The Impact of LC-MS Instrumentation in Modern Research
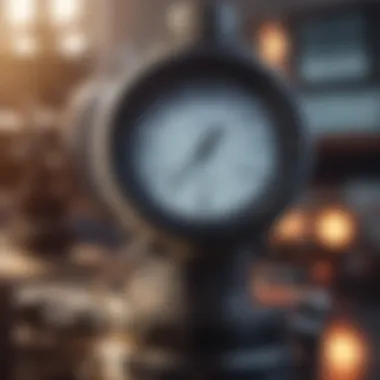
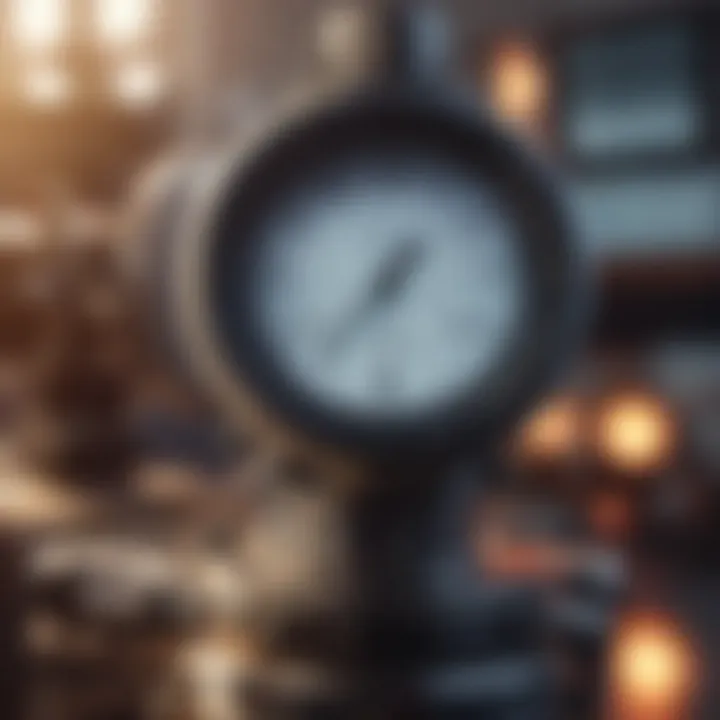
Intro
Liquid Chromatography-Mass Spectrometry (LC-MS) has emerged as a crucial technique in the realm of scientific research. This method combines the physical separation capabilities of liquid chromatography with the mass analysis capabilities of mass spectrometry. By doing so, it allows for detailed characterization of complex mixtures, providing insights that are essential across various fields including chemistry, biology, and environmental science. The relevance of LC-MS in modern research cannot be understated as it enhances our ability to analyze biomolecules, contaminants, and other substances with high precision.
In this article, we will delve into the methodology behind LC-MS, the principles guiding its operation, and the various applications that underscore its significance. We aim to provide a comprehensive overview that not only informs but also enhances the understanding of this intricate technology through detailed exploration of its foundational concepts, advancements, and challenges.
Methodology
Overview of Research Methods Used
The methodological framework of LC-MS encompasses several components that work together to ensure reliable and reproducible results. The process begins with effective sample preparation, which is critical for successful analysis. This may involve filtration, dilution, or concentration steps to minimize matrix effects and enhance analyte detection.
Separately, the liquid chromatography phase involves the distribution of analytes between a stationary phase and mobile phase, leading to their separation based on physical and chemical properties. The choice of column, mobile phase composition, and gradient elution are vital aspects that significantly influence separation efficiency.
Data Collection Techniques
Once the samples are analyzed, mass spectrometry detects the separated compounds. This stage involves ionization of analytes, and there are various ionization techniques such as Electrospray Ionization (ESI) and Matrix-Assisted Laser Desorption/Ionization (MALDI). The ions produced are then sorted and detected based on their mass-to-charge ratios.
Furthermore, data collection relies on software that interprets mass spectral data, generating information about molecular weight, structure, and concentration of analytes. The integration of advanced algorithms and data processing techniques is crucial for accurate interpretation of results. High-resolution mass spectrometry has become necessary for differentiating between compounds with similar mass spectra.
"The integration of liquid chromatography with mass spectrometry allows researchers to identify and quantify complex mixtures with unprecedented accuracy."
Future Directions
Upcoming Trends in Research
Looking ahead, the future of LC-MS is promising, with several trends expected to shape its evolution in scientific research. Continuous advancements in technology are likely to increase resolution, sensitivity, and speed of analysis. Additionally, developments in miniaturization and portability of LC-MS systems may facilitate field-based applications.
Areas Requiring Further Investigation
Despite its advancements, further investigation is needed in a few areas. This includes improving the reliability of results in complex biological samples, which often pose challenges due to high matrix effects. Moreover, the integration of artificial intelligence in data analysis is an area with great potential for enhancing LC-MS capabilities, allowing for smarter interpretations of large data sets. As researchers continue to explore novel applications in various sectors, including drug discovery and environmental monitoring, the depth of understanding regarding LC-MS will likely evolve.
The ongoing exploration and refinement of LC-MS technologies underscore their critical role in unraveling scientific queries across diverse domains. In closing, the narrative surrounding LC-MS is one of continuous growth and innovation, reflecting its significance in contemporary scientific advancements.
Prelims to LC-MS Instrumentation
Understanding LC-MS instrumentation is crucial in modern scientific exploration. Liquid Chromatography-Mass Spectrometry combines two powerful analytical techniques to analyze complex samples. This integration provides unmatched sensitivity, specificity, and versatility in various fields, including pharmacology and environmental science.
The significance of this instrumentation cannot be overstated. With increasing demands for precise analysis in research and industry, LC-MS has become a preferred method. It enables scientists to separate, identify, and quantify compounds in a single run.
Moreover, the ability to analyze different types of samples, from small molecules to large biomolecules, emphasizes its relevance. As such, LC-MS continuously evolves through technological advancements, making it a fundamental tool for contemporary scientists.
Historical Background
The development of LC-MS began in the late 20th century. Earlier forms of mass spectrometry, developed in the 1940s and 1950s, laid the groundwork for this innovation. However, the true breakthrough came with the advancement of high-performance liquid chromatography in the 1980s. Researchers started to see the potential of integrating these two methods. By the early 1990s, the first commercially available LC-MS systems emerged. The combination allowed for more efficient analyses, marking a significant shift in analytical chemistry.
Over the years, LC-MS has seen enhancements that improved its efficacy. The introduction of electrospray ionization in the 1990s further revolutionized the field, making it easier to analyze larger biological molecules. Today, LC-MS is standard in many labs, showcasing its adaptability and reliability in science.
Overview of LC-MS Technology
Liquid Chromatography-Mass Spectrometry works on two core principles: separation through liquid chromatography and identification through mass spectrometry.
- Liquid Chromatography: This technique separates components of a mixture based on their interactions with the stationary phase and mobile phase. Various methods, such as reversed-phase and size-exclusion chromatography, can be employed depending on the sample.
- Mass Spectrometry: Once separated, components are ionized and detected based on their mass-to-charge ratio. This allows for the identification of compounds with high precision. The combination of these techniques leads to a powerful analytical tool that provides insights into the composition of complex mixtures.
In summary, LC-MS technology is a cornerstone of analytical science. Its historical development illustrates its growing importance and ongoing evolution. Understanding these elements enhances the appreciation of its role in research.
Principles of Liquid Chromatography
Liquid chromatography (LC) is a crucial analytical technique, underpinning the effectiveness of Liquid Chromatography-Mass Spectrometry (LC-MS). Understanding the principles of liquid chromatography is essential for optimizing the analytical methods used in contemporary scientific research. This section elucidates the fundamentals of separation mechanisms, chromatography phases, and detector types, all while emphasizing their importance in achieving precise and accurate analytical results.
Separation Mechanisms
Separation mechanisms are the heart of liquid chromatography. The primary purpose of LC is to separate compounds in a mixture based on their chemical properties. For effective separation, two key mechanisms are primarily utilized: partitioning and adsorption. In partitioning, the analytes partition between two liquid phases, typically a stationary phase (liquid) and a mobile phase (also liquid). Conversely, in adsorption mechanisms, analytes adhere to a solid stationary phase and are then eluted by the mobile phase.
Different methods, like reverse phase and normal phase chromatography, leverage these mechanisms uniquely. Reverse-phase chromatography involves a non-polar stationary phase and a polar mobile phase, favoring compounds with lower polarity for retention and separation. This is valuable in pharmaceuticals and biochemistry, where precise separation of complex mixtures is often required.
Meanwhile, the effectiveness of separation can be influenced by factors such as:
- Flow Rate: Higher flow rates reduce separation efficiency but decrease analysis time.
- Column Temperature: Changes can affect viscosity and analyte behavior.
- Sample Composition: Variations in sample polarity can lead to differing retention times.
Understanding these mechanisms is vital for optimizing LC techniques and enhancing the quality of data produced.
Chromatography Phases
Chromatography consists of two phases: stationary and mobile. The stationary phase is the medium where separation occurs, and can have various forms such as silica, polymer-based, or bonded phases. The mobile phase, often a solvent or a mixture of solvents, carries the sample through the stationary phase.
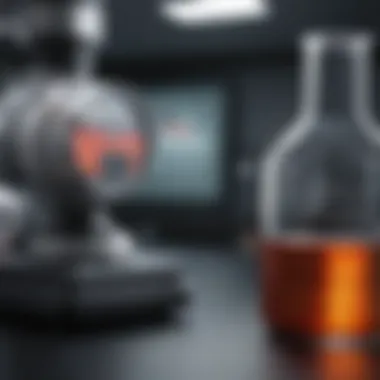
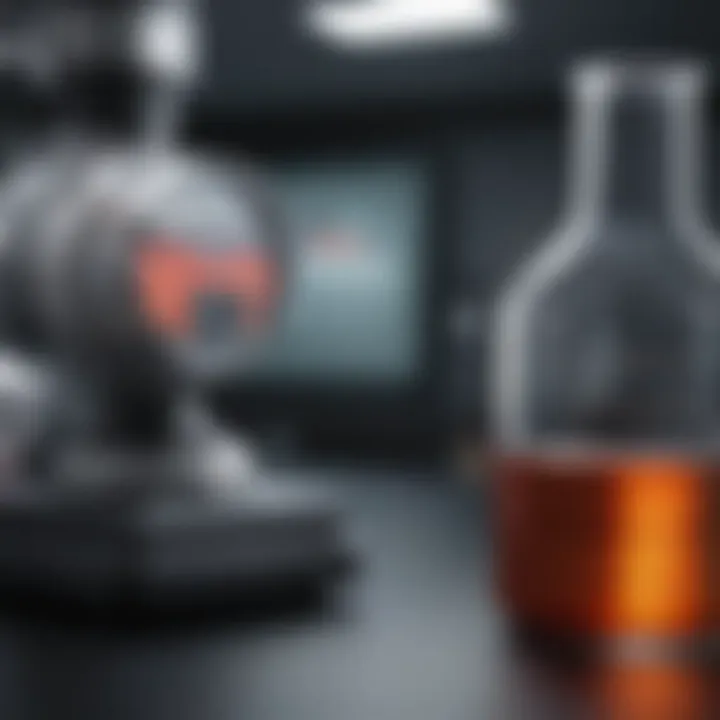
Key considerations include:
- Selection of Mobile Phase: This is critical as it influences the interaction of analytes with the stationary phase. For example, using a gradient elution technique can help achieve better resolution of complex mixtures.
- Reproducibility: The consistency of interactions between the stationary and mobile phases can greatly affect results.
Overall, the choice and interplay of chromatography phases can determine analytical performance and are key factors in method development.
Detector Types in
The detection of separated analytes is a pivotal aspect of liquid chromatography. Various detector types are available, each offering different advantages. These include:
- UV/Visible Detectors: Common in LC due to their simplicity and wide application range. They rely on the absorbance of UV or visible light by the analytes.
- Fluorescence Detectors: These provide higher sensitivity for specific compounds, especially in biomedical applications.
- Mass Spectrometric Detectors: In the context of LC-MS, these are integral, allowing for the determination of molecular weights and structural information, enhancing the capability of LC techniques.
The selection of a detector is influenced by the specific needs of the analysis, such as sensitivity, resolution, and response time.
Fundamentals of Mass Spectrometry
Mass spectrometry (MS) is a pivotal technique in analytical chemistry and biochemistry, significant for its ability to identify and quantify molecules at an unprecedented level of detail. Understanding the fundamentals of mass spectrometry is crucial in the context of LC-MS instrumentation as it combines the separation capabilities of liquid chromatography with the sensitive detection benefits of mass spectrometry. This section will break down essential components and operational principles of MS, bringing clarity to its intricate workings and relevance in contemporary research.
Mass Analyzers Overview
Mass analyzers are the core components of mass spectrometry systems responsible for sorting ions based on their mass-to-charge ratio (m/z). Different types of mass analyzers contribute unique advantages, influencing the choice of mass spectrometry in various applications:
- Quadrupole Mass Analyzers: These settings are widely used due to their robustness and versatility. They operate using oscillating electric fields to filter ions according to their m/z. The quadrupole analyzer is commonly utilized in tandem applications, enhancing the capabilities of LC-MS.
- Time-of-Flight (ToF): ToF analyzers measure the time it takes for ions to travel a set distance. They offer high-resolution measurements and are useful for determining the mass of large biomolecules accurately.
- Ion Trap: Traps ions in a confined space and subsequently ejects them for mass analysis. They allow for high sensitivity and can be used for MS/MS experiments, making them advantageous for detailed structural analysis.
By comprehensively understanding these analyzers, researchers can select the appropriate tool for their specific analytical needs, thereby enhancing the accuracy and reliability of their results.
Ionization Techniques
The ionization process is essential in mass spectrometry as it transforms neutral molecules into charged ions, which can then be manipulated in the mass analyzer. Different ionization techniques impact the type of analysis possible and the molecular information obtained. Common methods include:
- Electrospray Ionization (ESI): Particularly favorable for analyzing biological samples, ESI creates ions from a liquid. It is effective for both large and small molecules and combines seamlessly with LC-MS.
- Matrix-Assisted Laser Desorption/Ionization (MALDI): This method uses lasers to ionize samples embedded in a matrix. It is essential for analyzing high molecular weight compounds like proteins and polymers.
- Chemical Ionization (CI): In CI, gas-phase ions are produced through chemical reactions. This gentle ionization technique provides a softer approach, preserving the integrity of larger molecules.
Different ionization techniques influence the nature of the data obtained in mass spectrometry. (Choose the right technique depending on the sample type and the information needed.)
The choice of ionization method fundamentally affects the mass spectrometry analysis, impacting both the sensitivity and the specificity.
In summary, the fundamentals of mass spectrometry are essential for a thorough understanding of LC-MS technology. By grasping the principles of mass analyzers and ionization techniques, users can better navigate the complex landscape of mass spectrometry and apply it effectively in their research endeavors.
LC-MS Instrumentation Components
The examination of LC-MS instrumentation components is essential, as these elements work together to achieve effective analysis. Each component has its own critical role in ensuring that the LC-MS system operates efficiently and delivers accurate results. Therefore, understanding these components is pivotal for researchers and professionals who depend on LC-MS for their work.
Liquid Chromatography Modules
Liquid chromatography modules are the starting point in an LC-MS analysis. These modules separate the sample into its individual components before they are introduced to the mass spectrometer. The key aspects include:
- Pump Systems: These systems are responsible for pushing the mobile phase through the column. The pressure and flow rate must be precise to achieve optimal separation.
- Columns: The type of column and packing material affect separation efficacy. For instance, C18 columns are often used for their versatility in separating various chemical compounds.
- Autosamplers: These are crucial for automating the injection of samples into the system. They enhance reproducibility and efficiency in processing numerous samples.
Understanding the intricacies of liquid chromatography modules can significantly improve the quality of analyses and enhance method development.
Mass Spectrometer Configurations
Mass spectrometer configurations are at the core of the LC-MS system. They collect and analyze ions generated from the samples. Factors that influence the selection of configurations involve:
- Source Type: Different ionization techniques such as Electrospray Ionization (ESI) or Atmospheric Chemical Ionization (APCI) are utilized depending on the sample type. ESI is great for polar compounds, while APCI suits non-polar samples.
- Mass Analyzer Design: Various designs like Quadrupole, Time-of-Flight (TOF), or Orbitrap impact sensitivity and resolution. Each has distinct advantages depending on the analysis's needs.
- Interface: The interface connects the liquid chromatography and mass spectrometry components. Its design impacts the efficiency of ion transfer and minimizes signal loss.
Familiarity with these configurations allows researchers to make informed decisions tailored to specific analytical needs, ultimately enhancing research outcomes.
Data Acquisition Systems
Data acquisition systems play a pivotal role in LC-MS by capturing signals generated from ions and converting them into analyzable data. Key elements include:
- Software Integration: Advanced software solutions are used to control the LC-MS instrument, process data, and perform initial analyses. Tools like Thermo Scientific's Xcalibur and Waters MassLynx provide user-friendly interfaces for handling complex data.
- Signal Processing: Effective data acquisition systems filter, calibrate, and quantify signal output. Noise reduction and baseline correction are vital for ensuring high-quality data.
- Storage Solutions: Large volumes of data generated require reliable storage options. Solutions often include local servers or cloud-based systems, ensuring improved access and analysis availability for researchers.
"The diversity of components within the LC-MS instrumentation contributes significantly to its versatility, enabling applications across various scientific fields."
By grasping the roles and functionalities of each component in LC-MS instrumentation, individuals involved in scientific research can enhance their methodologies and improve the reliability of their analyses.
Sample Preparation Techniques
Sample preparation is a critical step in Liquid Chromatography-Mass Spectrometry (LC-MS) analyses. It significantly impacts the accuracy, precision, and overall reliability of the results. The main objective of sample preparation is to isolate the analytes of interest from complex matrices. A well-implemented preparation technique can minimize matrix effects, enhance signal detection, and ensure reproducible results.
In the realm of LC-MS, several sample preparation techniques are commonly utilized. Each method has its own advantages, considerations, and specific applications. Understanding these strategies is vital for researchers and professionals aiming to achieve high-quality data in their scientific investigations.
Solid Phase Extraction

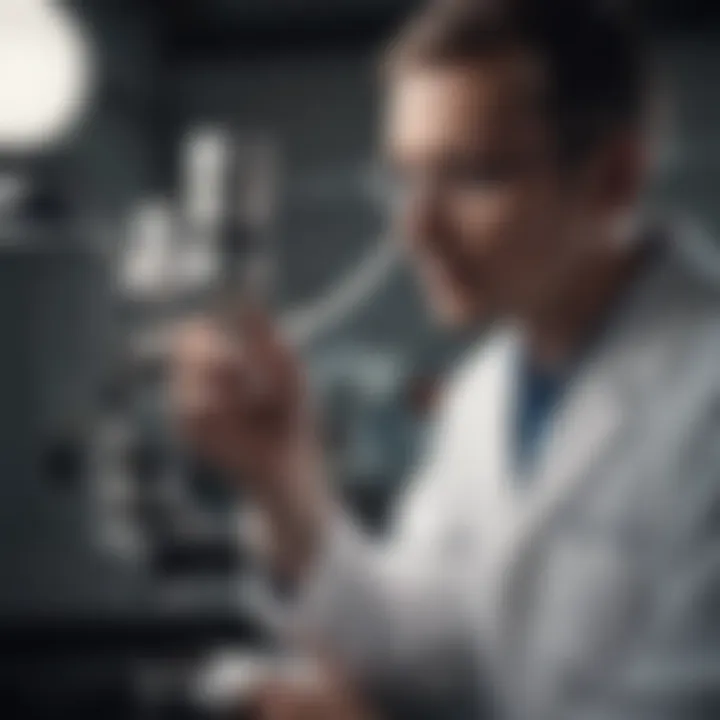
Solid phase extraction, or SPE, is a widely employed method in sample preparation for LC-MS. This technique revolves around passing liquid samples through a solid sorbent material. The analytes of interest are retained on the sorbent, while other unwanted components can be washed away. After this step, the analytes are eluted using a suitable solvent.
Key benefits of Solid Phase Extraction:
- Highly selective: This technique allows selective retention of target analytes, enhancing the purity of the sample.
- Versatile: SPE can be adapted for various types of samples, including biological fluids, environmental samples, and food matrices.
- Easily scalable: It can be efficiently scaled to process larger volumes if needed.
However, careful consideration must be given to the choice of sorbent, as this plays a crucial role in the efficiency and selectivity of the extraction process.
Liquid-Liquid Extraction
Liquid-liquid extraction, or LLE, is another essential technique in sample preparation for LC-MS. This method utilizes two immiscible liquids, typically an organic solvent and an aqueous solution. The solute will preferentially move from one phase to another based upon its solubility.
The advantages of Liquid-Liquid Extraction include:
- Effectiveness for non-polar compounds: LLE is particularly useful for isolating non-polar analytes from polar matrices.
- Simplicity: The procedure can be straightforward and easily implemented in various laboratory settings.
Nonetheless, LLE may be limited by emulsion formation and the need for multiple phases, which can complicate the process.
Filtration and Concentration
Filtration and concentration techniques are often applied as preliminary steps in sample preparation. Filtration involves removing particulate matter or impurities from the sample, ensuring that only the liquid phase enters the LC-MS analysis. On the other hand, concentration techniques aim to reduce the volume of the sample while increasing the concentration of the analytes.
Regarding filtration and concentration:
- Filtration can utilize various materials, like membrane filters or syringe filters, which can effectively eliminate unwanted solids.
- Concentration methods can include techniques such as rotary evaporation or lyophilization, which are beneficial for samples that are dilute or require a higher concentration for accurate quantification.
Analytical Method Development
Analytical method development is a crucial aspect of any scientific investigation that employs Liquid Chromatography-Mass Spectrometry (LC-MS). It not only influences the reliability of the results but also shapes the efficiency of the analytical processes. The importance of this topic lies in its direct impact on the outcome of analyses conducted across various fields, such as pharmaceuticals, environmental science, and biochemistry. A well-developed method ensures that the analytical procedures produce accurate, reproducible, and meaningful results that can withstand the scrutiny of peer review.
Effective analytical method development involves several key elements. First, researchers must define the objectives of their study clearly. This clarity allows them to select appropriate analytical techniques and parameters tailored to the specific characteristics of the sample matrix. Moreover, understanding the target analytes’ properties is essential, as it guides choices regarding extraction techniques, chromatography conditions, and mass spectrometry settings.
Method Validation Principles
Method validation is a fundamental step in analytical method development. Validation provides the necessary evidence supporting the suitability of the method for its intended purpose. It involves a series of tests designed to assess the method’s performance based on several criteria. These include specificity, accuracy, precision, detection limit, quantitation limit, linearity, and ruggedness.
Specificity ensures that the method can accurately identify the analyte of interest amidst potential interferences present in the sample matrix. Accuracy measures how close the results are to the true value; precision assesses the consistency of repeated measurements under the same conditions. Detection limit denotes the smallest amount of the analyte that can be reliably detected, while quantitation limit refers to the lowest concentration that can be quantified accurately.
The principles of method validation often follow guidelines laid out by regulatory bodies, such as the International Conference on Harmonisation (ICH) or the U.S. Food and Drug Administration (FDA). Adhering to these standards guarantees that the developed method meets industry expectations, thereby increasing its credibility.
Optimization Strategies
Optimizing an analytical method is a balancing act, often requiring multiple iterations and adjustments. The primary goal is to enhance the method's sensitivity and specificity while reducing analysis time and consumables. Several strategies can be employed during the optimization phase.
- Chromatographic Conditions: Altering the flow rate, temperature, and mobile phase composition can significantly affect the separation efficiency of analytes in liquid chromatography.
- Mass Spectrometry Settings: Adjusting parameters such as ion source temperature, gas flow rates, and collision energies contributes to better ionization and detection.
- Sample Preparation Method: Refining the sample extraction and concentration techniques can minimize matrix effects and improve the signal-to-noise ratio.
Successful optimization blends theoretical knowledge with practical experimentation. Researchers must be prepared to adjust their methods based on real-world results, continuously refining their approach until the analytical method meets all desired criteria effectively.
In summary, analytical method development is a cornerstone of LC-MS analysis. Through careful validation and ongoing optimization, researchers can ensure that their methods produce reliable, reproducible data that align with the highest standards of scientific inquiry.
Data Interpretation and Analysis
Data interpretation and analysis is a crucial aspect of utilizing Liquid Chromatography-Mass Spectrometry (LC-MS) in contemporary scientific research. This process allows researchers to derive meaningful insights from complex data generated during experiments. Proper interpretation can significantly impact the conclusions drawn, influencing subsequent research directions and applications.
In the context of LC-MS, data interpretation involves understanding the relationship between peaks in mass spectra and the chemical composition of the analyzed samples. Researchers must consider various factors that can affect result validity, such as calibration accuracy, signal-to-noise ratios, and method reproducibility.
Quantitative Analysis Techniques
Quantitative analysis using LC-MS is pivotal for determining concentration levels of specific compounds within a sample. Several key techniques are commonly employed to ensure accurate quantification:
- Calibration Curves: Creating calibration curves with known concentrations allows for comparison against sample data. This linear relationship is fundamental for accurate quantitation.
- Internal Standards: Adding a stable internal standard to samples can compensate for variations in ionization efficiency, ensuring more reliable quantification across different runs.
- Mass Accuracy: Utilizing high-resolution mass spectrometers helps in achieving greater accuracy in mass measurements, which is beneficial for quantitative studies.
When implementing these techniques, one should be mindful of the method validation principles involved, which include specificity, sensitivity, and reproducibility. Each of these elements contributes to robust quantitative analysis that serves as the backbone of many LC-MS applications.
Qualitative Analysis Approaches
Qualitative analysis in LC-MS focuses on identifying compounds and understanding their structural properties rather than quantifying them. This approach is instrumental in various scientific fields:
- Library Matching: Comparing unknown sample spectra against spectral libraries enables the identification of compounds based on matching patterns.
- Metabolite Profiling: LC-MS is extensively used for profiling metabolites in biological samples, which helps in understanding metabolic pathways and biomarker discovery.
- Data Mining Software: Advanced software tools aid in processing large datasets, facilitating efficient identification and characterization of compounds.
Qualitative analysis is often viewed as complementary to quantitative methods. While quantitation provides concentrations, qualitative analysis offers insights into the identities and interactions of compounds, enriching the overall research framework.
The integration of quantitative and qualitative methods in LC-MS analysis enhances the clarity and reliability of scientific data, supporting robust conclusions in research efforts.
In summary, the significance of data interpretation and analysis in LC-MS cannot be understated. Employing thorough quantitative and qualitative techniques affords researchers the ability to extract relevant information, validate hypotheses, and drive scientific advancements.
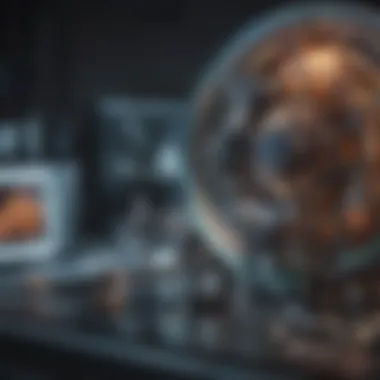
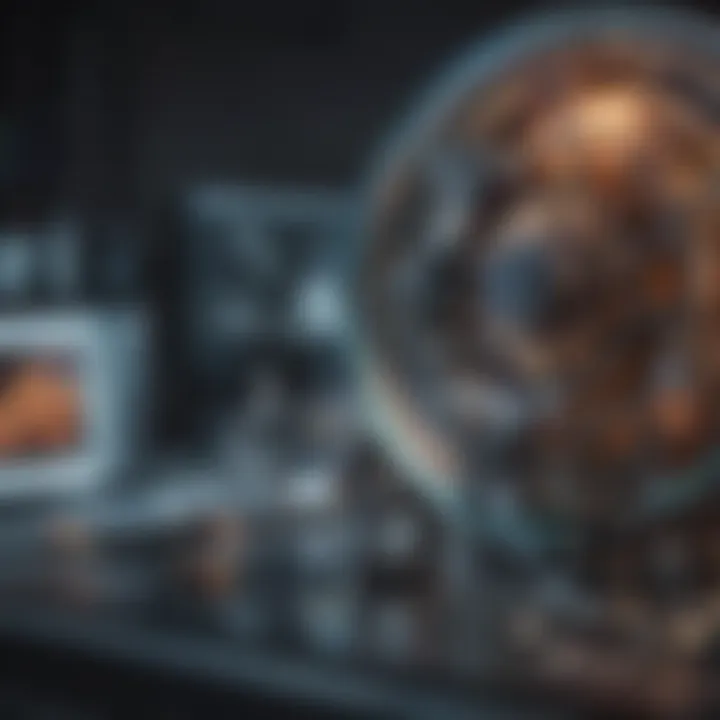
Applications of LC-MS
Liquid Chromatography-Mass Spectrometry (LC-MS) has emerged as a pivotal technique in modern scientific research. The versatility of LC-MS enables it to be utilized across various research fields, each demanding distinct applications tailored to their unique requirements. Understanding the wide-ranging benefits of LC-MS is crucial for many researchers and professionals.
One of the primary advantages of LC-MS is its high sensitivity and specificity. It allows for the detection of low-abundance compounds in complex matrices. This capability is especially valuable in environments where sample integrity is paramount. Furthermore, the technique facilitates quantitative analysis, critical for the evaluation of pharmacokinetics and drug development.
Pharmaceutical Research and Development
In pharmaceutical research, LC-MS plays an essential role in drug discovery and development. By analyzing the metabolism of drugs, researchers can identify metabolites and assess their pharmacological effects. The ability to discern between parent compounds and their metabolites helps in understanding the drug's efficacy and safety.
Using LC-MS, scientists can also conduct stability studies—where they monitor the degradation of drugs under various conditions. This information is critical for formulation development, as it determines the appropriate storage conditions and shelf life of a pharmaceutical product.
Additionally, LC-MS is instrumental in bioanalytical studies. It provides the means to measure drug concentrations in biological fluids. Accurate measurements inform dosage adjustments required for maximizing therapeutic effects while minimizing toxicity. The relevance of LC-MS in addressing regulatory requirements cannot be overstated. Pharmaceutical companies must adhere to strict guidelines for drug testing, and LC-MS facilitates compliance with these regulations.
Environmental Monitoring
Environmental science is another domain where LC-MS showcases its capabilities. This technology is vital for detecting pollutants in various environmental matrices such as water, soil, and air. It enables scientists to monitor contaminants and assess their accumulation and effects on ecosystems.
One key application is the detection of pesticides and heavy metals in agricultural waters. Timely and accurate analysis aids in public health assessments, determining the safety of drinking water. LC-MS also supports efforts to comply with environmental regulations, making it indispensable for environmental chemists and regulatory bodies.
Moreover, LC-MS can identify emerging contaminants, like pharmaceuticals and personal care products, often found in wastewater. Understanding their persistence and effects in the environment is necessary for developing strategies to mitigate pollution.
Proteomics and Metabolomics Studies
Within the fields of proteomics and metabolomics, LC-MS serves as an essential analytical tool. It allows researchers to explore the complexities of biological systems by profiling proteins and metabolites. In proteomics, LC-MS assists in the identification and quantification of proteins, crucial for understanding cellular functions and disease mechanisms.
Similarly, in metabolomics, the technique aids in analyzing metabolic pathways, providing insights into biochemical processes. By understanding how metabolites change in response to various conditions or treatments, researchers can uncover biomarkers for diseases.
In summary, LC-MS contributes significantly to advancing scientific knowledge and technological innovation. Its applications in pharmaceutical research, environmental monitoring, and omics studies underline its importance in contemporary research practices. The potential of LC-MS continues to expand, driving progress across multiple scientific disciplines.
Challenges in LC-MS Analysis
The topic of challenges in LC-MS analysis is crucial to understanding the instrument’s limitations and intricacies. Liquid Chromatography-Mass Spectrometry is widely applied in various scientific fields, yet it is not without its hurdles. Addressing these challenges improves the reliability of results and enhances the overall efficiency of the methodologies.
Matrix Effects and Interferences
Matrix effects are among the most significant challenges encountered in LC-MS. These effects arise when components of the sample matrix influence the ionization process, leading to biased results. The complexity of biological or environmental samples introduces various interferents that may suppress or enhance the detection of target analytes.
Although the ionization mechanism, whether electrospray or matrix-assisted laser desorption, may generally be effective, the presence of salts, proteins, and other matrix components tends to alter the expected outcomes. Therefore, proper sample preparation techniques, such as solid-phase extraction or dilution, should be utilized to minimize such interferences.
To mitigate matrix effects, several strategies can be employed:
- Standardization of Sample Preparation: Consistency in how samples are prepared can greatly reduce variability.
- Use of Internal Standards: Incorporating substances that behave similarly to target analytes helps normalize results.
- Dilution Techniques: Diluting samples before analysis may help alleviate the impact of matrix effects.
"Understanding how matrix components interfere with ionization helps in developing more accurate analytical methods."
Instrument Calibration and Maintenance
The need for precise calibration and regular maintenance of LC-MS instruments is paramount for obtaining reliable data. Proper calibration ensures that the responses of the instrument accurately reflect the concentration of analytes present in the sample. This involves aligning the instrument settings and confirming their accuracy through known standards.
Routine maintenance of the LC-MS system is essential. Regular checks of the ion source, mass analyzer, and other critical components can prevent technical issues that might arise during analysis. In particular, the ion source must be kept clean to preserve its efficiency, while the mass spectrometer’s settings must be carefully monitored for drift or misalignment.
Key practices for maintaining an LC-MS system include:
- Scheduled Cleaning: Frequent cleaning of the ion source and other components helps prevent contamination.
- Performance Checks: Regularly running quality control samples ensures that the system operates within the desired parameters.
- Software Updates: Keeping the data acquisition software up to date is crucial for performance enhancement and bug fixes.
So, understanding and addressing the challenges in LC-MS analysis not only safeguards the integrity of results but also solidifies the instrument's role as a critical tool in contemporary scientific research. This develops a more comprehensive framework for applying LC-MS in various analytical tasks.
Future Trends in LC-MS Technology
The field of Liquid Chromatography-Mass Spectrometry (LC-MS) is rapidly evolving, driven by advances in technology and research needs. Understanding these future trends is crucial for researchers, educators, and professionals, as they determine the direction of scientific inquiry and innovation. As the complexity of biological and environmental samples increases, it is essential that LC-MS systems become more efficient, accessible, and capable of handling diverse applications. This section will explore two promising trends: miniaturization and portability, as well as the integration of artificial intelligence and machine learning.
Miniaturization and Portability
Miniaturization is a significant trend within LC-MS technology. Smaller devices offer numerous advantages over traditional equipment. These systems are often more affordable and require less bench space, which is essential in many laboratory settings. Additionally, portable LC-MS systems facilitate field studies, allowing researchers to analyze samples in situ instead of relying on central labs. The key benefits include:
- Reduced sample volume: Smaller instruments can operate effectively with limited sample sizes, crucial for precious or rare samples.
- Laboratory flexibility: Compact devices can be shifted easily, accommodating various laboratory layouts or workflows.
- Real-time analysis: Portability enhances the capability for immediate sample analysis, especially in environmental monitoring or emergency response scenarios.
Moreover, miniaturization often drives technological innovation. As manufacturers strive to create smaller, more efficient systems, they develop new materials and components that improve performance metrics. This shift not only opens doors to novel applications but also enhances the overall efficiency of existing methodologies. The push for portable solutions in LC-MS is crucial for addressing the evolving demands in various research fields.
Integration of AI and Machine Learning
The incorporation of artificial intelligence and machine learning into LC-MS technology holds transformative potential. These algorithms can significantly improve data analysis, method development, and even instrument operation. Key considerations include:
- Automated data interpretation: AI can handle vast datasets generated by LC-MS systems. By recognizing patterns and trends, it allows for quicker decision-making in research.
- Enhanced method optimization: Machine learning algorithms can assist in identifying the best conditions for sample analysis, reducing development time and improving the robustness of results.
- Predictive maintenance: AI can analyze equipment performance data to anticipate failures, enabling proactive maintenance. This reduces downtime and extends instrument lifespan.
"The integration of AI in LC-MS is not just about efficiency, it's about transforming the way we approach complex analytical problems."
This trend is particularly relevant in high-throughput environments, where the ability to process large volumes of samples and extract meaningful results quickly is paramount. Furthermore, as AI technologies advance, they may contribute to lower operational costs and increased accessibility to state-of-the-art LC-MS techniques.
In summary, the future of LC-MS technology is shaped by miniaturization, portability, and the integration of AI and machine learning. These developments promise to broaden the scope of applications while improving efficiency and accessibility of LC-MS systems in contemporary scientific research.