In Vivo Fluorescence Microscopy: Techniques and Applications
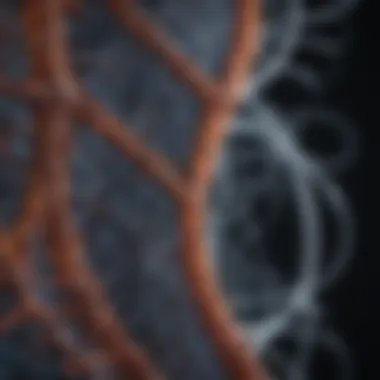
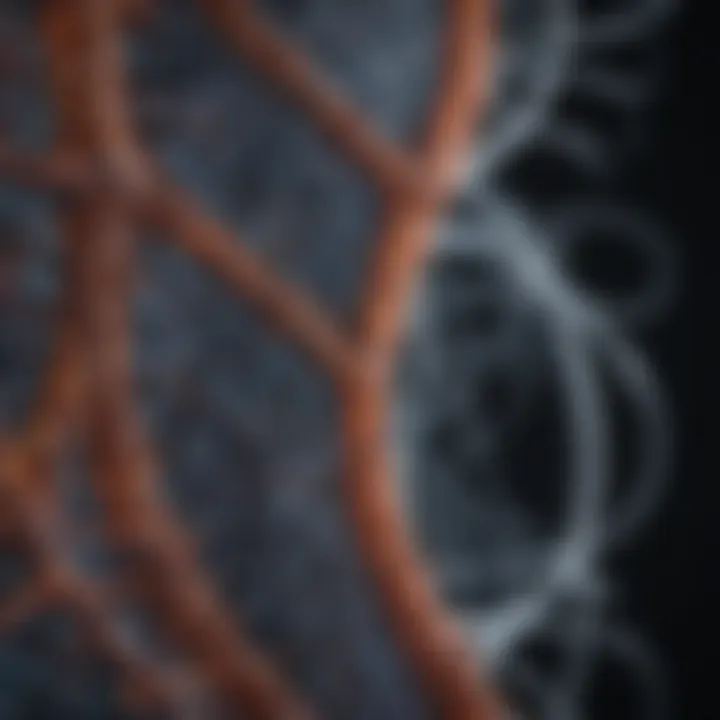
Intro
In the realm of modern biological research, the ability to observe live processes at a cellular level is invaluable. In vivo fluorescence microscopy serves as a key player in this domain, shedding light on an array of biological phenomena. This technique enables scientists to visualize cellular activities in real time, offering a window into the intricate workings of life. When researchers delve into this field, they are often driven by a quest to understand complex concepts such as disease progression, cellular communication, and even the dynamics of neural networks.
Key points to ponder involve not just the established practices but also the evolving nature of in vivo fluorescence microscopy. The significant advancements in technology have also introduced diverse applications, from cancer therapeutics to neurobiology. In this article, we aim to dissect these elements, providing readers, be they students, researchers, or seasoned professionals, with a thorough exploration of its principles and exciting applications.
Methodology
Overview of Research Methods Used
Research within in vivo fluorescence microscopy is multifaceted and requires a robust methodological framework. Typically, the process begins with the selection of appropriate fluorescent markers. Choosing the right markers is crucial, as they must be compatible with the biological targets without interfering with the natural functions of the cells being studied.
Additionally, the imaging systems utilized range from wide-field to two-photon microscopy, each serving different purposes and offering varying depths of imaging. This selection hinges on the specific requirements of the research question at hand.
Data Collection Techniques
Data collection in this space involves several steps:
- Fluorescent Labeling: Cells or tissues are labeled with fluorescent agents, either genetically encoded or chemically synthesized, to visualize specific structures.
- Live Imaging: Continuous imaging allows for changes in cellular dynamics over time to be captured, revealing a narrative of cellular behavior.
- Quantitative Analysis: Advanced software tools help in analyzing the captured images, providing quantitative data on fluorescent intensity and distribution, which can be linked back to biological significance.
"In vivo fluorescence microscopy provides an exceptional resolution and specificity that traditional imaging techniques simply cannot match."
Improvements in camera technology and image processing algorithms have further augmented the capabilities of in vivo fluorescence microscopy. These advancements facilitate capturing high-resolution images with enhanced depth penetration, thus opening new avenues in biological research.
Future Directions
Upcoming Trends in Research
As with many scientific fields, in vivo fluorescence microscopy is on the brink of various innovations. Notable trends include:
- Multi-Modal Imaging: The combination of fluorescence with other imaging techniques like MRI or CT scans, allowing for richer data.
- Expanded Use of AI: Artificial intelligence has the potential to revolutionize imaging analysis, making it faster and more accurate.
- Development of Novel Probes: Researchers are actively working on new fluorescent markers that offer higher specificity and reduced cytotoxicity.
Areas Requiring Further Investigation
While the field is making significant strides, there remains a wealth of unexplored areas. These include:
- Longitudinal Studies: More long-term studies involving live animals or humans could lead to breakthroughs in understanding chronic diseases.
- Increased Spatial Resolution: Advances in technology are needed to discern micro-level interactions within complex tissues.
- Ethical Considerations: As the technology progresses, addressing the ethical implications surrounding in vivo studies will become crucial.
Foreword to In Vivo Fluorescence Microscopy
In vivo fluorescence microscopy represents a profound shift in the realm of biological imaging, allowing scientists to observe living organisms in real-time with unparalleled detail. The ability to visualize biological processes as they unfold in live specimens offers insights that traditional methods simply cannot provide. Not only does this technique enhance our understanding of complex biological interactions, but it also holds the potential to accelerate developments in areas such as drug discovery and regenerative medicine.
Definition and Importance
In vivo fluorescence microscopy is a technique that utilizes fluorescence emission from tagged biological molecules to visualize cellular and subcellular structures. By applying specific wavelengths of light, fluorophores—substances capable of re-emitting light upon excitation—are activated, allowing researchers to track and study various biological processes. The importance of this methodology stems from its capacity to resolve dynamic interactions in the native environment of organisms, extending microscopic observations beyond static images.
The applications of in vivo fluorescence microscopy are vast and significant. Researchers can study cellular behavior during disease progression, observe drug interactions in real-time, and monitor cellular responses during therapeutic interventions. Furthermore, its non-invasive nature minimizes potential pain or harm to living subjects, making it an ideal choice for ethical research practices. Through this lens, one can appreciate not just the how, but also the why of biological phenomena.
Historical Development
The roots of fluorescence microscopy trace back to the early 20th century, when school of thought in cell biology started to embrace the potential of fluorescent dyes. The technique evolved considerably from the first uses of simple fluorescence in microscopy to the development of sophisticated imaging systems that capture high-resolution images of living tissues.
In the 1970s, major advancements were made with the introduction of confocal microscopy, which allowed for clearer imaging by reducing background fluorescence. Later on, in the late 1990s, innovations like two-photon microscopy emerged, revolutionizing depth imaging by enabling examinations deep within living tissues without the damaging effects of traditional methods. Today, the journey continues, as researchers explore novel fluorophores and imaging techniques that enhance the resolution and specificity of in vivo imaging even further.
"In vivo fluorescence microscopy isn’t just a tool; it’s a window into the living world, transforming how we approach biological research."
The growth of in vivo fluorescence microscopy reflects an increasingly detailed understanding of cellular functions and interactions, propelling it into a pivotal position within contemporary scientific research. As we delve deeper into its fundamental principles and applications, it becomes clear that this technique is at the forefront of bridging the gap between basic science and clinical applications.
Fundamental Principles of Fluorescence
Understanding the fundamental principles of fluorescence is crucial for harnessing its full potential in imaging techniques. This section dives into the core mechanisms that allow fluorescence microscopy to illuminate biological processes in living organisms. Recognizing these principles aids researchers in optimizing their experiments, leading to better imaging results and deeper insights into cellular functions.
Fluorescence Phenomenon
The fluorescence phenomenon hinges on the ability of certain molecules, known as fluorophores, to absorb light at specific wavelengths and subsequently emit it at longer wavelengths. This transformation occurs due to the electronic excitation of the molecules, which, upon returning to their ground state, releases energy in the form of light.
The importance of this process can't be overstated, as it forms the backbone of fluorescence-based imaging techniques. By tapping into specific excitation/emission wavelengths, scientists can visually trace certain biomolecules within cells and tissues, providing insight into their functions and distributions. Moreover, the use of multiple fluorescent dyes allows for multiplexing, enabling researchers to visualize several biomolecular events in real-time. This capability is invaluable in dissecting complex biological systems, as it presents a multi-faceted view of cellular dynamics.
Light-Matter Interaction
At the heart of fluorescence lies light-matter interaction. When photons encounter a fluorophore, they can be absorbed if the energy matches the energy gap required for the molecule's excitation. Post-excitation, the molecule enters an unstable state, quickly releasing energy—typically in the form of heat—before emitting a photon of longer wavelength light.
Understanding this interaction encompasses several factors:
- Absorption Spectra: Each fluorophore has unique absorption spectra, dictating which wavelengths can effectively excite it.
- Quantum Yield: This describes the efficiency of the fluorescence process; some fluorophores may absorb light well but emit weakly, resulting in low signal intensity.
- Photostability: This is the stability of fluorophores under illumination; higher photostability allows for prolonged imaging without losing signal, crucial for time-lapse studies.
This comprehensive understanding is vital for researchers as it guides the selection of appropriate fluorophores suitable for their specific experimental objectives. For instance, when studying action potentials in neurons, choosing a fluorophore with high photostability ensures more reliable and consistent readings over time.
Fluorophores: Selection and Characteristics
The choice of fluorophores is a pivotal aspect in the design of any fluorescence microscopy experiment. When selecting fluorophores, researchers must consider the following characteristics:
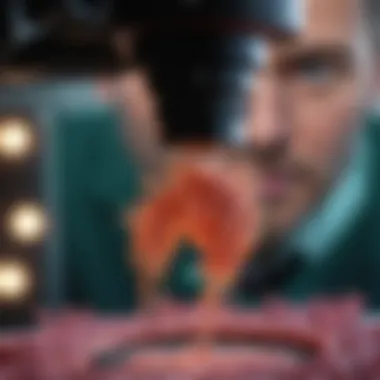
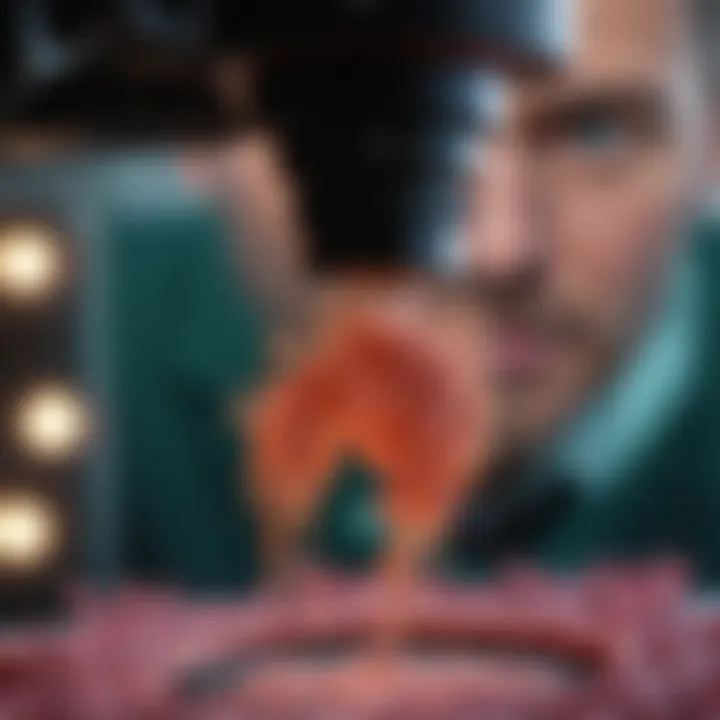
- Emission and Excitation Wavelengths: It’s essential to align the chosen fluorophore's spectra with the microscopy system's light source and filter sets to maximize signal capture.
- Photostability: This impacts the duration and intensity of the fluorescence. More stable fluorophores minimize the risk of photobleaching, maintaining signal strength over extended imaging periods.
- Biocompatibility: Particularly for in vivo studies, the selected fluorophores must be non-toxic to the biological subjects being studied.
- Multiplexing Ability: Some experiments demand the simultaneous visualization of multiple targets. Researchers must select fluorophores with distinct emission spectra to avoid spectral overlap.
Choosing the right fluorophore can make or break an imaging experiment. Opting for a dye that brilliantly illuminates the subject of interest provides a clear window into the microscopic world.
In summary, the fundamental principles of fluorescence—covering the fluorescence phenomenon, light-matter interaction, and fluorophore selection—are critical to understanding how in vivo fluorescence microscopy operates. By mastering these principles, researchers can optimize their methodologies and garner greater insights into the biological processes they aim to study.
Instrumentation for In Vivo Fluorescence Microscopy
The role of instrumentation in in vivo fluorescence microscopy cannot be overstated. It serves as the backbone of the technique, enabling researchers to observe biological phenomena in real time, at the cellular and molecular levels while maintaining the integrity of living organisms. The right set of tools not only enhances image quality but also optimizes the exploration of complex biological processes. Let's delve into the core elements that make up these sophisticated systems:
Microscope Components
Every in vivo fluorescence microscopy system is built on key components that work in harmony. At the heart of it lies the microscope body, which supports various optical elements crucial for imaging. Critical components include:
- Optical Lenses: These are pivotal for magnifying and focusing light onto the specimen. They influence the resolution and clarity of the images captured.
- Filter Sets: Fluorescent imaging relies on specific wavelengths; hence, filters are instrumental in selecting the appropriate emission and excitation wavelengths for fluorophores. Each filter must align with the spectral properties of the chosen dyes.
- Stage: This part holds the specimen and allows for precise movement. The ability to shift the sample accurately in multiple axes ensures that researchers can focus on specific areas of interest in a dynamic living environment.
Considerations:
- The quality of optical components matters; higher-quality lenses and filters lead to better imaging results.
- Calibration of the system ensures accurate measurements and reproducible results.
Detectors and Imaging Systems
The next crucial component involves the detectors and imaging systems, which convert the light that interacts with the fluorophores into digital signals. Common detector types include:
- Photomultiplier Tubes (PMTs): These detectors are known for their sensitivity, particularly useful when working with low light levels.
- Charged-Coupled Devices (CCDs): Widely used due to their ability to capture high-resolution images and their robust performance.
- Scientific CMOS (sCMOS): This newer technology offers higher frame rates and better sensitivity, making it ideal for fast imaging applications.
Effective imaging systems produce high-quality images and allow researchers to capture fleeting biological events.
"The choice of detector can significantly affect both spatial and temporal resolution in fluorescence imaging."
Key Factors:
- Responsiveness of the detector: It should suit the fluorophore’s emission characteristics.
- Frame rate: Higher frame rates are critical for dynamic imaging situations, such as observing cellular processes in action.
Laser and Light Sources
The final piece of the instrumentation puzzle is the laser and light sources, which are essential for exciting the fluorophores. Three main options are frequently utilized:
- Lasers: They provide intense illumination at specific wavelengths, making them highly efficient for fluorescence excitation. For instance, the Argon and Ti:sapphire lasers are popular due to their tunability.
- Light Emitting Diodes (LEDs): These are increasingly used owing to their flexibility, low heat generation, and ease of use. They also offer a range of wavelengths and can be switched on and off rapidly.
- Xenon Lamps: Often give broader spectra and are suitable for applications requiring simultaneous excitation of multiple fluorophores.
Advantages:
- Selective excitation leads to increased contrast and reduced background noise.
- Different sources can enable multi-fluorophore imaging, critical for complex biological interactions.
In summary, understanding the intricacies of the instrumentation used in in vivo fluorescence microscopy is vital for anyone looking to harness this powerful technique in biological research. The combination of top-notch microscope components, suitable detectors, and advanced light sources converge to enhance the ability to visualize the unseen, unlocking insights into the functioning of living systems.
In Vivo Imaging Techniques
In vivo imaging techniques are at the heart of fluorescence microscopy, providing the unique capability to visualize living organisms in real-time. This section aims to underscore the significance of these techniques, showcasing their continuous evolution and application in various fields of biological research. Such techniques not only allow researchers to observe the intricate processes of life but also help in understanding diseases at cellular and tissue levels. This understanding is crucial for developing targeted therapies and improving patient outcomes in numerous medical fields.
Wide-Field Microscopy
Wide-field microscopy is perhaps the most straightforward approach and has been a staple in the fluorescence imaging arsenal for many years. It captures images of the entire specimen simultaneously, producing a large field of view. This technique is particularly beneficial for observing dynamic processes within living cells, like cellular division or movement.
One of the primary advantages of wide-field microscopy is its speed. Researchers can acquire images rapidly, which is vital when studying processes that occur over short periods. However, it does have its downsides; due to the broad illumination of the sample, images may suffer from out-of-focus light contributing to background noise.
In such cases, advanced imaging software and techniques, like deconvolution algorithms, can help enhance image clarity, ensuring that the focus remains sharp on the area of interest.
Confocal Microscopy
Confocal microscopy takes imaging a notch higher by employing a pinhole aperture to eliminate out-of-focus light. This way, it provides clearer images and better resolution than wide-field microscopy, serving researchers well in imaging deeper tissues. The technique is especially instrumental in cellular biology, as it allows for optical sectioning, giving a three-dimensional view of the sample.
Confocal systems can scan points across the specimen, generating high-quality images layer by layer. This feature is invaluable when studying how cells interact in more complex architectures like tissues. Yet, it is essential to note that confocal microscopy requires longer exposure times, which may lead to phototoxicity, affecting live specimens.
Two-Photon Microscopy
Two-photon microscopy is a more specialized technique that utilizes longer wavelengths of light to excite fluorophores. This unique characteristic allows it to penetrate deeper into tissues, making it an ideal choice for in vivo imaging of opaque biological samples.
This method dramatically reduces the damage to cells since it primarily excites fluorophores only at the focal point, lowering phototoxic effects. As a result, researchers can capture stunning images of live tissues over extended periods without causing substantial harm to the specimens. However, it comes with its set of challenges such as the complexity of the equipment and higher cost.
Light Sheet Microscopy
Light sheet microscopy is emerging as a powerful imaging tool, particularly when observing dynamic events within live embryos or tissues. By illuminating the sample with a thin sheet of light, it minimizes photodamage and photobleaching, allowing researchers to watch biological processes unfold in real time.
This technique excels in capturing three-dimensional images in a non-invasive manner. It combines the advantages of both wide-field and confocal microscopy, enabling quick image acquisition with less light exposure. By reducing the risk of altering biological processes, it holds significant promise for studying developmental biology and large-scale imaging applications.
"Each imaging technique brings unique strengths, and understanding them can pave the way for breakthroughs in biological research."
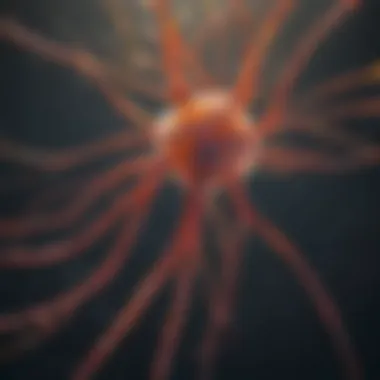
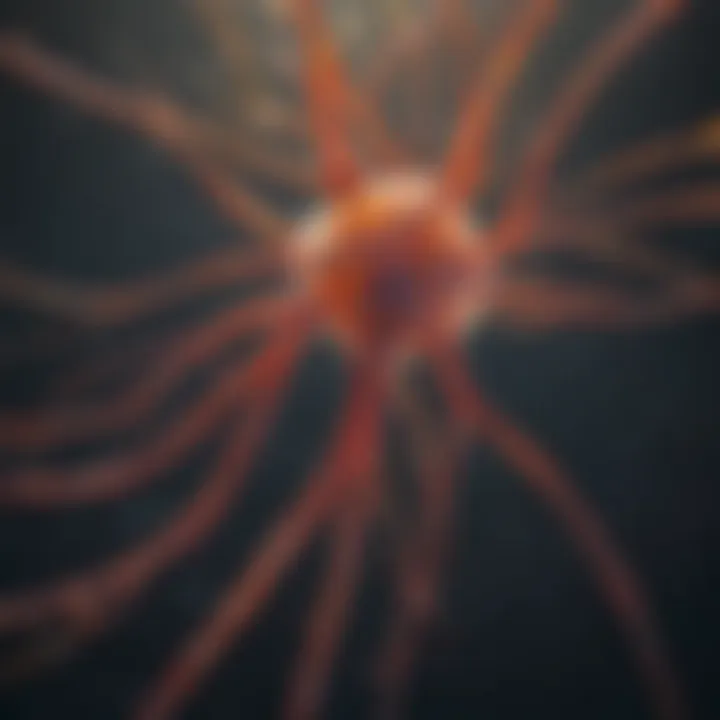
The choice of technique often hinges on the specific needs of the research. Whether one seeks speed, depth, or minimal damage to live cells, in vivo imaging techniques continue to evolve, driving further innovations in exploring the wonders of life.
Applications in Biological Research
In vivo fluorescence microscopy stands as a cornerstone in biological research, offering a window into the dynamic processes within living organisms. The techniques associated with this method have evolved significantly, leading to a richer understanding of cellular and molecular mechanisms. By enabling real-time observation, scientists can gather data that provides insights into various biological phenomena, effectively transforming hypotheses into tangible evidence.
One of the most compelling aspects of in vivo fluorescence microscopy lies in its versatility. The applications stretch across a wide range of fields, from cancer research to neuroscience and developmental biology. Each domain utilizes the technology to address specific biological questions, underpinned by the ability to visualize events as they unfold.
In this section, we will explore key applications of in vivo fluorescence microscopy, highlighting its significance and the specific advantages it offers in advancing our comprehension of biological systems.
Cancer Research
Tracking Tumor Dynamics
Tracking tumor dynamics is a critical component in cancer research that leverages in vivo fluorescence microscopy to visualize tumors in real-time. This method allows researchers to observe the growth patterns and spread of cancer cells within live organisms, setting it apart from traditional imaging tools that capture static images.
The key characteristic of tracking tumor dynamics is its ability to provide continuous insights into tumor behavior and response to therapies. This capability makes it a valuable tool for oncology researchers who aim to understand how different treatments affect tumor proliferation or regression over time.
One unique feature of this approach is the use of specific fluorophores that can bind to tumor cells, elucidating their localization and interaction with surrounding tissues. The advantages are notable; researchers are not restricted to laboratory conditions and can evaluate tumors in their natural environment. Although acquiring high-quality data can be challenging due to tissue absorption, the benefits of gaining real-time insights into tumor progression are unparalleled.
Assessing Treatment Responses
Assessing treatment responses is equally crucial in the context of cancer research. In vivo fluorescence microscopy allows the visual assessment of how tumors respond to various treatments, including chemotherapy and immunotherapy. By linking treatment regimens to observable changes in fluorescence, researchers can gauge efficacy on the go.
What makes this technique particularly beneficial is its non-invasive nature, which means patient welfare is upheld while gathering critical data. The unique aspect of assessing treatment responses is the ability to adapt treatment plans based on real-time feedback, which could result in considerably improved therapeutic outcomes for patients.
However, there are disadvantages; the necessity for precise calibration of fluorophores to ensure accuracy can pose challenges during experimentation. Despite this hitch, the capacity to visually assess and modify treatment plans remains a significant draw in clinical and research settings.
Neuroscience
Visualizing Neural Activity
In the field of neuroscience, visualizing neural activity through in vivo fluorescence microscopy opens doors to previously unattainable insights into brain function. This method allows scientists to observe real-time signaling within neuronal networks, crucial for understanding complex behaviors and neural dynamics.
The ability to visualize neural activity provides an enriching perspective for researchers who delve into the mechanisms of cognition and behavior. In vivo measurement through dynamic fluorescence tagging offers a direct window into neural activity patterns, illuminating the pathways engaged during various tasks.
A noteworthy feature of this approach is the use of genetically encoded calcium indicators, which light up when neurons fire. With these tools, it becomes feasible to investigate the intricacies of neuronal circuits, significantly impacting our knowledge of sensory processing, learning, and memory. While this method provides unique insights, the requirement of specific genetic modifications can limit its broad application, demanding careful consideration.
Studying Neurodegenerative Diseases
Studying neurodegenerative diseases, such as Alzheimer’s and Parkinson’s, employs in vivo fluorescence microscopy to unearth the pathological changes that characterize these conditions. Real-time imaging allows researchers to trace neurodegenerative processes in living organisms, driving forward our understanding of disease mechanisms.
This field thrives on surveying the cellular and molecular events leading to disease progression, and tracking misfolded proteins or abnormal cell death can clarify potential interventions. The core advantage of this technique lies in its ability to capture progressive changes as they occur, providing clear evidence that aids in developing therapies.
However, challenges present themselves when dealing with multifaceted diseases, where the interaction of various factors complicates the interpretation of data. Moreover, the chronic stress to cells caused by fluorescent labeling could lead to artifacts, thus needing careful experimental design.
Regenerative Medicine
Monitoring Stem Cell Behavior
Monitoring stem cell behavior through in vivo fluorescence microscopy is pivotal for advancing regenerative medicine. This technique allows researchers to trace stem cells in real-time, providing insights into the mechanisms of differentiation and cell fate decisions.
The essential quality of this application is its capacity to track stem cells within their native environment, revealing interactions with the surrounding microenvironment crucial for tissue repair. Fluorescence tags make it easier to monitor the behavior of these cells over time, enhancing our understanding of their functionality and potential in healing processes.
A unique feature of using live imaging for this purpose is the ability to witness stem cells migrate, proliferate, and differentiate in real-time. Despite its advantages, the challenge of ensuring stability and minimizing tissue reactions to fluorescent markers can complicate the assessment process.
Analyzing Tissue Repair Processes
Analyzing tissue repair processes through in vivo fluorescence microscopy provides significant insights into wound healing and regenerative mechanisms. The ability to visualize live responses to injury grants researchers a pivotal understanding of the biological events that orchestrate recovery.
What sets this application apart is its focus on the spatiotemporal dynamics of tissue repair, which can inform best practices in regenerative techniques. By utilizing targeted fluorescent markers, scientists can observe how various cells, such as fibroblasts and immune cells, contribute to healing.
While in vivo imaging offers distinct advantages, the difficulty in achieving deep tissue penetration can hinder observations of tissue repair at greater depths. Nevertheless, the insights gained from superficial layers are invaluable and contribute to refining therapeutic approaches and enhancing healing strategies.
Developmental Biology
Observing Embryonic Development
In developmental biology, observing embryonic development via in vivo fluorescence microscopy enables researchers to comprehend complex processes during early life stages. This technology elucidates the sequential events that unfold as embryos develop, contributing to our fundamental understanding of morphology and organogenesis.
The capacity to visualize and record embryonic development in living organisms makes this method indispensable for researchers in the field. It offers a unique opportunity to watch how cells communicate, migrate, and differentiate as the organism grows. Fluorescent markers help identify specific genes and cellular behaviors, offering insights into the developmental inscriptions of various species.
Nonetheless, challenges remain regarding the ethical implications of studying embryonic development along with the technical hurdles related to imaging at different developmental stages. Balancing these factors is crucial but fascinating as it adds layers to our understanding of life itself.
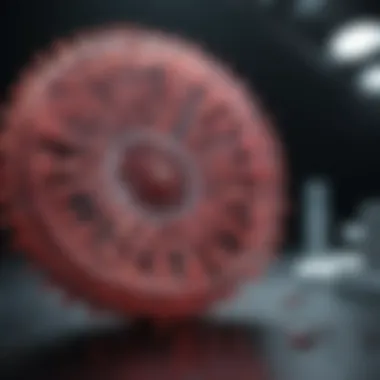
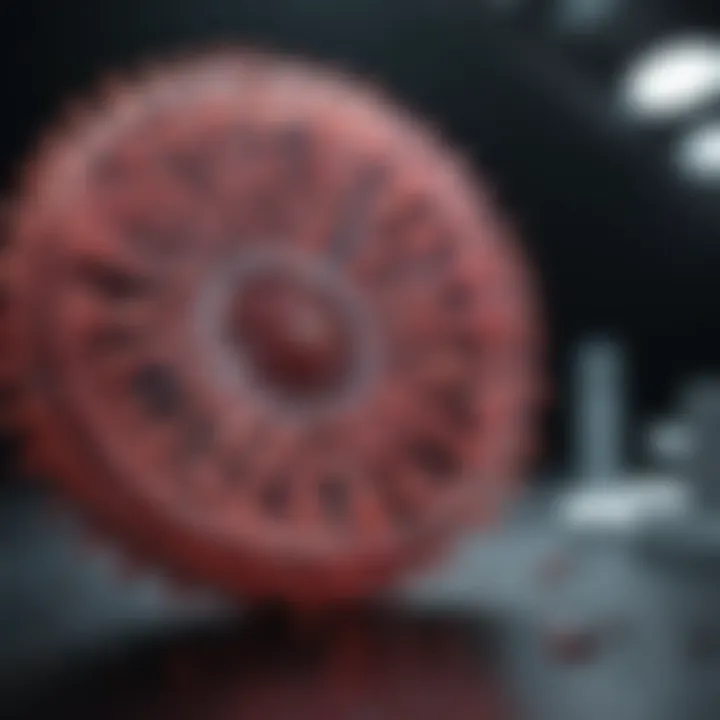
Studying Morphogenetic Processes
Studying morphogenetic processes entails using in vivo fluorescence microscopy to observe how shape and structure emerge within developing organisms. This technique identifies the cellular movements and interactions that yield the final anatomical structures, crucial for grasping how organismal form comes to be.
The significant advantage of using this imaging method revolves around its ability to provide a real-time narrative of developmental transitions. By employing specific fluorescent labels targeting proteins that guide cell shape changes, researchers can delineate the dynamics of morphogenesis effectively.
While the potential to capture intricate cellular processes in action is a major draw, the complexity arising from dealing with various cell types and signaling pathways can complicate analyses. Thus, using this method requires a careful design to tease apart meaningful insights amidst the chaos of development.
Challenges and Limitations
In the rapidly evolving field of in vivo fluorescence microscopy, it is essential to understand not only the techniques and applications but also the challenges and limitations that practitioners may encounter. Grasping these challenges enables researchers to better anticipate difficulties and develop methods to mitigate these issues. Addressing obstacles such as photobleaching, depth of penetration, and signal-to-noise ratio is critical for improving the accuracy and reliability of imaging outcomes.
Photobleaching and Phototoxicity
Photobleaching is one of the most significant hurdles in fluorescence microscopy. This phenomenon occurs when fluorophores irreversibly lose their ability to fluoresce due to prolonged exposure to light. In vivo studies, especially those requiring long observation periods, are particularly susceptible. Photobleaching not only reduces the quality of the imaging but also can lead to misinterpretations of biological processes. Researchers must be cautious when designing experiments to minimize exposure time and consider using more photostable fluorescent probes.
Moreover, along with photobleaching lies the concern of phototoxicity, which refers to the damage inflicted on living tissues due to the high-intensity light necessary for fluorescence imaging. This can adversely affect cellular functions and induce artifacts, skewing results. Balancing optimal imaging conditions while protecting the integrity of the biological sample remains a critical consideration.
Depth of Penetration
Another notable limitation is the depth of penetration of the imaging technique. Fluorescent light can only penetrate biological tissues to a certain degree before scattering diminishes signal intensity. This poses a significant challenge when trying to visualize structures within deeper tissues, like solid tumors or neuronal circuits in the brain. Researchers often have to grapple with finding a balance between sensitivity and specificity of imaging.
Techniques like two-photon microscopy help to overcome some of these depth limitations, as they allow imaging at greater depths with reduced scattering effects. However, these methods can be more complex and may require sophisticated setups and operational skills. This brings into play the idea that optimizing equipment and protocols is key to obtaining clearer images from deeper regions of tissue.
Signal-to-Noise Ratio
Signal-to-noise ratio (SNR) is another pivotal element that influences the quality of fluorescence microscopy. Low SNR can obscure relevant signals and make it difficult to discern meaningful biological information. Factors such as ambient light interference, detector sensitivity, and fluorophore concentration contribute to this challenge.
Practitioners must work diligently to maximize SNR by optimizing illumination settings and employing advanced detection technologies. Methods such as fluorescence lifetime imaging (FLIM) and super-resolution techniques can also enhance SNR, allowing for more subtle biological interactions to be captured.
In summary, while in vivo fluorescence microscopy holds tremendous potential for advancing biological understanding, it is not without its hurdles. A thorough awareness of challenges, including photobleaching, depth of penetration, and signal-to-noise ratio will enable more robust experiment designs and lead to more reliable interpretations of results. Understanding and tackling these limitations not only enhances the effectiveness of fluorescence microscopy but also fosters innovative solutions that can push the boundaries of current biomedical research.
Future Directions in In Vivo Imaging
In vivo fluorescence microscopy stands at the crossroads of significant advancements and evolving challenges, paving the way for its enhancement and application across multiple scientific disciplines. This section sheds light on potential future trajectories which could revolutionize how researchers perceive biological systems. By focusing on three core areas—technological innovations, interdisciplinary approaches, and clinical applications—this narrative aims to illuminate the importance of these directions in the context of in vivo imaging.
Technological Innovations
Advancements in technology play a crucial role in shaping the future of in vivo fluorescence microscopy. Enhancements in imaging speed, resolution, and sensitivity are being driven by several key innovations:
- Multi-Photon Excitation Techniques: These reduce phototoxicity and enable imaging deeper into living tissues. They can capture rapid dynamic processes such as cellular interactions, an area where traditional methods fall short.
- Adaptive Optics: By compensating for distortions in light, adaptive optics can drastically improve image quality. Such technology may provide unprecedented clarity in imaging cellular structures in real-time.
- Portable and Miniaturized Systems: There’s a push for smaller, user-friendly devices that can be used in various settings, making fluorescence microscopy accessible outside of traditional lab environments. Devices like the miniaturized fluorescence microscope, which can be deployed for field studies, highlight this trend.
With these technologies, the overall efficiency and efficacy of in vivo imaging will see a marked improvement, possibly unto an era of personalized medicine where imaging technologies can be standard practice in biological assessments.
Interdisciplinary Approaches
The future of in vivo fluorescence microscopy is not just confined to technological leaps; it also leans heavily on interdisciplinary collaboration. Different fields coming together often herald new insights and applications. Here are some pathways to consider:
- Collaborations Between Fields: Combining expertise from physics, biology, and materials science can engender innovative solutions. For instance, physicists may create new light sources, while biologists utilize these advancements to further study cellular behavior.
- Integration with Computational Biology: Data generated from imaging needs robust analysis techniques. By harnessing inter-disciplinary computational approaches, researchers can delve deeper into the data, revealing patterns and relationships that might go unnoticed otherwise.
- Application in Environmental Studies: Bringing in expertise from environmental sciences could lead to new applications for in vivo fluorescence microscopy—monitoring organisms in their natural habitats. This can have vast applications in conservation efforts.
These collaborative efforts can foster a deeper understanding of complex biological systems, enhancing both educational frameworks and research outcomes.
Potential for Clinical Applications
In the realm of healthcare, the promise of in vivo fluorescence microscopy is particularly compelling. Its integration into clinical practice could potentially revolutionize diagnostic and therapeutic landscapes:
- Early Detection of Conditions: In vivo imaging techniques can facilitate the early detection of cancers or other diseases by visualizing changes in tissue at the molecular level. Detecting diseases before they manifest fully is paramount in improving patient outcomes.
- Surgical Guidance: With improved imaging, surgeons could gain real-time insights during procedures, ensuring greater precision in minimally invasive surgeries. This advancement would minimize complications and promote faster recovery.
- Personalized Medicine: Tailoring treatments based on specific cellular responses observed through fluorescence microscopy could significantly enhance therapeutic efficacy and promote better patient compliance.
By harnessing in vivo fluorescence microscopy, the healthcare industry could shift towards more preventive and personalized strategies, benefitting both patients and practitioners.
A strong focus on these future directions will undoubtedly propel in vivo fluorescence microscopy onto new frontiers, solidifying its pivotal role in advancing scientific research, healthcare, and beyond.
"The intersection of innovative technology and interdisciplinary collaboration opens up limitless avenues for discovery, enhancing our understanding of life at every level."
End
The conclusion serves as the compass that directs the reader back to the essential insights gleaned throughout the discourse on in vivo fluorescence microscopy. It underscores the multidimensional importance of the subject matter discussed and reflects on its far-reaching impact across various scientific fields. Ultimately, the key takeaways crystallize the critical thinking applied to the challenges and advancements in this imaging modality.
Summary of Key Points
To encapsulate the core themes of this article, a few significant points are worth revisiting:
- Fundamental Principles: The basic principles governing fluorescence microscopy, including the crucial interactions of light and matter, set the groundwork for understanding more complex techniques.
- Diverse Imaging Techniques: A variety of imaging strategies such as confocal and two-photon microscopy broaden the applicability of in vivo imaging, granting researchers the tools to investigate biological phenomena in unprecedented detail.
- Real-World Applications: The utility of this technology in cancer research, neuroscience, and regenerative medicine illustrates its versatility and critical role in advancing scientific knowledge.
- Challenges and Future Directions: Addressing the limitations such as depth of penetration and photobleaching enables ongoing improvements and innovative techniques that can further enhance imaging capabilities.
In essence, these points form the backbone of in vivo fluorescence microscopy's narrative, highlighting its significance in the journey of scientific exploration.
Implications for Future Research
The horizon ahead for in vivo fluorescence microscopy is bright and teeming with potential. With continual research, several implications stand out for the future:
- Integration of Emerging Technologies: The fusion of in vivo fluorescence microscopy with other imaging modalities, such as magnetic resonance imaging (MRI) or positron emission tomography (PET), may yield a more holistic view of biological processes.
- Refinement of Fluorophore Applications: There's a growing need for tailoring fluorophores that are more photostable and less toxic, thereby improving the quality and safety of imaging studies.
- Advancements in Data Analysis: The rise of artificial intelligence and machine learning can transform how we analyze the vast amounts of data generated, making sense of complex biological interactions faster and more accurately.
- Broader Clinical Adoption: As the technique becomes more refined and accessible, the potential for clinical applications in diagnostics and treatment planning becomes increasingly tangible, promising to revolutionize patient care.