mass spectrometr, are essential for detailed PTM study.
- Challenges faced in PTM research: Factors like the complexity of biological systems and low abundance of certain PTMs complicate the analysis.
- Future Directions: Integrating omics approaches can enhance our understanding, and advancements in technology may overcome current limitations.
Implications for Future Research
The insights gained from this exploration invite further investigation in several areas:
- Omics Integration: Combining proteomics with genomics and metabolomics could provide a more holistic view of cellular mechanisms.
- Technological Advances: Continuous improvements in mass spectrometry technologies promise higher sensitivity and specificity. This will lead to better detection of rare and low-abundance PTMs.
- Clinical Relevance: Understanding PTMs can significantly impact biomarker discovery and therapeutic strategies in personalized medicine.
- Innovative Approaches in PTM Detection: Development of novel methodologies along with traditional techniques could pave the way for new discoveries in the field.
In essence, the avenue of mass spectrometry in PTM analysis holds much promise. The ongoing evolution in this field not only enhances our understanding of protein functionality but also opens numerous paths for future exploration.