Understanding the Mechanism of Action of Protease Inhibitors
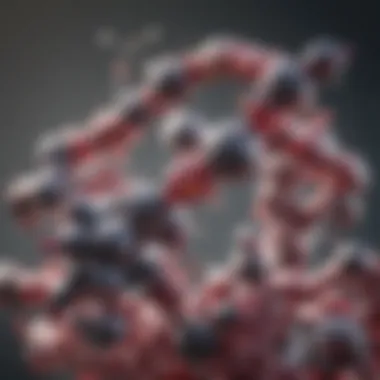
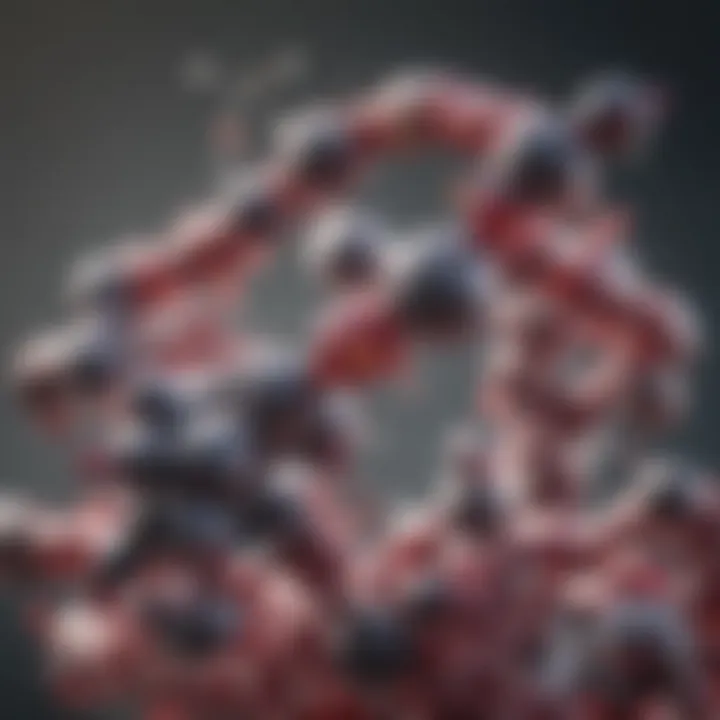
Intro
Protease inhibitors have emerged as essential components in contemporary medicine, particularly in the landscape of viral infections and cancer treatment. The increasing prevalence of conditions such as HIV and various forms of cancer necessitates a deeper understanding of these compounds. This section aims to elucidate the underlying mechanisms of protease inhibitors, shedding light on their biochemical interactions and the vital roles they play in therapeutic contexts.
In basic terms, protease inhibitors work by blocking the activity of proteases, enzymes critical for protein degradation. Their action can prevent viruses from replicating and halt the progression of certain cancer types by interfering with cell cycle regulation. Understanding these mechanisms is not only pivotal for academic research but also for clinical applications. This article will provide a detailed examination of how these inhibitors function and the implications of their mechanisms in fighting diseases.
Methodology
Overview of research methods used
The study of protease inhibitors' action mechanisms encompasses various research methods. Primarily, biochemical assays are employed to investigate how these compounds interact with specific proteases. High-throughput screening techniques allow researchers to identify potential inhibitors from vast compound libraries, facilitating the discovery of promising candidates.
Data collection techniques
Data collection in this field usually involves experimental setups, including:
- In vitro studies: These studies analyze the effects of protease inhibitors on cultured cells and isolated protein systems.
- In vivo experiments: Animal models are often used to assess the therapeutic efficacy of protease inhibitors in a living organism.
- Clinical trials: These are critical for determining the safety and effectiveness of new protease inhibitors in human patients.
Additionally, molecular docking simulations are employed to predict how inhibitors bind to protease active sites, providing insights into their potential effectiveness.
Mechanisms of Action
Protease inhibitors function primarily through competitive inhibition, non-competitive inhibition, or irreversible inhibition.
- Competitive Inhibition: This occurs when the inhibitor binds to the active site of the protease, preventing substrate access.
- Non-competitive Inhibition: In this case, the inhibitor binds to an allosteric site, altering the enzyme's shape and decreasing its activity, regardless of substrate concentration.
- Irreversible Inhibition: These inhibitors form covalent bonds with the enzyme, permanently inactivating it.
Understanding these mechanisms allows researchers to design more effective protease inhibitors tailored to specific diseases.
Future Directions
Upcoming trends in research
Future research in protease inhibitors is likely to focus on:
- Targeting new proteases: Researchers are exploring inhibitors that target proteases involved in various diseases
- Combination therapies: Studies will likely examine the efficacy of combining protease inhibitors with other therapeutic agents.
- Personalized medicine: Tailoring protease inhibitors to individual patient profiles is an emerging area of interest.
Areas requiring further investigation
While there have been significant advances, several areas require deeper exploration:
- Resistance mechanisms: Understanding how viruses or tumors develop resistance to protease inhibitors is crucial for improving therapy.
- Long-term effects: More research into the long-term implications of protease inhibitor therapies is necessary to ensure effective patient outcomes.
In summary, protease inhibitors are invaluable in the treatment of certain diseases. Ongoing research will continue to unearth the complexities related to their mechanisms, further enhancing their therapeutic potential.
"The role of protease inhibitors in modern medicine cannot be understated as they facilitate breakthroughs in addressing some of the most challenging health issues."
By delving into the mechanisms of action, methodologies, and future directions, this article aspires to offer a comprehensive guide for students, researchers, educators, and professionals engaged in this field.
Prolusion to Protease Inhibitors
Protease inhibitors hold significant relevance in modern medicine, particularly in the control and treatment of viral infections and certain cancers. Their role is pivotal in the study of biochemistry and pharmacology. Understanding the mechanisms by which these inhibitors operate provides essential insights into how they regulate processes that are crucial for maintaining cellular health. This section offers a foundational framework for comprehending protease inhibitors, setting the stage for a detailed exploration of their action mechanisms, interactions, and therapeutic applications.
Definition and Importance
Protease inhibitors are molecules designed to block the activity of proteases, enzymes that degrade proteins by cleaving peptide bonds. Their importance in pharmacology cannot be overstated. These compounds selectively inhibit proteases involved in various biological functions, which influences cellular processes such as viral replication and tumor growth.
In viral infections, for example, protease inhibitors prevent the maturation of viral proteins, thus impairing the ability of viruses like HIV and Hepatitis C to proliferate. In cancer therapy, they disrupt the proteolytic pathways that cancer cells exploit to survive and metastasize. Therefore, the therapeutic importance of these inhibitors extends far beyond mere enzyme inhibition; they form the cornerstone of targeted treatment strategies that can significantly improve patient outcomes.
Historical Context
The discovery and development of protease inhibitors date back several decades. Early research in the 1980s highlighted the potential of these compounds in treating HIV/AIDS. The first HIV protease inhibitor, Saquinavir, was approved by the FDA in 1995, marking a crucial advancement in antiretroviral therapy. This approval opened the door to a new class of antiviral drugs, providing a significant improvement in managing HIV infection and transforming the treatment landscape.
Following this success, the exploration of protease inhibitors expanded into oncology. Advances in cancer research revealed that certain proteases facilitated tumor progression and metastasis. Consequently, this led to the development of protease inhibitors targeting tumor-specific proteases, thereby further broadening the therapeutic applications of these compounds.
The evolution of protease inhibitors exemplifies a significant intersection between biochemistry and clinical application, illustrating how scientific research can yield effective therapeutic agents. The historical context not only highlights the progress made in this field but underscores the continuing need for innovative solutions in combating complex diseases.
Types of Protease Inhibitors
Protease inhibitors serve as essential tools in treating various diseases, particularly those linked with infections and certain types of cancer. Understanding the different classes of protease inhibitors is fundamental for appreciating their mechanisms and impacts on therapeutic strategies. This section explores two primary categories: HIV protease inhibitors and protease inhibitors used in cancer treatment. Both types illustrate unique mechanisms of action and highlight considerations crucial for their effectiveness and optimal use.
HIV Protease Inhibitors
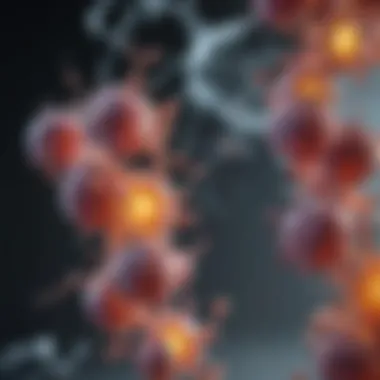
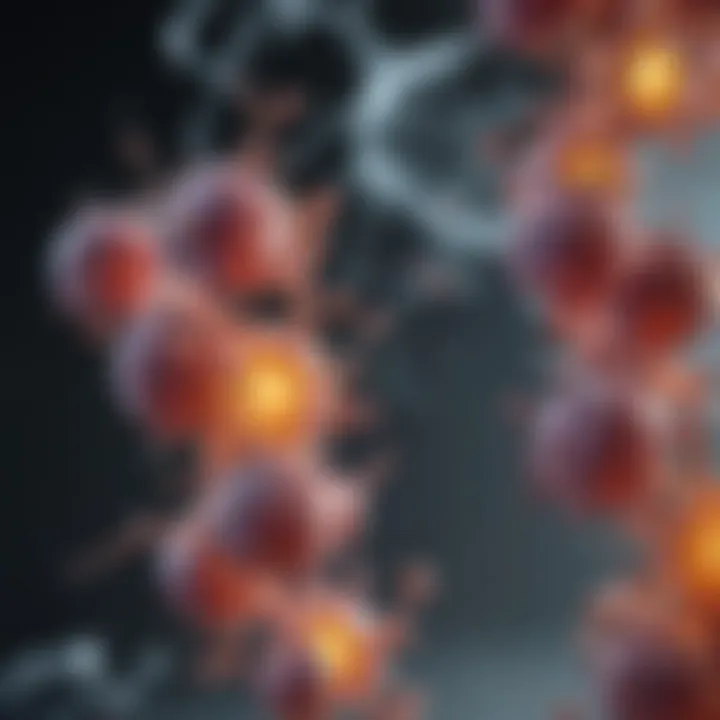
HIV protease inhibitors are a vital class of antiretroviral drugs that help manage HIV infection. They function by blocking the protease enzymes that the virus uses to replicate. Without these enzymes, HIV cannot process its proteins properly, stifling its ability to multiply and spread within the host. Common examples include Ritonavir, Saquinavir, and Lopinavir. Each of these drugs targets the viral protease distinctively, showcasing the critical role of specificity in drug design.
The significance of HIV protease inhibitors extends beyond merely enhancing patient outcomes. Their introduction marked a pivotal moment in HIV treatment, shifting the paradigm towards combination therapies. This approach helps in overcoming resistance, which can arise due to mutations in the virus. Moreover, HIV protease inhibitors have contributed to reducing HIV-related morbidity and mortality rates significantly. Evaluating their effectiveness requires careful attention to dosing regimens, possible drug-drug interactions, and patient adherence, all of which play fundamental roles in the success of the antiretroviral therapy.
Protease Inhibitors in Cancer Treatment
Protease inhibitors also have a prominent role in oncology. Inhibitors such as Bortezomib are utilized for treating multiple myeloma and certain other malignancies. The action of protease inhibitors in cancer treatment focuses primarily on the inhibition of the proteasome, an essential cellular structure responsible for degrading ubiquitinated proteins. By preventing this degradation, these inhibitors lead to an accumulation of regulatory proteins that induce apoptosis or cell cycle arrest in cancer cells.
Cancer cells often exploit the proteasome system for their survival and proliferation. Therefore, the use of protease inhibitors can disrupt these processes, giving clinicians a potent means of combating tumor growth. However, the development of resistance remains a critical challenge in this area. Variations in the proteasome's structure or expression in cancer cells can diminish the efficacy of treatment over time.
Biochemical Mechanisms
Understanding the biochemical mechanisms behind protease inhibitors is essential for harnessing their therapeutic potential. This segment elucidates how these inhibitors interact at the molecular level, significantly affecting proteolytic activities in various biological contexts. By revealing the intricate details of these processes, we can appreciate how protease inhibitors contribute to disease management, particularly in viral infections and cancer. Their role in modulating enzymatic reactions is of paramount importance, as it directly correlates with their efficacy as therapeutic agents.
Enzymatic Processes
Proteases are enzymes that catalyze the breakdown of proteins by cleaving peptide bonds. The action of protease inhibitors focuses on disrupting this enzymatic activity. These inhibitors often mimic substrate structures, allowing them to fit into the active site of the protease. This interaction prevents the target protease from performing its natural function.
- Mechanism of Binding: The binding of a protease inhibitor can be either reversible or irreversible. In reversible inhibition, the inhibitor can detach from the active site, whereas irreversible inhibitors form a permanent bond with the enzyme.
- Examples of Protease Inhibitors: Drugs like Ritonavir and Saquinavir are designed to inhibit HIV proteases effectively. Understanding the binding affinity and specificity can guide drug development and therapy adjustments.
The significance of these enzymatic processes cannot be overstated. They dictate the potency and selectivity of a drug, and further insights into these interactions can lead to refined therapeutic strategies.
Inhibition Kinetics
The kinetics of inhibition describes the rate at which a protease inhibitor acts to decrease the activity of its target enzyme. This concept is crucial for understanding how quickly and effectively these inhibitors can alter biological processes. Different inhibition kinetics models can explain various outcomes and provide insight into drug interactions.
- Michaelis-Menten Kinetics: Many enzymes, including proteases, follow the Michaelis-Menten model. Here, the rate of reaction is dependent on substrate and inhibitor concentrations. Knowing these rates helps in predicting how changes in drug dosage can influence treatment outcomes.
- Factors Influencing Kinetics: Several variables affect inhibition kinetics, including pH, temperature, and enzyme concentration. Researchers must consider these factors when designing experiments or clinical trials.
Inhibition kinetics studies not only enhance our understanding of the inhibitors themselves but also offer valuable information for optimizing treatment protocols. The speed and efficiency of inhibition play a crucial role in therapeutic efficacy, particularly in acute diseases like HIV or rapidly proliferating cancers.
Understanding these biochemical mechanisms is vital for the continued development of effective protease inhibitors, which can dramatically improve patient outcomes in various diseases.
Interactions with Target Proteases
Understanding the interactions between protease inhibitors and their target proteases is essential for comprehending their mechanisms of action. These interactions are not just biochemical occurrences; they are fundamental to the efficacy of these inhibitors in therapeutic applications. Protease inhibitors are designed to mimic the substrate of proteases, allowing them to bind effectively and block the protease’s activity. This inhibition is crucial for controlling various diseases, particularly viral infections and certain cancers.
Binding Affinity
Binding affinity refers to the strength of the interaction between a protease inhibitor and its target protease. A high binding affinity suggests that the inhibitor will effectively block the protease’s function. The extent of this affinity is determined by several factors, including the size, shape, and charge of the inhibitor. These physical properties allow the inhibitor to fit snugly into the protease's active site, preventing substrate access.
- Importance of Binding Affinity
High binding affinity contributes significantly to therapeutic outcomes. In the context of HIV treatment, for instance, a drug like Lopinavir is highly effective due to its strong binding affinity to the HIV protease, leading to decreased viral load in patients. This characteristic ensures that smaller doses can be used, which potentially minimizes side effects. - Considerations
It is worth noting that an excessively high binding affinity can lead to prolonged side effects, as the inhibitor may remain bound to the protease longer than desired. Therefore, optimizing binding affinity is crucial in drug design.
Active Site Interactions
The active site of a protease is the region where substrate molecules bind and undergo chemical reactions. Protease inhibitors target these active sites to prevent substrate binding, thereby inactivating the protease. This process involves several interactions: hydrogen bonds, hydrophobic interactions, and van der Waals forces.
- Types of Interactions
- Hydrogen Bonds: These are weak interactions but can significantly stabilize the binding of an inhibitor to a protease. Understanding how to manipulate these bonds is vital in the design of effective inhibitors.
- Hydrophobic Interactions: Inhibitors often contain hydrophobic regions that interact favorably with hydrophobic areas in the active site. This interaction can enhance binding strength and specificity.
- Van der Waals Forces: These forces arise from transient shifts in electron density and help the inhibitor to adhere closely to the protease.
In summary, the interactions at the active site define the specificity and strength of protease inhibitors. The design of new inhibitors often involves detailed studies of these interactions, aimed at optimizing the therapeutic potential while minimizing adverse effects.
"The intricate balance of binding affinity and active site interactions forms the cornerstone of effective protease inhibitor design, influencing both efficacy and safety in treatment regimens."
Supporting advancements in this area leads to better treatment protocols and improved patient outcomes.
Therapeutic Applications
Protease inhibitors have emerged as vital tools in modern medicine, particularly in the field of antiviral and anticancer therapies. Their ability to target and inhibit specific proteases has profound implications for treatment protocols, leading to improved patient outcomes and innovative therapies. This section delves into the specific therapeutic applications of protease inhibitors, focusing on their role in HIV treatment protocols and anticancer strategies, as well as the considerations that accompany their use.
HIV Treatment Protocols
HIV protease inhibitors, such as Ritonavir, Saquinavir, and Lopinavir, play a crucial role in the management of HIV infection. These drugs block the action of the protease enzyme, which is essential for the maturation of infectious virions. By inhibiting this enzyme, protease inhibitors prevent the cleavage of viral polypeptides, thereby producing non-infectious virus particles.
The incorporation of protease inhibitors into combination antiretroviral therapy (cART) has led to significant reductions in viral load and improved immune function. The synergy observed when these drugs are used in conjunction with reverse transcriptase inhibitors enhances their overall efficacy. However, it is important to monitor patients for potential side effects and drug interactions. Many protease inhibitors are metabolized by the liver, which necessitates careful management of additional medications.
Furthermore, adherence to treatment regimens is paramount. Studies show that missed doses can lead to resistance, limiting the utility of these valuable drugs. The development of fixed-dose combinations has helped improve compliance and facilitate easier treatment plans for patients.
Anticancer Strategies
In oncology, protease inhibitors are gaining traction as potential therapeutic agents. Cancer cells often exploit proteolytic processes for progression and metastasis. By disrupting these pathways, protease inhibitors can induce apoptosis in tumor cells and inhibit processes like angiogenesis.
For instance, the use of bortezomib in multiple myeloma demonstrates how proteasome inhibition can lead to accumulation of pro-apoptotic factors, effectively reducing tumor burden. Similarly, drugs like Marizomib are being explored in clinical trials, targeting specific proteases involved in tumor growth and metastasis.
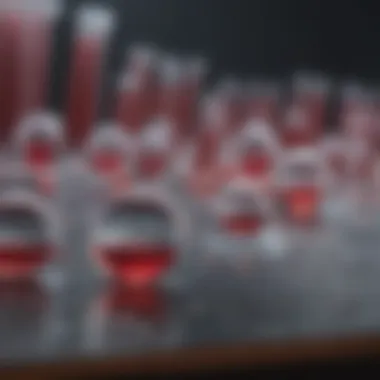
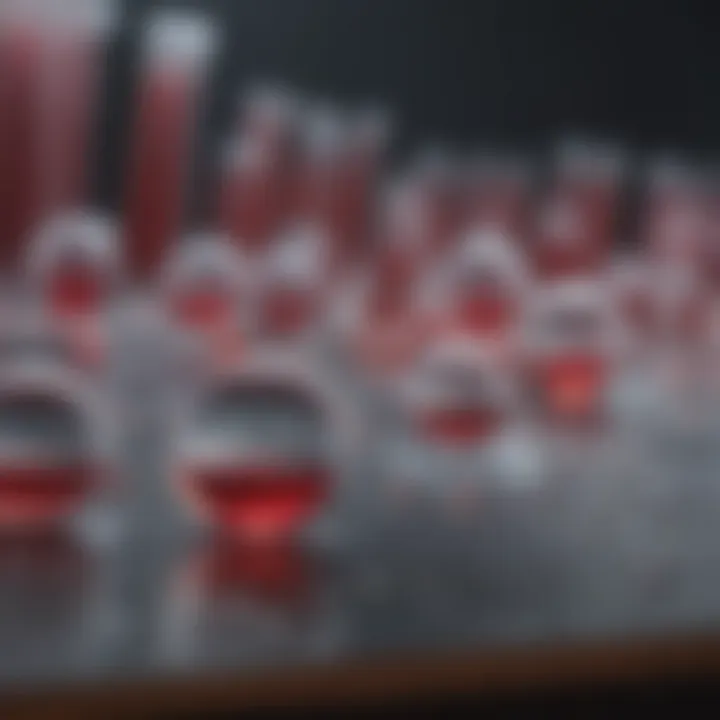
The adaptability of protease inhibitors extends to various cancers, including breast cancer and pancreatic cancer, where specific protease expressions correlate with disease outcomes. However, the application of these inhibitors is not without challenge. The tumor microenvironment can adapt to the therapeutic pressure, leading to resistance. Continuous research is essential to identify biomarkers that predict response to treatment and to develop next-generation protease inhibitors that surpass the limitations of current therapies.
"Protease inhibitors are not just tools; they alter the landscape of therapeutic strategies in infectious diseases and cancer."
The evolving understanding of protease inhibitors informs ongoing research, guiding the design of future drug candidates and optimizing treatment regimens. As our comprehension of their mechanisms deepens, so too does their potential to transform treatment paradigms across disciplines.
Advances in Research
Research into protease inhibitors is evolving rapidly. As wider applications in medicine are discovered, understanding these advances is vital. The continuous exploration of protease inhibitors offers hope for better treatment strategies against viral infections and cancers. This section examines recent breakthroughs and provides insights into how these developments enhance therapeutic efficacy.
Clinical Trials and Findings
Clinical trials are the backbone of testing the effectiveness and safety of protease inhibitors. Recent studies have illuminated their role in various therapeutic contexts. For instance, trials involving Darunavir and Atazanavir demonstrate their effectiveness in treating HIV. Evidence shows that these inhibitors can reduce viral load significantly and improve patient outcomes.
New combinational approaches are also being assessed. By pairing protease inhibitors with other classes of antiretroviral drugs, researchers seek to enhance treatment regimens, potentially leading to shorter treatment durations and increased adherence.
Moreover, the impact of protease inhibitors in oncological settings is gaining attention. Clinical findings suggest that certain inhibitors like Lopinavir can inhibit proteases that are crucial for tumor growth. This biological mechanism opens avenues for targeted therapies that could enhance patient survival rates.
"Ongoing trials provide essential insights that shape the future of protease inhibitor therapy."
Emerging Inhibitors
The landscape of protease inhibitors is continually changing. Emerging inhibitors show promise in their ability to target various proteases with high specificity. For instance, newer compounds like Nirmatrelvir, an antiviral drug developed for COVID-19, exemplify the shift towards addressing viral infections with innovative strategies.
These new inhibitors often employ different mechanisms. Some might act as allosteric modulators, altering the active site's conformation to prevent substrate binding. This represents a significant advancement in the field, as it may lead to the development of drugs that can overcome existing resistance mechanisms.
Additionally, research into plant-based inhibitors is underway. Compounds derived from natural sources can provide alternative mechanisms of inhibition that may prove successful against resistant strains of viruses and cancers.
The exploration of these emerging inhibitors is crucial not only for improving drug efficacy but also for addressing the challenges posed by resistance in treatment protocols.
In summary, advances in the research of protease inhibitors underline a commitment to enhancing therapeutic interventions. The focus on clinical trials and the emergence of innovative compounds are reshaping the future landscape of medicine.
Effects on Cellular Processes
The effect of protease inhibitors on cellular processes is a central theme in understanding their therapeutic mechanisms. Protease inhibitors play a significant role in regulating proteolytic activities, which are essential for maintaining cellular homeostasis. When these inhibitors interact with target proteases, they effectively modulate various biochemical pathways that are fundamental to both viral infections and cancer progression.
Role in Protein Regulation
Proteins undergo a series of regulatory processes that are crucial for their function. Protease inhibitors limit the action of proteases, enzymes that break down proteins. This inhibition can lead to the stabilization of certain proteins that might be otherwise degraded. In viral infections, for instance, viral proteins often require proteolysis for maturation. By blocking this process, protease inhibitors prevent the virus from replicating effectively. The stabilization of host proteins can also have profound effects, often enhancing antiproliferative signals that limit tumor growth.
Protease inhibitors do not only affect the target proteases directly but also indirectly impact many other cellular functions.
- Cell Cycle Regulation: Protease inhibitors can influence cyclins and cyclin-dependent kinases that are crucial for cell cycle control.
- Apoptotic Pathways: The inhibition of specific proteases can protect against apoptosis, allowing cells to survive longer, which can be both beneficial and detrimental in different contexts.
Impacts on Cell Signaling
Cell signaling is a complex process that allows cells to communicate with each other. Protease inhibitors have notable effects on signaling pathways by altering the proteolytic events that can activate or deactivate signaling molecules. For example, certain signaling pathways rely on proteolytic cleavage as a mechanism of activation. When protease inhibitors block this cleavage, the signaling pathways can be disrupted.
In cancer, altered signaling can lead to aberrant cell growth and survival. Protease inhibitors can restore normal signaling by preventing the cleavage of specific substrates, which can affect pathways like the MAPK or PI3K/AKT signaling cascades.
Furthermore, protease inhibitors can influence the activity of cytokines and growth factors that are crucial for cellular communication and immune responses.
"Protease inhibitors not only inhibit viral replication but also reshape cellular signaling networks, making them versatile agents in disease management."
As the research community continues to explore the intricate relationships between proteases and cellular processes, the implications for future therapies become more evident. Understanding these dynamics provides insights into how to enhance the efficacy of protease inhibitors, tailoring them to specific therapeutic needs.
Resistance Mechanisms
Understanding resistance mechanisms is critical in the study of protease inhibitors. Resistance can significantly affect the effectiveness of these drugs, impacting treatment outcomes in both viral infections and certain cancers. The presence of resistance mechanisms complicates therapy regimens and may necessitate adjustments in drug selection or combinations. Thus, it becomes essential to explore these mechanisms in depth, which can provide insights for improving therapeutic strategies.
Viral Resistance
Viral resistance to protease inhibitors often arises during chronic infections. For instance, in HIV, mutations in the protease enzyme can confer resistance, rendering the inhibitors less effective. These mutations typically occur when the virus replicates in the presence of a protease inhibitor, making the virus adapt by altering its protease structure. Consequently, there are specific residues in the HIV protease that, when changed, hinder the drug's binding capabilities.
Key considerations include:
- Mutational Pathways: Various pathways can lead to resistance. For example, the substitution of amino acids can change the shape of the active site, decreasing inhibitor binding.
- Cross-Resistance: Certain inhibitors may have overlapping activity profiles. This means resistance to one inhibitor can lead to resistance against others in the same class, complicating treatment choices.
- Combination Therapy: Using multiple drugs can sometimes circumvent resistance. This approach aims to reduce the viral load beyond a threshold that permits effective treatment.
"Continued surveillance of viral mutations is essential for the effective management of HIV treatment protocols."
Tumor Microenvironment Challenges
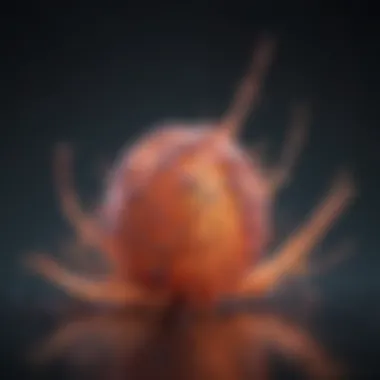
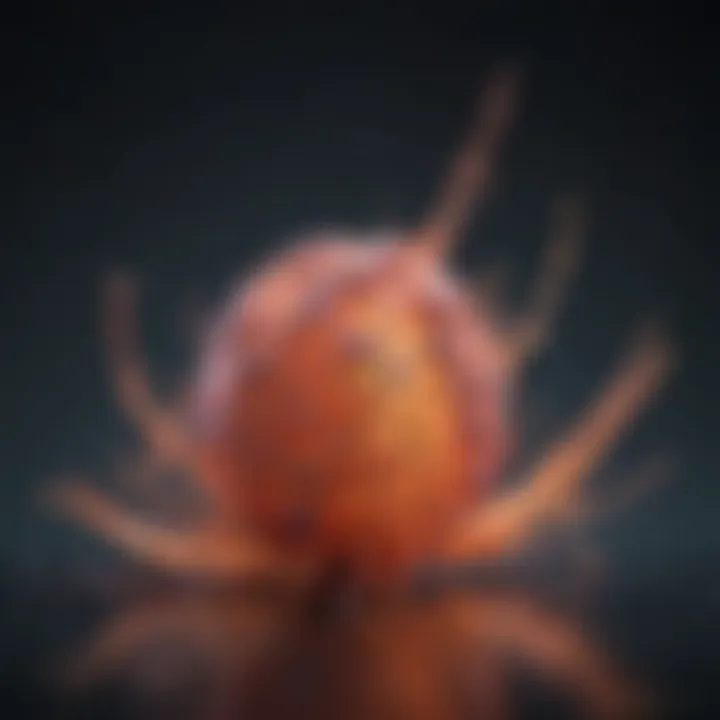
In cancer treatment, the tumor microenvironment poses significant challenges to the efficacy of protease inhibitors. Tumor cells can develop resistance mechanisms that allow them to evade drug action. Factors such as hypoxia, altered pH, and the presence of extracellular matrix components in tumors can affect drug delivery and action.
Important aspects of the tumor microenvironment include:
- Cellular Heterogeneity: Tumors are often composed of diverse cell populations, some of which may exhibit resistance to protease inhibitors.
- Drug Transport: The physical properties of tumors can affect how drugs penetrate them. Inadequate penetration can lead to subtherapeutic drug levels, promoting resistance.
- Adaptive Responses: Tumor cells might adapt to protease inhibition by upregulating alternative pathways, compensating for the inhibited proteolytic processes.
These factors highlight the need for ongoing research to understand resistance mechanisms fully and develop strategies to improve the effectiveness of protease inhibitors in treating cancer.
Regulatory and Ethical Considerations
Understanding the regulatory and ethical considerations surrounding protease inhibitors is essential for both the development of new therapies and ensuring patient safety. The rigorous approval processes and ethical frameworks guide researchers and organizations in their quest to discover effective treatments while prioritizing public health and moral responsibilities.
Approval Processes for New Inhibitors
The approval process for new protease inhibitors involves several stages, ensuring that each drug is evaluated thoroughly before it reaches patients. Typically, this process begins with laboratory research, followed by preclinical trials that assess the drug’s safety and efficacy. Once promising results are obtained, the drug moves on to clinical trials, which are usually conducted in three phases:
- Phase I: Focuses primarily on safety. A small group of healthy volunteers receives the drug in varying doses to determine its safety profile and identify any potential side effects.
- Phase II: Involves a larger group of patients who have the disease or condition the drug is intended to treat. This phase aims to evaluate the drug’s effectiveness and further assess safety.
- Phase III: Conducted on an even larger population, this phase compares the new drug against current standard therapies. It aims to provide information about the drug's overall benefit-risk profile.
Once these phases are completed satisfactorily, regulatory bodies like the U.S. Food and Drug Administration or the European Medicines Agency review all gathered data. Their objective is to ensure that the drug is both effective and safe for public use, before granting approval for market release. Such meticulous processes are crucial, as they safeguard patients from harmful side effects and unproven treatments.
Ethical Implications in Research
Ethical considerations in the research of protease inhibitors encompass various aspects, ranging from informed consent to equitable access to treatments. Researchers must ensure that participants involved in clinical trials understand the study’s purpose, potential risks, and their right to withdraw at any time without penalty. This principle of informed consent is fundamental in maintaining trust between researchers and participants.
Moreover, ethical dilemmas can arise when considering vulnerable populations. Researchers have a responsibility to ensure that these groups are not exploited and that they benefit from the research outcomes without facing disproportionate risks.
Access to new treatments raises additional ethical questions. As protease inhibitors can be expensive and not universally available, there is an ongoing debate about how to ensure equitable distribution. Ethical research must consider long-term impacts not just on individual patients, but also on broader society and healthcare systems.
"The balance between innovation and ethical responsibility in protease inhibitor research is delicate and crucial."
In summary, regulatory and ethical considerations are indispensable in the realm of protease inhibitors. They help to navigate the complexities of drug development and ensure that new therapies serve both individual patient needs and the greater societal good.
Future Perspectives
The future perspectives concerning protease inhibitors are a critical area of examination in the ongoing advancements in medical therapeutics. As researchers delve deeper into understanding the intricate details of protease functions, the development of more potent and selective inhibitors becomes increasingly feasible. This not only enhances the treatment options available but also raises the possibility of tailoring therapies to individual patients.
Innovations in Drug Design
The innovations in drug design of protease inhibitors focus on improving efficacy and minimizing side effects. Recent studies emphasize the role of structure-based drug design, which harnesses computational methods to predict how new compounds will interact with target proteases. This approach allows for the identification of novel inhibitors that bind more effectively to active sites while increasing specificity.
Technologies like high-throughput screening and combinatorial chemistry also play a significant role. They enable rapid identification of the most promising candidates among thousands of molecules. Notably, advancements in nanotechnology are leading to the creation of nanocarrier systems that can deliver inhibitors directly to diseased cells. This targeted delivery enhances the therapeutic profile and reduces systemic exposure, which may alleviate toxicity concerns.
Potential New Applications
The potential new applications of protease inhibitors extend beyond their traditional roles in treating HIV and certain cancers. Recent research indicates their promising role in managing autoimmune diseases and neurodegenerative disorders. By modulating proteolytic pathways, these inhibitors may help regulate immune responses or reduce the aggregation of proteins associated with conditions like Alzheimer's disease.
Furthermore, protease inhibitors are being explored for their antitumor properties. For example, certain inhibitors may disrupt specific proteolytic pathways that cancers exploit for invasion and metastasis. This therapeutic versatility showcases the adaptability of protease inhibitors in addressing varied health challenges.
Recent studies suggest that protease inhibitors could revolutionize treatment protocols for complex diseases, reflecting their potential as multifaceted therapeutic agents.
In summary, as research progresses, the realm of protease inhibitors continues to evolve. Innovations in drug design, along with discoveries of new application areas, present a landscape ripe with possibilities for enhancing patient outcomes and expanding therapeutic approaches.
Closure
Protease inhibitors play a significant role in modern medicine, especially concerning antiviral and anticancer strategies. Their ability to interrupt protein catalytic processes can be leveraged to manage various diseases effectively. Understanding the precise mechanisms of action for these compounds not only illustrates their therapeutic benefits but also highlights the necessary precautions involved in their use.
The synthesis of data in this article highlights several critical elements of protease inhibition:
- Blocking Enzyme Activity: Protease inhibitors bind competitively or non-competitively to specific proteases, reducing their activity. This effectively limits viral replication in cases of HIV or impedes the growth of malignant cells.
- Biochemical Interactions: Understanding how these inhibitors interact at the molecular level assists in drug design, improving efficacy and safety profiles.
- Resistance Mechanisms: Insight into how viruses or cancer cells evade these inhibitors provides a roadmap for future research and development.
Thus, the discussion on protease inhibitors extends beyond mere chemical compounds. It evolves into a narrative of targeted therapy, offering new avenues for treatment while also presenting challenges that require ongoing investigation. The focus on future research directions is crucial as the landscape of medicine is ever-evolving, and maintaining relevance is paramount.
Summary of Mechanistic Insights
Protease inhibitors are designed to interfere with the function of proteases, enzymes that are pivotal in numerous biological processes. Their mechanism is often complex, hinging on specific biological targets. The insights into these mechanisms unfold as follows:
- Active Site Binding: Many protease inhibitors are structurally analogous to the substrates for proteases, allowing them to fit into the active site. This competitive binding is a staple strategy in inhibitor design.
- Allosteric Regulation: Some inhibitors work by binding to sites other than the active site, inducing conformational changes that decrease enzyme activity.
- Kinetic Parameters: The effectiveness of an inhibitor can often be assessed through its kinetic parameters, such as Ki (inhibition constant) and IC50 (the concentration needed to inhibit 50% of enzyme activity). These metrics help researchers compare different inhibitors and assess their potential clinical impact.
Ultimately, understanding these mechanisms clarifies how protease inhibitors can be optimized for specific therapeutic targets.
Final Thoughts on Research Directions
The landscape for protease inhibitors is ripe with possibilities. Continued research is vital to not only address the challenges of drug resistance but also to expand the therapeutic applications of these compounds. Areas for future exploration could include:
- Novel Inhibitor Classes: Identifying and synthesizing new classes of protease inhibitors that exhibit unique properties or target different proteases.
- Combination Therapies: Researching the synergistic effects of protease inhibitors with other therapeutic modalities could enhance treatment efficacy.
- Personalized Medicine: Tailoring protease inhibitor strategies based on individual genetic profiles may optimize therapeutic responses and mitigate adverse effects.
By pursuing these directions, researchers can improve the trajectory of protease inhibitors in both viral and oncological contexts, ensuring they remain a cornerstone of targeted therapies in the coming decades.
"The future of protease inhibitors is not just in understanding their action but in innovating the approaches that will define the next generation of treatments."