The Genetic Origins of Sickle Cell Anemia Explained
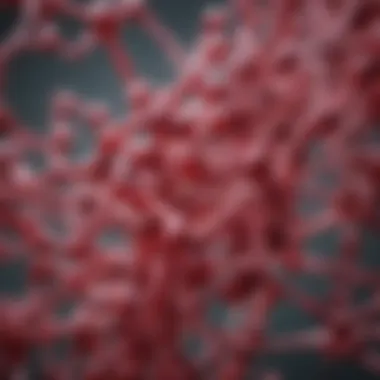
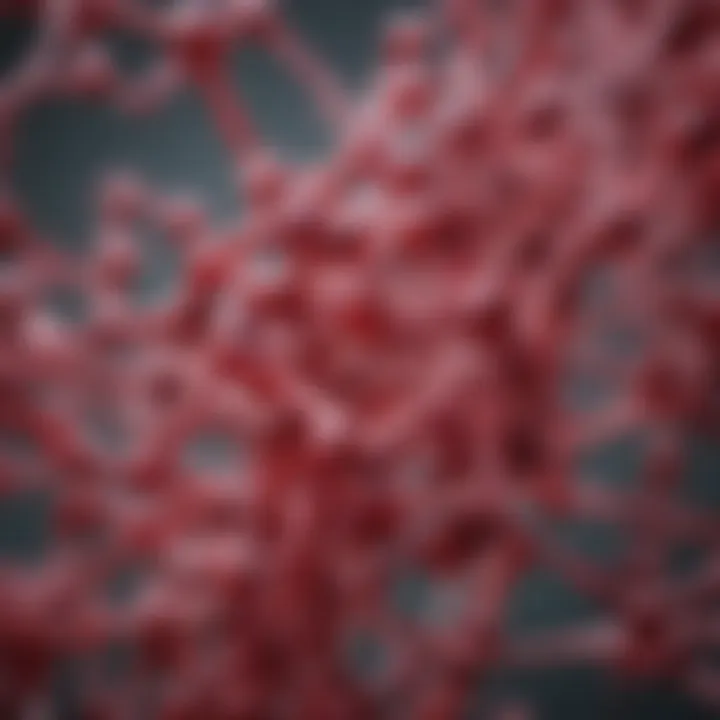
Intro
Sickle cell anemia is not merely a health condition; it's a complex intertwining of genetics and biology that shapes the experiences of millions worldwide. The deep-rooted nature of this disorder comes from a seemingly simple alteration in our DNA—the hemoglobin, the protein responsible for carrying oxygen in our blood, undergoes a transformation that leads to significant changes in the red blood cells. Instead of the typical disc shape, these cells become rigid and crescent-shaped, hence the name, sickle cell. This mutation doesn’t just impact individual health, it reverberates through families and communities, raising questions about genetics, inheritance, and the need for supportive measures like genetic counseling.
The mutation that causes sickle cell anemia occurs in the HBB gene, which provides instructions for making the beta-globin subunit of hemoglobin. A single nucleotide change leads to the production of an abnormal form of hemoglobin known as hemoglobin S (HbS), which drastically alters how red blood cells behave. The implications of this change—ranging from pain crises to increased risk of infections—are critical areas of study for both researchers and clinicians.
This article will explore in detail the genetic mutation behind sickle cell anemia, its inheritance patterns, and the critical role of genetic counseling. Understanding these aspects can guide patients and families as they navigate the complexities of this disease.
Prelude to Sickle Cell Anemia
Sickle cell anemia, a genetic disorder, holds a significant place in the annals of medicine and genetics. Understanding this condition goes beyond the clinical implications; it opens up discussions about the intricate relationship between genetics and health. This #article dives into the multifaceted nature of sickle cell anemia by exploring the mutation at its core, unraveling how a single nucleotide change can lead to such profound consequences in an individual's life.
At the heart of these explorations are a few compelling elements. First, there’s the importance of grasping the basic definitions and mechanisms of sickle cell anemia. Without this foundation, one might miss the broader implications that such genetic mutations have on human health and disease management.
Second, historical context matters. The journey of understanding sickle cell anemia isn’t just a scientific endeavor; it intertwines with social, cultural, and ethical narratives. When one reads about sickle cell disease, they encounter the evolution of research, the impact of historical injustices, and the ways in which societies have responded.
Moreover, sickle cell anemia serves as a case study in genetic inheritance. It highlights the principles of autosomal recessive patterns, thereby informing not just the affected individuals but also their families and future generations. This mutation offers profound insights into disease expression and variability, informing medical best practices and therapeutic approaches.
This topic isn't merely academic. It directly affects those living with the disorder and their loved ones. By investigating the genetic underpinnings of sickle cell anemia, this article will provide crucial takeaways for students, researchers, educators, and professionals alike, encouraging a deeper understanding of not just a disease, but the broader implications of genetic disorders in society.
"Sickle cell anemia is more than a textbook example; it's a living reality for many, rich with implications for genetics and health management."
In sum, understanding sickle cell anemia lays the groundwork for future discussions on treatment advancements, genetic counseling, and ongoing research, making this an urgent and vital topic worth exploring.
The Genetic Basis of Sickle Cell Anemia
Understanding the genetic foundations of sickle cell anemia is crucial for grasping how this disorder manifests and affects individuals. This section dives into the intricate world of DNA and genetic mutations, focusing on how these elements play a vital role in the condition's development and progression. By breaking down the complexities of genetic material and the mutations associated with sickle cell anemia, we can uncover insights that steer research, treatment, and advice for patients and families.
Understanding DNA and Genes
Structure of DNA
The structure of DNA holds significant importance in the realm of genetics. At its core, DNA, or deoxyribonucleic acid, is composed of two long strands forming a double helix, twisted like a spiral staircase. Each strand is made from a sequence of nucleotides, which include the bases adenine (A), thymine (T), cytosine (C), and guanine (G). The way these bases pair (A with T, and C with G) is pivotal.
One of the key characteristics of this structure is its stability. The double helix protects the genetic information it carries, which is especially beneficial in this article as we explore the mutation that leads to sickle cell anemia. This stable structure allows for accurate replication during cell division, ensuring that genetic material is passed down correctly.
Additionally, the unique feature of DNA being double-stranded affords it a remarkable resilience to mutations, at least under normal circumstances. However, when mutations do arise due to various factors, the implications can be significant, adding complexity to our understanding of conditions like sickle cell disease.
Function of Genes
Genes are segments of DNA that carry instructions for making proteins, essential for the structure and function of living organisms. Each gene's function is to code for specific proteins that play roles in everything from enzyme activity to cell structure.
A vital aspect of gene function is its regulation. Genes can be turned on or off, which determines when and how much protein is produced, impacting cellular processes significantly. This characteristic is particularly important in the context of sickle cell anemia.
The specific gene involved in sickle cell disease, the HBB gene, details how genetic instructions translate to hemoglobin production. The malfunctioning of this gene caused by mutation leads to the production of abnormal hemoglobin, which directly contributes to the symptoms of the disease. This focus on gene function enhances our understanding of the disease and its potential treatment options.
Types of Genetic Mutations
Point Mutations
A point mutation represents a change in a single nucleotide base within the DNA sequence. This slight alteration can have monumental impacts, especially in the context of sickle cell anemia. In fact, the condition specifically arises from a point mutation in the HBB gene, where adenine is swapped for thymine.
The principal characteristic of point mutations is their simplicity—a single change leads to diverse outcomes. This simplicity makes point mutations a popular subject of study, as they can lead to significant disease phenotypes despite their minor nature.
However, one unique feature of point mutations is that while many might be silent and have no impact, some can have profound effects, such as those leading to sickle cell anemia. Understanding these mutations aids in appreciating how a small change can alter the function of an entire protein.
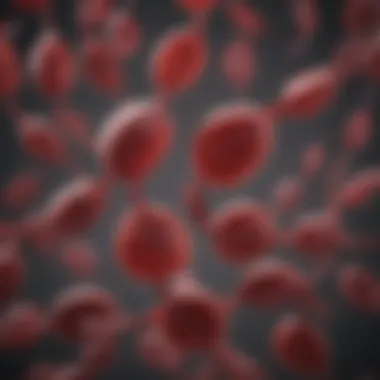
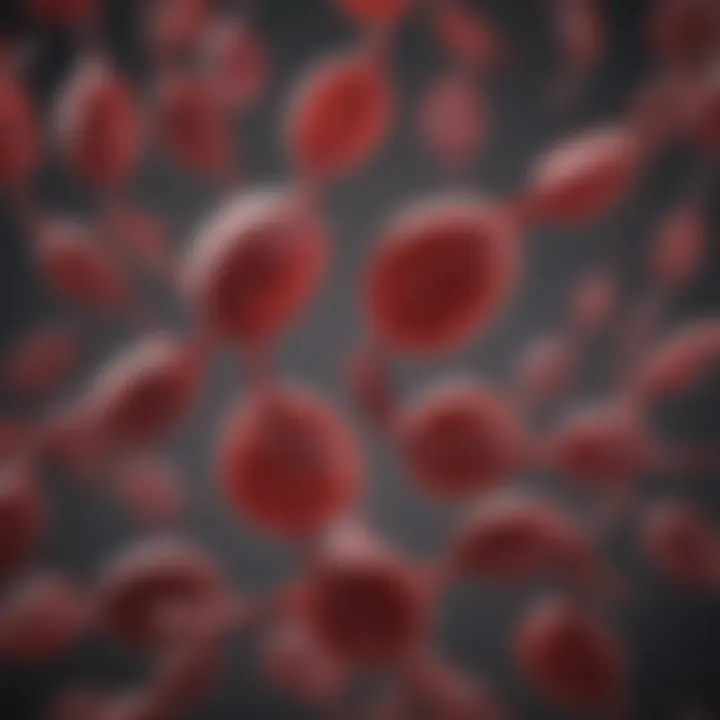
Insertions and Deletions
Insertions and deletions are genetic mutations where extra bases are added or removed from the DNA sequence. Unlike point mutations, these types of mutations can lead to frameshifts in the genetic code, producing downstream consequences on protein structure and function.
One key characteristic of insertions and deletions is their potential to cause more extensive disruptions than point mutations. This makes them a significant area of interest. They are not as straightforward as point mutations, often leading to nonfunctional proteins if not correctly managed by the cell.
One unique aspect of these mutations is their unpredictability; while they can have negative effects, some could potentially provide an adaptive advantage in certain environments. This ambiguity adds depth to the examination of genetic mutations and highlights the complexity inherent in understanding sickle cell anemia and its associated mutations.
Understanding the types of genetic changes enhances our capacity to develop targeted treatments and genetic counseling strategies, making the study of these mutations vital in the fight against sickle cell disease.
Sickle Cell Disease and Mutation Mechanism
Understanding sickle cell anemia begins with delving into the mutation mechanisms that drive this condition. The genetic foundation of sickle cell disease is pivotal, as it reveals how mild alterations at the molecular level can manifest in significant physiological consequences. What seems like just a small change in the DNA sequence can lead to a cascade of health issues, impacting the quality of life for millions. By studying these mechanisms, we can better appreciate how genetics interweave with biology to affect individuals.
In this section, we focus specifically on the HBB gene and the mutation that lies at the heart of sickle cell disease. Analyzing the mutation mechanism gives insights not only into the nature of the disease but also its implications regarding treatments and genetic counseling. This is essential for both researchers and anyone affected by the disease, as understanding can translate into action, helping manage symptoms more effectively.
The Role of the HBB Gene
The HBB gene, which encodes for the beta-globin subunit of hemoglobin, is crucial in the pathophysiology of sickle cell disease. Human hemoglobin is composed of two alpha and two beta chains, and any disruption in this balance may lead to altered hemoglobin function. In the case of sickle cell anemia, it is the mutations specifically located in the HBB gene that result in abnormal hemoglobin, dubbed hemoglobin S. An interesting aspect is that this substitution is not entirely detrimental—it also offers some protection against malaria, highlighting how a genetic defect can have mixed outcomes depending on environmental context.
Details of the Specific Mutation
Substitution of Adenine with Thymine
One key characteristic of sickle cell anemia is the single nucleotide polymorphism where adenine (A) is replaced by thymine (T) in the HBB gene. This substitution changes the codon from GAG to GTG, which ultimately alters the amino acid from glutamic acid to valine. Valine's hydrophobic nature causes the hemoglobin molecules to stick together under low oxygen conditions, causing the red blood cells to change shape into the characteristic sickle form.
This mutation is a striking example of how a single base change can lead to drastic phenotype alteration. Its role is not just limited to the disease aspect but also illustrates the broader implications of genetic variation in human evolution. Those who carry one copy of the mutated gene might enjoy advantageous traits, which can explain the persistence of such mutations in various populations.
Consequences on Hemoglobin Structure
The consequences of this mutation on hemoglobin structure are substantial. As a result of the substitution of amino acid, hemoglobin S tends to polymerize, especially when it is deoxygenated. This polymerization leads to the deformation of red blood cells, which can clog blood vessels and hinder circulation — a hallmark symptom of vaso-occlusive crises. Moreover, sickle-shaped cells have a shorter lifespan than normal red blood cells, contributing to chronic anemia.
This structural alteration is what separates sickle cell anemia from other forms of hemoglobin disorders. While individuals with normal hemoglobin maintain healthy, flexible red blood cells, those afflicted face a myriad of complications, including pain, infections, and organ damage. Understanding these consequences helps researchers develop targeted treatments and interventions, providing a map for future therapies aimed at managing this complex condition.
"A small step in nucleotide change, a giant leap into medical research implications."
In sum, exploring the genetic foundations behind sickle cell anemia not only casts light on the mechanics of the disease but also opens the door to further inquiries into how we might address it through innovative treatments and comprehensive genetic education.
Phenotypic Expression of Sickle Cell Anemia
The phenotypic expression of sickle cell anemia is crucial to understanding how genetic mutations translate to real-world implications. It serves as a pathway linking the microscopic changes in DNA to the macroscopic symptoms patients endure. Studying the phenotype unveils how a single genetic alteration can result in significantly different health outcomes, highlighting the interplay of genetics and environment.
Symptoms and Complications
Vaso-Occlusive Crises
Vaso-occlusive crises represent a hallmark symptom in sickle cell anemia and are characterized by the sudden blockage of blood vessels by sickled red blood cells. This occurrence leads to excruciating pain episodes and can affect various organs. The key characteristic of vaso-occlusive crises lies in their unpredictable nature; they can strike without warning, necessitating swift medical intervention.
The significance of focusing on vaso-occlusive crises lies in their profound impact on patients' lives. These pain episodes not only induce physical suffering but also hinder daily activities, leading to a reduced quality of life. Unique to vaso-occlusive crises is that they can lead to further complications, such as acute chest syndrome, which can be life-threatening.
The advantages of understanding this symptom in the context of sickle cell anemia lie in the potential for developing targeted management strategies. Recognizing triggers and educating patients on pain management may mitigate some of the crises' inevitable occurrences.
Anemia and Fatigue
Anemia is another prominent feature of sickle cell anemia, driven by the rapid breakdown of sickled red blood cells, which have a shorter lifespan compared to normal cells. This condition leads to chronic fatigue due to insufficient oxygen delivery throughout the body. The key characteristic of anemia in this context is its persistent nature; patients often describe feeling unremitting tiredness, impacting their cognitive and physical abilities.
Choosing to discuss anemia and fatigue is beneficial as it connects directly to patient care and lifestyle management. The unique aspect of this symptom is its subtlety; unlike acute pain crises, anemia can be insidious and may not prompt patients to seek immediate help. This makes it critical for caregivers and families to recognize fatigue as a significant symptom of sickle cell disease.
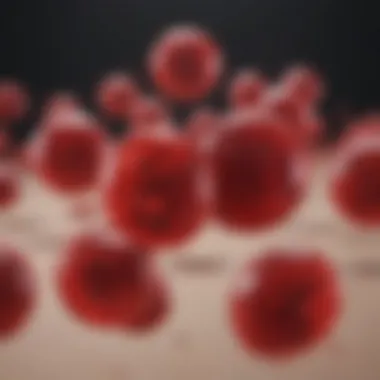
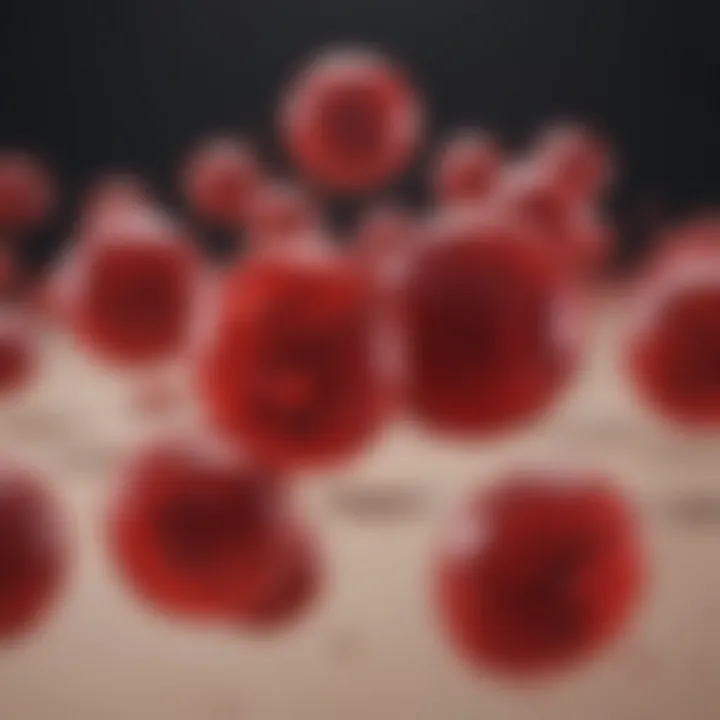
Addressing anemia within this article serves to underline the importance of blood transfusions and medications like hydroxyurea, which can assist in managing hemoglobin levels and improving energy levels, thus improving life quality.
Variability in Disease Severity
Genetic Factors
Genetic factors play a pivotal role in the phenotypic variability of sickle cell disease. This variability can be attributed to a range of minor mutations within the HBB gene that affect how hemoglobin is produced. The key aspect of genetic variability is the polymorphisms present which can influence the expression of the disease, potentially leading to milder or more severe manifestations.
The choice to emphasize genetic factors is beneficial as it gears towards creating personalized treatment plans. Understanding individual genetic profiles can help predict disease progression and tailor therapies accordingly. A unique element of studying these factors is the ongoing research into gene editing techniques that may one day rectify harmful mutations.
However, the disadvantages might emerge from the complexity of genetic interactions and the challenge in effectively communicating this to patients and families who may not have a scientific background.
Environmental Factors
Environmental factors equally contribute to the expression of sickle cell anemia. Elements such as climate, stress levels, and access to comprehensive healthcare can greatly influence the severity of symptoms. One notable characteristic of these factors is their dynamic nature; they can vary significantly from one individual to another, thus affecting overall health outcomes.
Focusing on environmental factors is a robust choice for this article as it shines a light on the lifestyle changes that can improve quality of life. For instance, staying hydrated and avoiding extreme temperatures can alleviate many symptoms. A unique aspect of considering these factors is the involvement of social determinants of health; by addressing these determinants, health care providers can employ holistic management strategies.
The drawback, however, resides in the variability of environmental circumstances that may not be universally applicable to all patients, underscoring the need for tailored advice based on personal and local conditions.
"The study of phenotypic expression in sickle cell anemia illuminates how deeply intertwined genetics and environment are, shaping the lives of individuals affected by this condition."
With these insights into the symptoms, complications, and variability in disease expression in sickle cell anemia, the understanding builds toward a more holistic picture necessary for advancing both treatment and patient care.
Inheritance Patterns of Sickle Cell Anemia
Understanding the inheritance patterns of sickle cell anemia is crucial for grasping how this condition is passed down through generations. Sickle cell anemia, which stems from a specific genetic mutation in the HBB gene, follows an autosomal recessive inheritance pattern. This means that an individual must inherit two copies of the mutated gene—one from each parent—to develop the disease. In contrast, individuals who inherit only one copy become carriers, often without symptoms themselves but can pass the gene to their offspring.
This topic has broad implications for genetic counseling, screening, and public health initiatives. Recognizing the inheritance pattern allows families to understand risks and provides necessary information for informed decisions regarding family planning. It also sheds light on the potential need for interventions or treatments that could ease the impact of sickle cell anemia.
Autosomal Recessive Inheritance
In the case of autosomal recessive inheritance, both parents must carry at least one copy of the mutated HBB gene for their children to be affected. When both parents are carriers, there is a 25% chance with each pregnancy that their child will inherit two copies of the mutation, leading to sickle cell disease. This also means there’s a 50% chance that the child will be a carrier like the parents, and a 25% chance that the child will not inherit the mutation at all.
Families impacted by sickle cell anemia can often trace their genetic lineage to see a pattern of carriers and affected individuals, helping them understand their own circumstances better. Knowing whether family members are carriers can inform future health decisions, knowing if testing might be necessary for future offspring.
Genetic Counseling and Testing
Genetic counseling offers families the support they need to navigate the complexities of genetic mutations and their implications. Health professionals can explain the autosomal recessive pattern of inheritance, helping individuals comprehend the specific risks involved.
Importance of Carrier Testing
Carrier testing is a pivotal service in genetic counseling for sickle cell anemia. This testing identifies individuals who carry one copy of the mutated gene. Knowing carrier status enables families to assess the risk for their children and choose options that may affect future health outcomes.
A key characteristic of carrier testing is that it is a fairly straightforward process, often involving a blood sample or cheek swab. This gives it a practical edge, making it a valuable choice for many families.
One unique feature of carrier testing is its applicability across different populations. As sickle cell anemia is more prevalent in certain ethnic groups—such as those of African, Mediterranean, or Middle Eastern descent—this type of testing serves as a beneficial screening tool. However, many people may not realize their carrier status until conducting a test, highlighting a challenge—proactive approaches to testing could provide more benefits.
Implications for Family Planning
The implications for family planning stemming from genetic counseling and carrier testing are significant. Understanding genetic backgrounds aids parents in making informed choices about having children. For instance, couples who are both carriers of the sickle cell trait might consider prenatal testing to prepare for any potential health challenges their baby may face.
Family planning discussions often take into account strategies such as in-vitro fertilization with genetic screening or the option of donor gametes, which can bypass the genetic risk altogether. This aspect is critical as it provides a pathway for parents concerned about passing on the disorder.
However, family planning discussions are not without their complexities. Emotional factors, cultural beliefs, and personal circumstances can influence decisions. It's essential to approach these discussions with sensitivity and a thorough understanding of the family's unique situation.
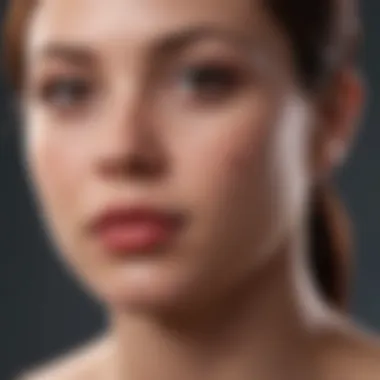
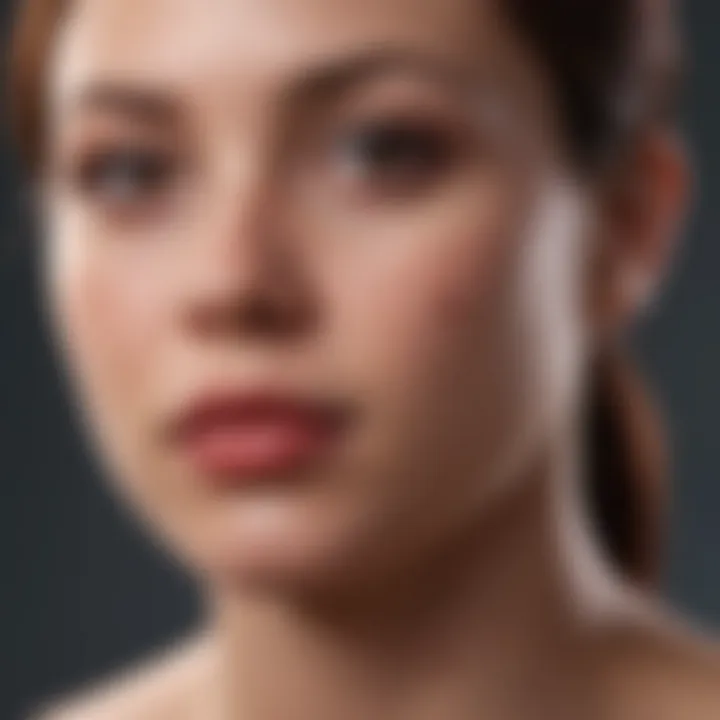
Advancements in Research and Treatment
Advancements in research and treatment of sickle cell anemia represent a beacon of hope, offering innovative strategies for better management and potential cures for a disease that has long burdened countless individuals and families. This area of study garners significant attention due to the complex genetic underpinnings of the condition and its profound impact on the quality of life for those affected. The research not only enhances our understanding but also drives the development of effective therapies that could alter the course of the disease.
Gene Therapy Approaches
CRISPR and Its Applications
CRISPR technology has emerged as a revolutionary tool in genetic research, particularly concerning sickle cell anemia. At its core, CRISPR enables targeted editing of specific DNA sequences, allowing for precise modifications that can potentially correct mutations like those responsible for sickle cell disease. One key characteristic of CRISPR is its relative ease of use compared to earlier methods of gene editing, making it a popular choice for researchers.
The unique advantage of CRISPR lies in its efficiency; it can simultaneously target multiple genes simultaneously, a feature that could be invaluable in addressing the complexities of sickle cell pathology. However, this technology comes with disadvantages, particularly concerns surrounding off-target effects, where unintended genetic alterations could occur. Therefore, while CRISPR holds immense promise, careful consideration and rigorous testing are essential before wide-scale application in clinical settings.
Challenges and Future Directions
Even as CRISPR shows great potential, there are notable challenges that need addressing. The complexity of the human genome, variations in patient responses, and ethical considerations all present hurdles in advancing gene therapy approaches. One of the key characteristics of these challenges is the regulatory landscape. Navigating this terrain is crucial for the safe deployment of new therapies.
A unique feature of ongoing research in this area is the movement toward personalized medicine—tailoring therapies to fit individual genetic profiles. While this offers potential benefits, it also adds layers of complexity to treatment protocols. As scientists work to refine these approaches, it is pivotal to maintain a balance between innovation and safety, ensuring that new methods do not lead to unforeseen complications.
Management Strategies
Hydroxyurea Treatment
Hydroxyurea is one of the well-established treatments for sickle cell anemia. It offers a means to reduce the frequency of painful crises and the need for blood transfusions by increasing fetal hemoglobin levels. A significant aspect of hydroxyurea treatment is its ability to re-promote the production of healthy hemoglobin, thus alleviating some symptoms of the disease. Its accessibility and relative safety make it a popular choice among healthcare providers.
However, this treatment also comes with its own set of challenges, including potential side effects and the need for constant monitoring. While hydroxyurea can offer substantial benefits, optimal responses can vary among individuals, leading to a nuanced approach in managing therapy for different patients.
Supportive Care Approaches
Supportive care is an essential component in the management of sickle cell anemia, focusing on pain relief and the prevention of complications. This approach incorporates a variety of strategies, including hydration, pain management techniques, and vaccinations to prevent infections. A major characteristic of supportive care is its holistic nature, addressing both physical and emotional challenges faced by patients.
The unique aspect of supportive care is its adaptability; care plans can be tailored to each patient's needs, emphasizing patient-centered strategies. However, while supportive care enhances the quality of life, it does not directly impact the underlying genetic causes. Thus, ongoing research into both treatment options and supportive strategies is critical for comprehensive management of sickle cell anemia.
"The advances in treatment and research for sickle cell anemia not only signify hope for patients but also a testament to the incredible potential of science to combat genetic disorders."
Overall, the advancements in research and treatment for sickle cell anemia represent a critical frontier in the fight against this condition, illuminating a path that melds scientific progress with patient-centric care.
Ending and Future Perspectives
Understanding sickle cell anemia through the lens of its genetic foundations provides a solid framework for grasping the complexities of this condition. The key elements discussed throughout this article underscore not only the significance of recognizing the specific mutation that leads to sickle cell disease, but also the social, personal, and health implications entwined with it. By comprehending the complete picture—from genetic underpinnings to phenotypic expressions—one can appreciate the holistic approach required in managing sickle cell anemia.
The connections made between genetics and treatment options reveal the potential for more targeted therapies that could significantly improve the quality of life for those affected. Furthermore, broadening the research scope in this area is pivotal. As our understanding deepens, so does the possibility of enhancing existing treatments and possibly developing new therapies that could make a real difference.
Summary of Key Points
- Sickle cell anemia is primarily caused by a mutation in the HBB gene, resulting in abnormal hemoglobin.
- The disease displays variability in severity, influenced by both genetic and environmental factors.
- Genetic counseling plays an essential role in guiding families about the risks of inheritance and available testing options.
- Recent advancements in gene therapy, such as CRISPR, show promise in correcting genetic mutations leading to sickle cell anemia.
The Future of Sickle Cell Anemia Research
Emerging Technologies
Emerging technologies enlighten the horizon for sickle cell anemia research, offering tools that could revolutionize our understanding and treatment of genetic disorders. One standout technology is CRISPR, which allows for precise gene editing to correct mutations directly. This is a game-changer, as it opens the door to potentially curing the condition rather than just managing its symptoms. The key characteristic of CRISPR is its high accuracy and efficiency. This makes it a favored option, as researchers can perform targeted edits without the risk of affecting other genes.
However, CRISPR is not without challenges. Although the promise it holds is bright, ethical considerations and potential off-target effects remain hurdles needing addressing. Its unique feature lies in the ability to not only modify genes but also to study their functions in real-time, thus leading to advances in personalized medicine.
Potential for Innovative Therapies
Innovative therapies pave the way for a new chapter in the management of sickle cell anemia. Current medications, like hydroxyurea, are already helping patients lead a better life, but there’s so much potential for development. One of the most significant aspects of potential innovative therapies is the use of stem cell transplants, which can offer a curative option for a select group of patients.
These therapies are beneficial as they utilize the body’s own cells to fight the disease which can lead to fewer side effects. A unique feature of these therapies is their adaptability; they can be tailored to individual patients' needs, drawing from the principles of personalized medicine.
However, barriers such as cost, accessibility, and the complexity of procedures can limit availability. Thus, while the potential is vast, deliberate efforts will be necessary to ensure that breakthroughs translate to effective and equitable healthcare solutions for those affected by sickle cell anemia.
As we stand on the verge of new discoveries, the future of sickle cell anemia research remains full of possibilities, promising not just advancements in treatment but also improved lives for many.