Hydrogen Gas Detection: Techniques and Innovations
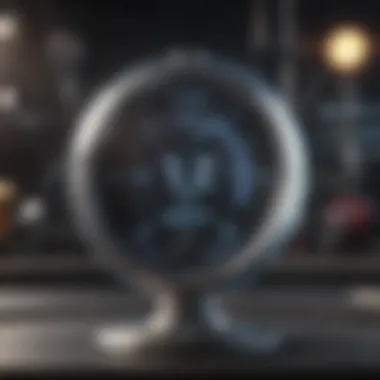
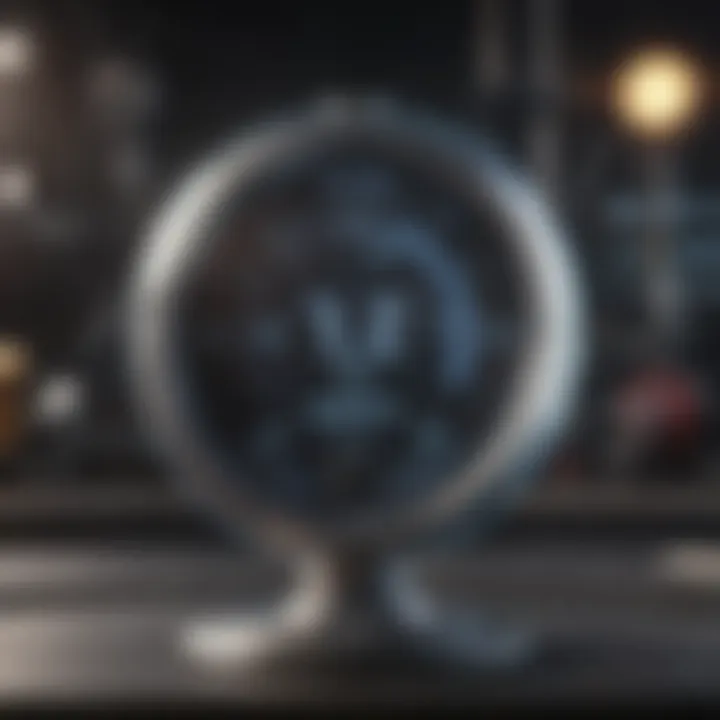
Intro
As we stand at the crossroads of energy innovation and environmental safety, understanding hydrogen gas detection becomes crucial. Hydrogen, with its rising prominence in green energy technologies, presents not just opportunities but also formidable challenges. Its infamous tendency to diffuse quickly and its odorless, colorless nature complicate detection efforts. Recognizing these intricacies helps illuminate why accurate detection methods are essential across multiple industries, from automotive to energy production.
In the face of these complexities, various detection methods have emerged, each with its own strengths and weaknesses. By delving deeper into these approaches, along with the accompanying obstacles researchers encounter, we can appreciate the ongoing innovations in this field. This exploration will lay the groundwork for comprehending the role hydrogen plays in our future energy landscape.
Methodology
Overview of research methods used
When it comes to detecting hydrogen gas, researchers employ a multitude of methods, each divergent in approach yet unified in purpose. Primarily, these methods can be categorized into physical, chemical, and electrochemical detection techniques. Each category employs unique principles, shaping the feasibility and effectiveness of hydrogen detection in various conditions.
Physical Methods encompass techniques such as mass spectrometry and gas chromatography. These allow for quite precise measurements, yet they can be expensive and require sophisticated equipment.
Chemical Methods focus on the interaction of hydrogen with other substances, producing measurable byproducts. Commonly, these might include colorimetric testing, where the presence of hydrogen can lead to a visible change in color.
On the other hand, Electrochemical Sensors stand out due to their adaptability and lower costs. They typically rely on the oxidation-reduction reaction of hydrogen at an electrode, enabling real-time monitoring. This makes them particularly attractive for use in industrial applications.
Data collection techniques
Data collection for hydrogen detection typically revolves around real-time monitoring systems equipped with various sensors. These sensors generate data that can be analyzed to assess hydrogen concentrations and fluctuations, thus signaling potential hazards. Researchers analyze the incoming data using various statistical methods to ensure reliability and accuracy.
Moreover, field testing is an integral aspect at which sensors are employed in actual operational environments. This hands-on approach provides valuable insights into real-world performance and helps pinpoint areas for refinement.
Future Directions
Upcoming trends in research
Looking ahead, the field of hydrogen detection is ripe for advancement. One notable trend is the integration of smart sensor technology. This approach combines traditional measurement methods with internet connectivity, allowing for enhanced real-time monitoring and remote analysis.
Another area of focus is improving the sensitivity and selectivity of sensors. Innovations in nanomaterials have shown tremendous potential to boost sensor performance significantly. Researchers are experimenting with nano-coatings, which provide not only increased surface area for reaction but also improved resilience under challenging operating conditions.
Areas requiring further investigation
Despite the advances made, several areas remain under scrutiny. For instance, the long-term stability of sensors in harsh environments still poses a challenge. Understanding the degradation mechanisms of sensor materials can aid in designing more durable instruments.
Furthermore, cross-sensitivity to other gases is a major concern for accurate hydrogen detection. Exploring methods to minimize these interferences is vital for achieving high precision in measurements.
With expanded research in these directions, the hope is to create robust solutions that enhance the safety and efficiency of hydrogen applications across various sectors.
"Investing in rigorous research now may yield dividends in safety and efficiency down the road, safeguarding our transition to a hydrogen economy."
Intro to Hydrogen Gas Detection
Detecting hydrogen gas is a matter that spans various fields, stretching across industries, research laboratories and safety sectors. The importance of this topic cannot be understated, as hydrogen, despite being the simplest and lightest element, bears a significant weight in terms of potential dangers and practical applications. Considering that hydrogen is highly flammable and can form explosive mixtures in air, implementing effective detection methods is essential to safeguard human life, property, and the environment. The correct identification of hydrogen gas leaks is critical—not just for maintaining compliance with safety regulations but also for fostering trust among stakeholders.
Importance of Hydrogen Gas Detection
Hydrogen gas detection serves multiple significant purposes. Firstly, from a safety standpoint, timely identification of hydrogen leaks not only helps in preventing explosions but also protects workers in various environments, especially in industries where hydrogen is a common by-product, such as petrochemical, aerospace, and electronics.
Secondly, as renewable energy sources like hydrogen fuel cells gain momentum, the need for accurate gas detection grows. This technology underpins many sustainable practices by ensuring safe utilization of hydrogen as a clean energy carrier.
Additionally, effective hydrogen detection can minimize environmental impacts. In settings such as landfill and wastewater management, monitoring hydrogen emissions can contribute to reduced greenhouse gas outputs.
Applications in Various Industries
The versatility of hydrogen detection methods stretches across myriad industries. Here’s a look at some critical applications:
- Petrochemical Industry: Routine monitoring of hydrogen, which is ever-present during refining processes, is paramount to avoid accidents and ensure proper functioning of systems.
- Energy Sector: Hydrogen fuel cells are increasingly utilized in transportation and stationary power generation, calling for reliable detection methods to ensure operational safety.
- Automotive: In hydrogen-powered vehicles, sensors are essential to detect potential leaks, ensuring passenger safety while travelling.
- Aerospace: In spacecraft and aircraft, precise hydrogen monitoring is crucial either for fuel systems or during hydrogen production for propulsion experiences.
- Research Facilities: Laboratories handling hydrogen for experimental purposes must integrate detection systems to create a secure working atmosphere.
"Effective hydrogen gas detection can minimize environmental impacts and ensure worker safety across all sectors involved."
With such broad applications, it’s clear that strengthening hydrogen detection technologies not only enhances safety but also bolsters efficiency in various industries.
Chemical Properties of Hydrogen Gas
Understanding the chemical properties of hydrogen gas is pivotal in comprehending its behavior, applications, and implications in various sectors. Hydrogen, the simplest and most abundant element in the universe, presents unique characteristics that influence its detection and safety measures. Among these properties, its physical traits and reactivity define how it interacts with other substances, making it crucial for engineers, safety officials, and researchers alike to grasp these fundamentals.
Physical Characteristics
Hydrogen gas is colorless, odorless, and tasteless, which makes it a tricky character in terms of detection. Because it is lighter than air, it disperses rapidly when released. This property can be a double-edged sword. On the one hand, it can help mitigate immediate containment issues, but on the other, its propensity to rise means that leaks can be hard to spot.

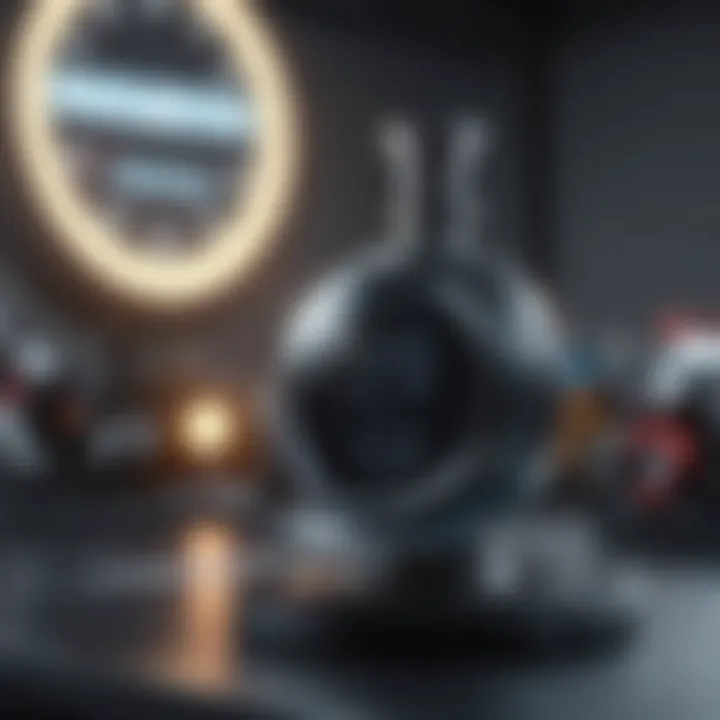
- Molecular Structure: Hydrogen exists primarily as diatomic molecules (H₂), which are formed when two hydrogen atoms bond together. This form is crucial because the diatomic structure facilitates various reactions, particularly in energy generation.
- Density and Diffusion: With a density of about 0.0899 g/L at standard conditions, hydrogen gas is significantly less dense compared to air. This property results in fast diffusion, which is essential in applications like buoyant gas balloons and potential future hydrogen-fueled vehicles.
Reactivity and Combustion
Hydrogen is inherently reactive, primarily due to its electron configuration. It has the ability to form compounds with a myriad of elements, making it a versatile player in chemical reactions. When mixed with oxygen and ignited, hydrogen exhibits its most dramatic characteristic: combustion. The resulting reaction produces water vapor and generates substantial energy, a phenomenon eagerly harnessed in various applications, from rocket propulsion to powering fuel cells in electric vehicles.
- Combustion Characteristics: The combustion of hydrogen is vigorous and exothermic. When hydrogen burns, it forms a high-temperature flame that is nearly invisible in daylight, adding another layer of danger in leak scenarios.
- Controlled Reactions: In controlled environments such as fuel cells, hydrogen reacts electrochemically with oxygen to produce electricity, thereby showcasing its utility beyond traditional combustion engines.
"The potential uses of hydrogen gas are vast, but understanding its properties is crucial for harnessing this power safely and efficiently."
Focusing on these properties does not only help inform effective detection methods but also plays a role in ensuring safety protocols across industries. Whether in an industrial application or in research laboratories, delving into these fundamental characteristics lays the groundwork for innovations in detection technologies. As the world moves towards sustainable energy solutions, grasping the nuances of hydrogen gas's chemical properties becomes not just beneficial but fundamental.
Fundamental Detection Principles
The detection of hydrogen gas hinges on a variety of fundamental principles that guide the design and functionality of sensors employed in different settings. Understanding these principles is crucial not just for enhancing technology but also for ensuring safety and efficiency in industrial applications. The core idea is to identify the presence of hydrogen gas accurately and reliably, given its highly flammable nature and the significant safety risks associated with leaks. This section will delve into three major detection techniques: electrochemical methods, optical sensors, and thermal conductivity measurements, each offering unique benefits and considerations.
Electrochemical Detection Methods
Electrochemical detection methods are among the most widely used in detecting hydrogen gas due to their robustness and sensitivity. These methods work on the principle of measuring the current produced by a chemical reaction when hydrogen gas interacts with a specific electrode material. In a nutshell, when hydrogen gas contacts the electrode, it undergoes an oxidation process, thus generating an electrical signal proportional to its concentration. The main advantage of electrochemical sensors is their low detection limit; they are capable of identifying even minute traces of hydrogen, making them ideal for environments where safety is paramount.
However, there are several considerations to keep in mind. The lifespan of these sensors can be relatively short, especially if exposed to high gas concentrations or harsh environments. Moreover, they are subject to interference from other gases, potentially leading to false readings. Thus, ongoing advancements aim to improve the stability and selectivity of electrochemical detectors, enabling them to function more effectively in real-world scenarios.
Optical Sensors
Optical sensors, on the other hand, utilize the properties of light to detect hydrogen gas. These sensors generally rely on the principles of absorption spectroscopy or scattering techniques. When a light source passes through a sample containing hydrogen, the molecules absorb specific wavelengths of light based on their unique spectral characteristics. By analyzing the light that passes through, the system can quantify the amount of hydrogen present.
One major advantage of optical sensors is their ability to provide real-time data without requiring direct contact with the gas. This characteristic minimizes the risk of sensor degradation due to environmental factors. Additionally, they are less prone to interference from other chemicals, allowing for a more accurate reading. However, they can be more expensive and may require sophisticated equipment for analysis.
Thermal Conductivity Measurements
Thermal conductivity measurements represent yet another approach in hydrogen detection, measuring how the presence of hydrogen alters the thermal conductivity of a gas mixture. The method involves passing heat through the gas; since hydrogen has a lower thermal conductivity compared to many other gases, the change in heat transfer can indicate its concentration within the mixture.
This technique is particularly beneficial in environments where fast response times are crucial, as it can provide immediate feedback on gas concentration levels. Moreover, thermal conductivity sensors generally exhibit high durability, capable of withstanding fluctuating conditions. However, they usually require calibration to account for the presence of other gases, and they may not be as sensitive to lower concentrations of hydrogen as other methods.
In the upcoming sections, we will further explore the various types of hydrogen detection sensors, the challenges faced in their implementation, and the recent innovations emerging in this vital field.
Types of Hydrogen Detection Sensors
When it comes to detecting hydrogen gas, the type of sensor utilized can significantly impact accuracy, response time, and overall performance in various applications. Selecting the right detection method is not just a matter of preference; it fundamentally affects safety measures, environmental monitoring, and industrial processes. Each sensor type has its advantages and drawbacks, making it crucial to understand the distinct characteristics and functionality of individual technologies.
Metal-Oxide Semiconductor Sensors
Metal-oxide semiconductor sensors are among the most commonly used devices for hydrogen detection. These sensors operate on the principle of changing resistance in response to hydrogen presence. When hydrogen gas comes into contact with the metal-oxide layer, it reacts and alters the electrical resistance of the sensor, leading to measurable changes. This kind of sensor is valued for its sensitivity and relatively low cost, making it attractive for a range of applications.
- Advantages:
- Considerations:
- Low production costs
- Quick response times
- Suitable for a variety of environments
- Sensitivity to other gases can lead to false readings
- Temperature variations may affect performance
Utilizing metal-oxide sensors, industries can effectively enhance their hydrogen detection protocols, but they must also take care to calibrate these devices regularly to ensure reliability.
Catalytic Bead Sensors
Catalytic bead sensors utilize a different approach to detect hydrogen gas. These devices employ a small bead that is coated with a catalyst material, typically platinum. When hydrogen is present, it reacts with the catalyst and causes the bead to heat up. This change in temperature generates a measurable electrical signal, which translates into a hydrogen concentration reading. Ideal for explosive environments, these sensors are favored in safety-critical applications.
- Advantages:
- Considerations:
- Reliable and robust operation in hazardous areas
- Maintains accuracy even in varying conditions
- Requires careful calibration to maintain accuracy
- More expensive than some alternatives
Catalytic bead sensors provide a balance between performance and safety, truly standing out where explosive risks are a concern, thereby allowing industries to maintain high safety standards.
Fiber-Optic Sensors
Fiber-optic sensors represent a newer frontier in hydrogen detection. These sensors utilize light transmission through optical fibers, making them less susceptible to electromagnetic interference. The principle of operation revolves around changes in light transmission properties when hydrogen interacts with special coatings on the fibers. This approach grants exceptional sensitivity and the ability to operate in harsh environments.
- Advantages:
- Considerations:
- High sensitivity and specificity to hydrogen
- Ideal for monitoring in challenging environments
- Minimal maintenance required
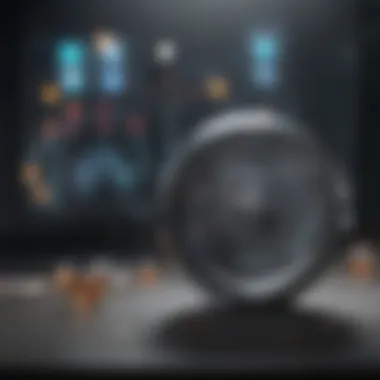
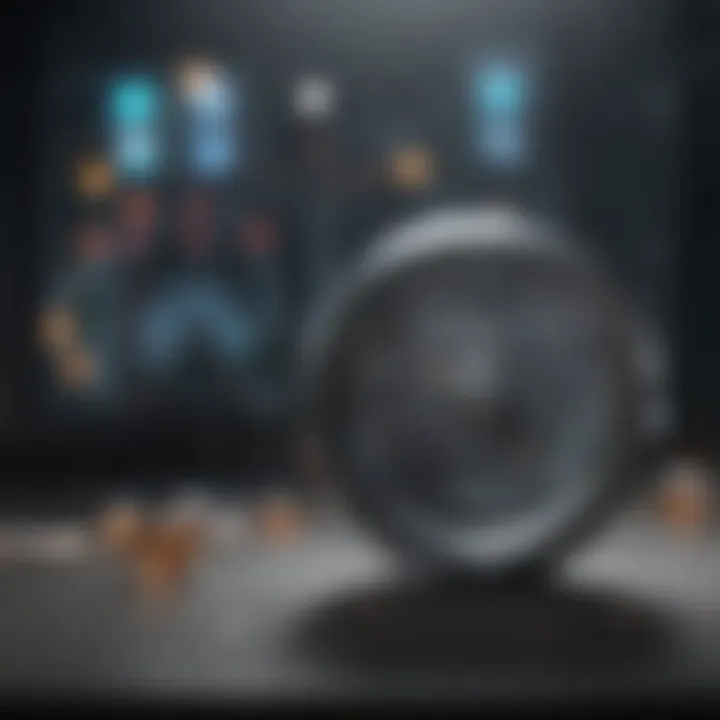
- Higher initial setup cost
- Technical complexity in installation
Fiber-optic sensors might seem a bit costlier upfront, but their performance and low maintenance needs can justify the investment over time, particularly in specialized applications like research labs and safety monitoring tasks.
In summary, choosing the right type of hydrogen detection sensor is essential for meeting safety, cost-effectiveness, and operational efficiency. With the advancement of sensor technology, understanding these types will equip industries to tackle challenges effectively.
Challenges in Hydrogen Detection
Detecting hydrogen gas is not just about finding the presence of this elusive molecule; it’s a complex challenge that encompasses various technical and environmental factors. Understanding these challenges is essential for advancing detection technology and ensuring safety in numerous applications. Hydrogen, being the simplest and lightest element, presents unique problems that must be tackled diligently. This section will delve into the critical issues faced in hydrogen detection, emphasizing their implications for industries reliant on precise measurements.
Sensitivity and Selectivity Issues
Hydrogen sensors need to be both sensitive and selective. Sensitivity refers to the ability of a sensor to detect small concentrations of hydrogen, while selectivity ensures that it correctly identifies hydrogen without interference from other gases.
- Sensitivity: Many industries operate in environments filled with various gases. This makes the ability to detect low levels of hydrogen crucial. For instance, hydrogen is often present alongside methane or carbon monoxide, and sensors must accurately respond to changes in hydrogen concentration even at parts per million levels.
- Selectivity: A sensor that reacts to multiple gases can lead not only to false alarms but also to dangerous situations, where the detection of one gas could mask the presence of hydrogen. This selection process can be extremely challenging, as compounds can often have similar chemical signatures.
In short, achieving a high level of both sensitivity and selectivity remains a pivotal hurdle. Sensors need to balance these two parameters to prevent erroneous readings that can have serious safety implications.
Interference from Other Gases
One of the most significant challenges in hydrogen detection is the interference from other gases present in the same environment. Hydrogen sensors may respond to other combustible gases, leading to inaccurate measurements that can misjudge the safety conditions in a given setting.
- Common Culprits: Several hydrocarbons, such as propane and butane, can produce false readings in hydrogen detectors. This interference can be particularly problematic in natural gas facilities or chemical plants where a mixture of gases may be prevalent.
- Environmental Effects: Factors such as humidity, pressure changes, and temperature can also affect sensor performance. For example, high humidity can lead to condensation on sensors, altering their readings or even causing failure in extreme cases.
To mitigate these issues, researchers are developing more sophisticated algorithms to better interpret sensor data, but the complexity of gas interactions continues to pose a challenge.
It is imperative for safety regulations to evolve alongside detection technologies to address these inherent challenges, thereby ensuring that hydrogen detection is both reliable and safe in all applications.
Recent Innovations in Detection Technology
In the rapidly evolving field of hydrogen gas detection, adopting cutting-edge innovations is paramount. As industries become more aware of the potential hazards associated with hydrogen, advancing detection technology not only enhances safety protocols but also contributes to more efficient energy solutions. Recent innovations in detection technology are addressing definable challenges, ranging from sensitivity and specificity in sensor readings to the integration of smart technologies that empower proactive risk management. Understanding these breakthroughs allows industries to improve operational safety while supporting broader energy initiatives.
Nanotechnology in Sensor Development
Nanotechnology has transformed various scientific fields, and hydrogen gas detection is no exception. Sensors developed using nanomaterials—like carbon nanotubes or metal oxide nanostructures—offer significantly enhanced sensitivity compared to traditional sensors. These nanoscale materials can detect quantities of hydrogen gas at much lower thresholds, which is crucial for preventing potential leaks before they escalate into serious threats.
One core advantage of nanotechnology in sensor design is the increase in surface area relative to volume, which means more active sites for hydrogen molecules to bind. This results in faster response times, making it possible to react quickly in an industrial environment where conditions can change rapidly. Additionally, the lightweight and compact nature of nanomaterials allows for portable sensor devices, facilitating on-site monitoring in a range of settings.
"The integration of nanotechnology in sensor development has redefined detection accuracy, potentially saving lives and reducing significant financial losses in industries."
Smart Technology Integration
The rise of smart technology integration into hydrogen detection systems marks a significant leap forward. Modern sensors often come equipped with IoT (Internet of Things) capabilities, allowing them to communicate data in real-time to monitoring systems. This fusion of technology enables not only immediate alerts for hydrogen detection but also historical data logging, which can help identify patterns or potential sources of leaks.
One notable example is the use of cloud-based platforms that analyze data collected from various sensors across different locations. This enables businesses to create a comprehensive map of hydrogen concentration levels in their facilities, leading to more informed decision-making. Furthermore, integrating artificial intelligence algorithms can predict potential risk zones based on past data, thus helping to ensure safe operations.
Companies investing in such smart integrations can expect increased compliance with safety regulations, improved response times in emergencies, and a reduction in maintenance costs associated with manual monitoring. This approach not only boosts operational efficiency but also enhances a company’s reputation, as stakeholders increasingly prioritize safety and environmental responsibility.
Overall, the recent innovations in detection technology hold immense promise for not just enhancing safety protocols around hydrogen gas but also paving the way for sustainable practices in energy usage.
Regulatory Standards and Guidelines
The realm of hydrogen gas detection is not only an area of scientific curiosity but also one of substantial regulatory significance. Regulatory standards establish a baseline for safety and operational efficiency in various industrial and research settings. The importance of these standards cannot be overstated, as they guide the manufacturing, testing, and implementation of detection technologies crucial for maintaining a safe environment while handling hydrogen gas.
Understanding these guidelines ensures that both researchers and organizations prioritize safety. Compliance with established standards serves two key purposes. First, it protects personnel and the environment from the potential hazards associated with hydrogen gas. Second, it fosters industry-wide confidence in the technologies utilized for detection, thereby enhancing overall system reliability.
Global Standards
Various organizations and governing bodies have laid down specific global standards for hydrogen detection. These guidelines cover a multitude of factors, including sensor performance, calibration methods, and operational protocols. For instance, standards set by the International Electrotechnical Commission (IEC) provide clarity on the technical specifications required for hydrogen sensors. This encompasses aspects like sensitivity, response time, and the ability to function accurately in varying environmental conditions.
Moreover, adherence to ISO 26142 outlines a standardized method for measuring hydrogen concentrations in different settings. This gives an internationally recognized framework that manufacturers can follow. The implementation of such global standards promotes interconnectivity and comparability of hydrogen detection technologies, allowing for collaborative advancements across borders.
It's important to note that not following these standards can lead to dire consequences. Unsafe practices in hydrogen detection might not only compromise the integrity of a facility but also endanger human lives.
Industry Compliance
Compliance with regulatory standards in the hydrogen detection space is crucial for industries utilizing hydrogen in their operations. Companies in sectors such as chemical manufacturing, food processing, and oil and gas must ensure their detection methods meet the applicable guidelines. This often involves rigorous testing and validation processes, ensuring that safety measures align with legal requirements.
Many organizations employ a mix of internal and third-party audits to confirm compliance. This helps in identifying any deficiencies that could pose risks. In situations where sensors fail the compliance tests, industries are then faced with the critical task of either upgrading their technologies or shifting to alternative methods of detection.
Furthermore, maintaining compliance can also provide a competitive edge. Companies that demonstrate adherence to regulatory standards can enhance their reputation, leading to increased customer trust and potentially greater market share.
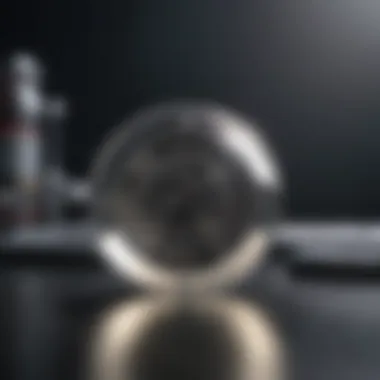
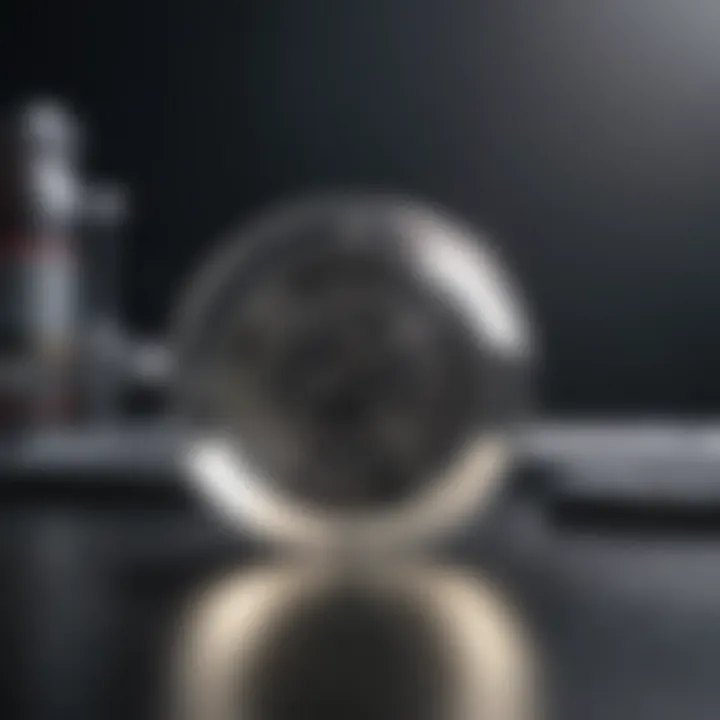
The path to regulatory compliance fosters a culture of awareness and proactivity in addressing safety concerns, which is fundamental to any organization that handles hydrogen gas.
As the landscape of hydrogen gas detection continues to evolve, so too will the associated regulations. Staying abreast of these developments ensures that industries remain ahead of the curve while prioritizing safety and reliability in their operations.
Case Studies
Case studies play a vital role in understanding the real-world implications of hydrogen detection methods. They offer insights into how theoretical principles translate into practical applications, enriching our comprehension of both the technology and its operational challenges. Through the lens of specific examples, we can draw valuable lessons about performance, efficiency, and safety protocols that might not be evident in a laboratory setting.
Engaging with case studies also showcases the broader societal impact of effective hydrogen detection. We can observe how various industries implement these technologies, ultimately affecting regulations, safety standards, and emergency response systems.
Hydrogen Detection in Industrial Settings
In industrial contexts, hydrogen detection is not just a choice; it’s often a matter of life and death. Industries like oil refining, chemical manufacturing, and food processing realize how pivotal accurate detection is. For instance, in chemical plants, hydrogen is frequently produced and utilized, posing both a risk and a necessity. Utilizing advanced sensor technologies helps in continuously monitoring hydroen levels ensures not only the safety of the workforce but also the integrity of the processes at hand.
- Real-Life Example: A prominent refinery recently installed a network of metal-oxide semiconductor sensors throughout the facility. This move was predicated on past incidents where undetected leaks resulted in catastrophic explosions. The proactive measure led to a significant reduction in hazard assessments and an increase in operational efficiency.
The case of a major automotive company is equally illustrative. They harnessed fiber-optic sensors in their hydrogen fuel cell vehicle assembly line to enhance detection sensitivity. Not only did this ensure worker safety, but it also streamlined quality control processes, demonstrating how effective hydrogen detection can intersect with productivity goals.
Research Laboratories and Safety Protocols
In research laboratories, strict adherence to safety protocols concerning hydrogen gas detection cannot be overstated. Scientists handle hydrogen in various forms for experimentation, making accurate detection crucial. A simple misstep can lead not only to loss of data but can also pose grave threats to personal safety.
- Example in Academia: A university research lab focused on experimenting with hydrogen fuel cells exemplified the importance of choice in detection systems. They implemented advanced optical sensors that provide real-time monitoring, which not only ensured student and faculty safety but also provided immediate feedback for ongoing experiments.
Effective protocols dictate not only installation procedures but also regular maintenance checks to calibrate and ensure consistent performance. Standard Operating Procedures (SOPs) in laboratories, such as regular training sessions on how to respond to false alarms, illustrate a comprehensive culture of safety.
Moreover, cross-collaboration between safety engineers and researchers enhances the detection capabilities while fostering communication within teams. The integration of new technologies, such as smart monitoring systems and data analytics, can vastly improve responsiveness to hydrogen gas events, as seen in a national lab that recently overhauled its detection systems.
Overall, studying these case studies encapsulates a wealth of understanding that informs future advancements in hydrogen detection. Each real-world instance reaffirms the necessity for continued innovation, thorough methods of detection, and the essential nature of safety practices across sectors.
Future Directions in Hydrogen Detection
As the world pivots towards sustainable energy, the quest for better detection methods for hydrogen gas is gaining traction. Technological advancements not only promise improved safety measures but also enhance efficiency in industries reliant on hydrogen. This section peeks into the future, spotlighting potential innovations and collaborations that could shape hydrogen detection.
Emerging Technologies
New technologies are on the horizon, which could radically change how hydrogen detection is approached. For instance, developments in nanotechnology are paving the way for sensors that are more sensitive and faster at detecting even trace amounts of hydrogen. These sensors often utilize nanostructured materials, which enhance their responsiveness due to increased surface area relative to volume. In addition, solid-state technologies are emerging, with potential applications in portable detection devices.
- Key technologies to look out for:
- Graphene-based sensors: Exhibiting exceptional conductivity and sensitivity, graphene could revolutionize hydrogen detection.
- MEMS (Micro-Electro-Mechanical Systems): These devices could offer compact and highly sensitive solutions for real-time monitoring.
Another frontier is the adaptation of AI and machine learning in detection systems. By analyzing patterns and data from multiple sources, these systems can potentially identify hydrogen leaks much more accurately. This integration can lend a hand in interpreting vast amounts of sensor data, eliminating false triggers while ensuring rapid response times.
Interdisciplinary Collaborations
No innovation exists in a vacuum, and the future of hydrogen detection will likely depend on robust interdisciplinary collaborations. Engineering, chemistry, environmental science, and data analytics must come together to push the boundaries of what’s achievable in this field.
- Potential avenues for collaboration:
- Academia and Industry Partnerships: Collaborative research between universities and private companies can accelerate the development of novel detection technologies, creating solutions that are both practical and pioneering.
- Cross-sector Initiatives: For instance, combining expertise from the automotive sector with those developing hydrogen infrastructure can yield benefits in both safety and efficiency.
Indeed, partnerships across different disciplines not only expand the scope of research but can also lead to shared resources, knowledge, and strategies that would be difficult to attain individually.
Interdisciplinary approaches can significantly amplify the potential for breakthrough technologies in hydrogen detection, benefiting both scientific inquiry and practical application.
The End
Hydrogen gas detection is an area of significant importance that intersects energy safety and environmental stewardship. This article has navigated through several critical aspects regarding the detection of hydrogen gas, underscoring how vital it is to implement effective methodologies grounded in sound scientific principles. The rising concern for safety in industrial settings, coupled with the need to harness hydrogen's potential as a clean energy source, emphasizes the gravity of developing reliable hydrogen detection systems.
Summary of Key Findings
Throughout the discussion, several key points have emerged:
- Diverse Detection Methods: Various detection methods, including electrochemical sensors, optical technologies, and thermal conductivity sensors, each bring distinct advantages and challenges to the table.
- Sensor Challenges: Sensitivity and selectivity issues often plague hydrogen sensors, where the presence of other gases can create false readings, necessitating continuous innovation in sensor technologies.
- Emerging Innovations: Recent advancements, particularly in nanotechnology and smart technology integration, showcase promising avenues for improving detection technologies, enhancing safety protocols across various industries.
- Compliance and Standards: The necessity for adherence to regulatory standards has been highlighted, as they guide industry practices to ensure worker safety and environmental protection.
"The path forward in hydrogen detection not only supports existing safety frameworks but also propels forward the possibilities for a cleaner energy future."
The Path Ahead for Hydrogen Gas Detection
The road ahead for hydrogen gas detection is laden with opportunities that blend scientific research with technological advancement. One notable area includes the integration of artificial intelligence into sensor technologies. Such innovations can enhance the precision of readings while reducing the potential for human error. Additionally, fostering interdisciplinary collaborations, particularly between chemists, engineers, and environmental scientists, can lead to groundbreaking techniques designed to tackle current detection hurdles efficiently.
Furthermore, embracing sustainable practices in sensor manufacturing and material choice will not only reduce the ecological footprint but also meet burgeoning regulatory demands. As the global push for clean energy sources intensifies, so too will the need for innovative approaches to hydrogen detection. The future lies in transforming theoretical practices into effective real-world applications, ensuring safety while propelling the hydrogen economy.
In summary, a focused approach toward resolving the challenges in hydrogen detection is paramount. It will not only mitigate risks associated with hydrogen use but also bolster the transition to hydrogen as a sustainable energy source in the years to come.
As we reflect on the discussions highlighted in this article, it serves as a call to action for scientists, policymakers, and industry leaders alike to prioritize advancements in this essential field.