Function of Mitochondria: The Cell's Powerhouses
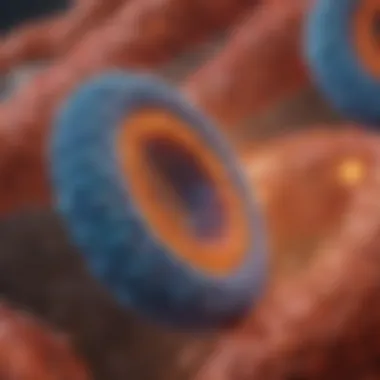
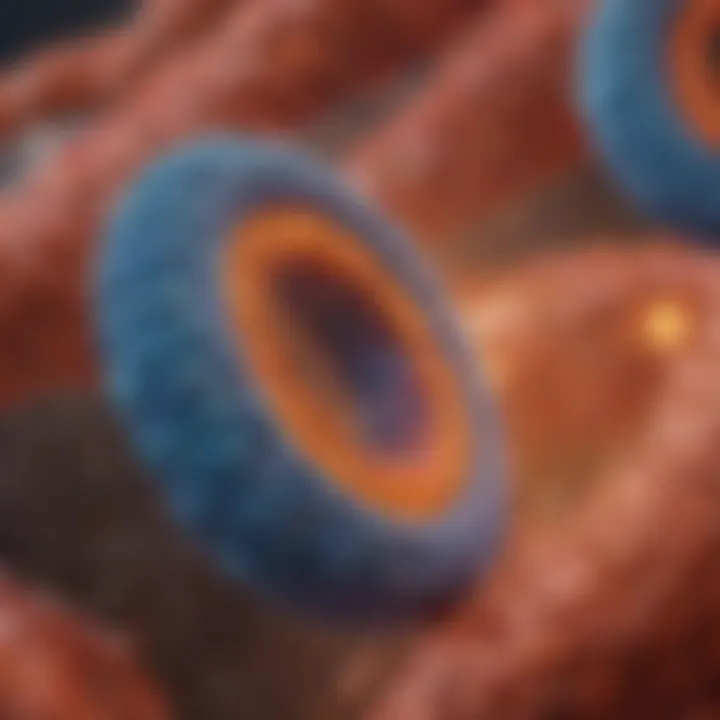
Intro
Mitochondria are often referred to as the powerhouses of the cell, signifying their critical role in energy production. They are double-membraned organelles found in nearly all eukaryotic cells. Their principal function is to convert organic compounds into adenosine triphosphate (ATP), which cells utilize for various metabolic processes. Understanding the intricacies of mitochondrial function is indispensable in cell biology, particularly when considering their implications in health and disease. This article will explore the multifaceted roles of mitochondria beyond energy production, including their involvement in cellular signaling, apoptosis, and metabolism. It aims to provide a detailed examination of mitochondrial DNA and its impact on cellular function, as well as the consequences of mitochondrial dysfunction in various diseases.
Methodology
Overview of research methods used
The information presented in this article is gathered from a comprehensive review of existing literature on mitochondrial function. Studies included peer-reviewed journals, articles from reputable sources such as Britannica, and current research findings highlighted in medical and scientific platforms.
Research methodologies varied, but primary focus was on experimental biology, biochemistry, and genetics. Meta-analyses provided insights into existing knowledge gaps and the complexities of mitochondrial roles in the cell.
Data collection techniques
Data was collected through various techniques:
- Experimental Studies: These involved laboratory-based experiments focusing on mitochondrial bioenergetics, dynamics, and genetics.
- Clinical Research: Analysis of patient data linked to mitochondrial diseases has shown patterns that help understand dysfunction and associated syndromes.
- Surveys and Meta-analysis: Gathering data from multiple studies provides a consolidated view of current knowledge, allowing for better understanding of topics previously studied in isolation.
Future Directions
Upcoming trends in research
Current research trends indicate a growing interest in mitochondrial medicine. Promising areas include gene therapy and targeted mitochondrial interventions. These approaches aim to address mitochondrial dysfunction linked to various conditions, such as neurodegenerative diseases and metabolic syndromes.
Areas requiring further investigation
Despite advances in our understanding, several areas warrant further research. Some of these include:
- The interaction between mitochondria and other organelles.
- Mechanisms underlying mitochondrial biogenesis and degradation.
- The influence of mitochondrial DNA variability on health.
- Mitochondrial roles in aging and longevity.
Understanding mitochondria is not only crucial for comprehending energy production but also for the wider implications on cellular health, disease prevention, and potential therapeutic strategies.
Prologue to Mitochondria
Mitochondria are critical organelles that serve as the powerhouse of eukaryotic cells. Their primary function revolves around energy production through a complex series of biochemical processes. Understanding mitochondria is essential not just for cellular biology but also for grasping their implications in health and disease. They are involved in not just generating ATP, the energy currency of the cell, but also in critical processes like metabolism, signaling, and apoptosis.
Historical Perspective
The study of mitochondria dates back to the late 19th century. Their discovery contributed substantially to our understanding of cellular structures and functions. Early researchers like Richard Altmann were pivotal, as they termed these large organelles as 'bioblasts', hinting at their role in life and metabolism. Over decades, advancements in microscopy allowed scientists to observe their unique structure and draw conclusions about their function.
Mitochondrial Discovery
The formal identification of mitochondria occurred in the 1940s. Mitochondria were identified as distinct organelles within cells. This provided a foundation for understanding their specific roles in energy metabolism. Their discovery was crucial as it revealed the importance of these organelles in cellular respiration and energy production, leading to a broader exploration of bioenergetics.
Mitochondrial Structure
Mitochondria are highly structured organelles, consisting of multiple membranes that define their internal compartments. This regular structure is vital for their function, particularly in energy production.
Outer Membrane
The outer membrane serves as the first barrier surrounding mitochondria. It is distinctive for its smooth surface and contains porins, which are proteins allowing the passage of ions and small molecules. This characteristic makes it permeable, allowing substances to flow in and out freely, essential for the organelle’s metabolic activities.
Inner Membrane
The inner membrane is highly folded into structures called cristae. This folding increases the surface area available for biochemical reactions. The inner membrane plays a fundamental role in oxidative phosphorylation, where the production of ATP occurs. Its unique property lies in being impermeable to most ions, thus maintaining a distinct environment necessary for energy production.
Intermembrane Space
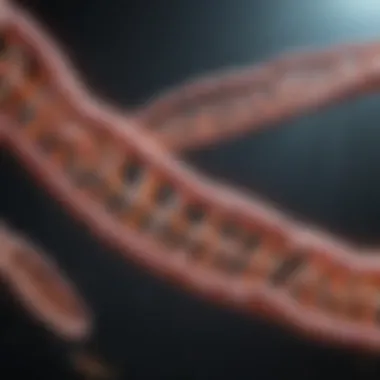
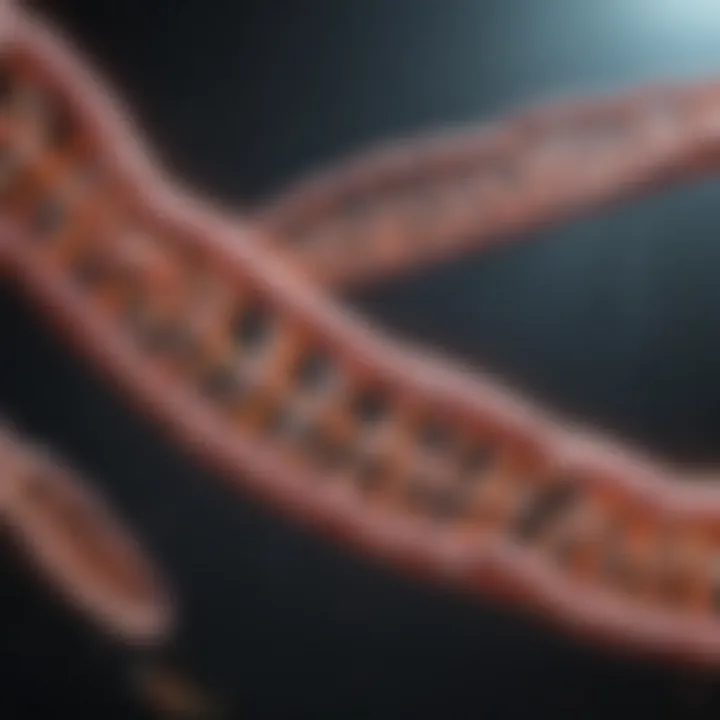
The space between the outer and inner membranes is called the intermembrane space. This compartment plays a crucial role in the electrochemical gradient formation that is vital for ATP synthesis. The intermembrane space accumulates protons during electron transport, contributing significantly to the proton motive force used in ATP generation.
Mitochondrial Matrix
The mitochondrial matrix is the innermost compartment of the organelle, encased by the inner membrane. It contains enzymes essential for the Krebs cycle and a variety of mitochondrial DNA. This unique feature of having its own DNA allows mitochondria to replicate independently of the cell’s nuclear DNA. The matrix is central to many metabolic pathways, making it fundamental to mitochondrial function.
Energy Production
Energy production is a fundamental function of mitochondria, marking them as essential players in cellular biology. These organelles are vital for converting nutrients into adenosine triphosphate (ATP), the energy currency of the cell. Understanding the mechanisms governing energy production is crucial for comprehending how cells thrive and respond to various metabolic demands.
Role of Oxidative Phosphorylation
Oxidative phosphorylation is the primary pathway through which ATP is generated in mitochondria. This complex process occurs within the inner mitochondrial membrane, where electron transport chains transfer electrons from NADH and FAD, derived from the breakdown of nutrients. The passage of electrons through these chains leads to the pumping of protons into the intermembrane space, creating a gradient. This gradient is vital for the subsequent synthesis of ATP.
Mitochondria function as more than just energy-generating units; they also balance redox processes and contribute to overall cellular homeostasis. The efficiency of oxidative phosphorylation can greatly influence a cell’s metabolic flexibility, impacting processes like growth, survival, and apoptosis.
Chemiosmosis and ATP Synthesis
Chemiosmosis is the mechanism by which the energy stored in the proton gradient is harnessed to produce ATP. The enzyme ATP synthase facilitates this process. Protons flowing back into the mitochondrial matrix drive ATP synthesis. This refinement of energy production is a critical link between electron transport and ATP generation. An imbalance in this system can lead to decreased ATP levels, affecting various cellular functions.
ATP synthesized through chemiosmosis is not just vital for energy. It also plays a role in signaling pathways and metabolic processes throughout the cell. Therefore, understanding how chemiosmosis occurs sheds light on broader implications for cellular health and efficiency.
Substrate-Level Phosphorylation
Substrate-level phosphorylation occurs in the mitochondrial matrix and is an alternative method of generating ATP. This process involves the direct transfer of a phosphate group from a phosphorylated substrate to ADP, forming ATP. Although it produces significantly less ATP than oxidative phosphorylation, it remains important, particularly during anaerobic conditions or in specific cell types that require rapid ATP synthesis.
In summary, energy production through oxidative phosphorylation, chemiosmosis, and substrate-level phosphorylation is integral to mitochondrial function. Each aspect of this process plays a crucial role in maintaining cellular energy levels and overall metabolic health. A comprehensive understanding of these mechanisms paves the way for further exploration into mitochondrial diseases and potential therapeutic interventions.
"Mitochondria are not just energy factories; they are critical for maintaining cellular signaling and metabolism."
For a deeper understanding, consider exploring additional resources on mitochondrial biology at Wikipedia or Britannica.
This exploration into mitochondrial energy production invites further inquiry into how these dynamics relate to health and disease.
Mitochondrial DNA
Mitochondrial DNA, often abbreviated as mtDNA, represents a critical component of cellular function and inheritance. This unique genetic material provides vital insight into mitochondrial biology. Understanding mtDNA is essential, as it plays multifaceted roles in energy metabolism and cellular signaling. The examination of mtDNA can also shed light on evolutionary biology and human diseases, linking genetic variations to specific health conditions. As research progresses, the importance of mtDNA continues to evolve, suggesting potential therapeutic targets and interventions.
Characteristics of mtDNA
Mitochondrial DNA differentiates itself from nuclear DNA in several ways. Firstly, mtDNA is circular and much smaller than nuclear DNA, typically encompassing around 16,500 base pairs in humans. This compact structure allows for efficient replication and regulation. Secondly, mtDNA is inherited exclusively from the mother, leading to maternal lineage tracking in genetic studies. Additionally, the high mutation rate of mtDNA contributes to its role in evolutionary studies, influencing population genetics.
The mitochondrial genome encodes for 37 genes essential for mitochondrial function. This gene set includes 13 proteins critical for oxidative phosphorylation, along with 22 tRNA and 2 rRNA genes that are integral for mitochondrial protein synthesis. A significant characteristic of mtDNA is its lack of introns, which streamlines the genetic information.
Inheritance Patterns
Mitochondrial inheritance operates quite differently from that of nuclear genes. Since mtDNA is passed down maternally, sons do not transmit it to their offspring. This unique pattern leads to intriguing implications for genetic studies. For example, mutations in mtDNA can reveal information about ancestral lineages and migration patterns of populations. Moreover, this inheritance model can exacerbate the understanding of specific diseases that follow maternal patterns, such as Leber's Hereditary Optic Neuropathy.
In practice, examining mitochondrial inheritance patterns can help identify carriers of mitochondrial diseases. Genetic testing may provide individuals and families with essential information for health management and family planning decisions.
Mutations and Their Impact
Mutations in mtDNA can have profound implications on human health. The accumulation of specific mutations can disrupt the normal functioning of mitochondria. This dysfunction is central to a range of disorders, particularly those affecting high-energy demanding tissues like the muscles and brain. Conditions such as mitochondrial myopathy and neurodegenerative diseases, including Parkinson's, can arise from mtDNA mutations.
Moreover, not all mutations are detrimental. Some variations may confer certain advantages, potentially influencing individual adaptability to environmental factors. However, the delicate balance of maintaining genetic integrity is crucial. Insights into mtDNA mutations provide a clearer picture of disease mechanisms and may guide future research in gene therapy and personalized medicine.
"Mitochondrial DNA serves not just as an energy generator but as a cornerstone of human genetic heritage, influencing both evolution and personal health."
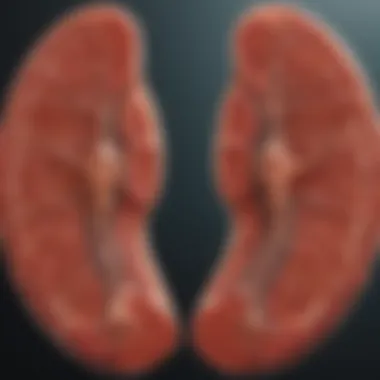
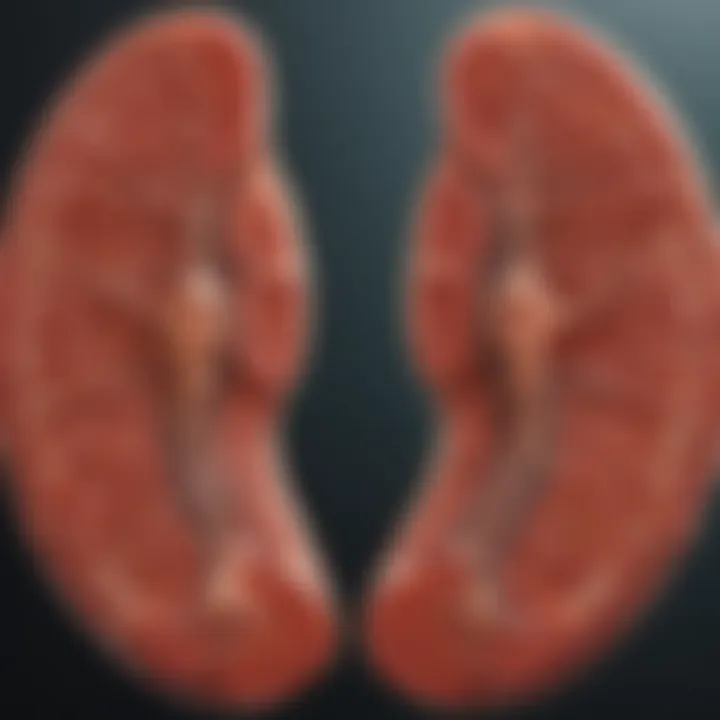
In summary, mitochondrial DNA is a powerful player in the cellular landscape. Its unique characteristics, distinct inheritance patterns, and the impact of mutations offer rich avenues for research and understanding. The continued exploration of mtDNA will likely reveal even more connections to health and disease, emphasizing its significance in both biological and medical contexts.
Mitochondrial Function Beyond Energy
Mitochondria are not merely the powerhouses of the cell; their roles extend significantly into various cellular functions that are essential for overall cellular health and integrity. Beyond their central function in energy metabolism, mitochondria contribute to the regulation of metabolic processes, cell death, and cell signaling pathways. Understanding these functions is crucial for appreciating the full impact of mitochondrial health on both normal physiology and disease.
Regulation of Metabolism
Mitochondria play a critical role in regulating metabolism through several pathways. They are central to the metabolism of carbohydrates, lipids, and proteins, converting these substrates into usable forms of energy. Mitochondria manage the TCA cycle, also known as the citric acid cycle, which generates NADH and FAD, key molecules that feed into the electron transport chain. This integration of metabolic pathways allows cells to respond dynamically to energy demands.
Moreover, mitochondria also regulate the balance between catabolism and anabolism. They do this by producing various intermediate metabolites that participate in biosynthetic pathways. By doing so, mitochondria influence not just energy production but also the synthesis of important biomolecules, highlighting their dual role in energy and metabolism regulation.
Role in Apoptosis
Apoptosis, or programmed cell death, is another crucial function of mitochondria. When cells encounter damage or stress, the mitochondria can release cytochrome c, which initiates a cascade of events leading to apoptosis. This process is vital for maintaining homeostasis and eliminating damaged cells that could turn cancerous.
The involvement of mitochondria in apoptosis underscores their importance in the overall health of an organism. Abnormalities in apoptotic signaling can lead to various diseases, including cancer and neurodegenerative disorders. Proper functioning of mitochondrial pathways is therefore essential in regulating cell survival and death.
Mitochondrial Signaling Pathways
Mitochondria are also critical to various signaling pathways, impacting cellular responses to different stimuli. Their ability to interact with other cellular components allows them to act as signaling hubs.
Calcium Signaling
Calcium signaling mediated by mitochondria is a significant aspect of intracellular communication. Mitochondria are capable of taking up calcium ions, which can modulate various cellular processes, including metabolism and cell proliferation. A key characteristic of calcium signaling is its role in influencing ATP production; when mitochondria take up calcium, they stimulate the activity of dehydrogenase enzymes in the TCA cycle, enhancing energy production.
This unique feature of calcium signaling is beneficial as it connects energy metabolism with cellular signaling, thereby coordinating the cell's energetic state with its functional requirements. However, excessive calcium uptake can lead to cell stress and death, making regulatory mechanisms essential.
Reactive Oxygen Species (ROS)
Reactive oxygen species (ROS) generation is another critical aspect of mitochondrial function. While ROS are often associated with cellular damage, they also act as important signaling molecules. Mitochondria produce ROS during the process of oxidative phosphorylation, and when in controlled amounts, they help in regulating various cellular processes, including metabolism and apoptosis.
The balance of ROS is crucial. Low to moderate levels can reinforce signaling pathways that promote survival, while high levels may lead to oxidative stress, damaging cellular components such as DNA and proteins. Understanding the dual role of ROS in cellular processes supports the idea that mitochondria, in their function as signaling entities, play a pivotal role in maintaining a balance within the cell.
Mitochondrial Dysfunction and Disease
Mitochondrial dysfunction is a critical aspect of cellular biology that has far-reaching implications for health and disease. Mitochondria, as energy producers, are crucial for the proper functioning of cells. When these organelles do not function correctly, the consequences can be severe, leading to a multitude of diseases and disorders. Understanding the link between mitochondrial dysfunction and various health conditions not only sheds light on the mechanisms of these diseases but also opens up potential avenues for treatment and management.
Neurological Disorders
Neurological disorders are closely connected to mitochondrial dysfunction. The brain is highly energy-dependent, relying on mitochondria to meet its energy demands. Deficiencies in mitochondrial function can lead to insufficient energy supply, resulting in neuronal damage or death. Conditions such as Alzheimer's disease, Parkinson's disease, and Huntington's disease have been linked to mitochondrial impairment. Evidence suggests that abnormalities in mitochondrial DNA and defective oxidative phosphorylation contribute to the pathogenesis of these disorders. This connection highlights the importance of targeting mitochondrial dysfunction in developing therapies for neurological diseases.
Metabolic Syndromes
Metabolic syndromes are another area where mitochondrial dysfunction has significant consequences. The mitochondria are central to metabolic processes, influencing pathways such as fatty acid oxidation and glucose metabolism. When mitochondrial efficiency declines, it leads to an imbalance in energy homeostasis. Conditions like obesity and type 2 diabetes exhibit such mitochondrial inefficiencies. Research indicates that targeting mitochondrial biogenesis and improving mitochondrial function may help mitigate metabolic disorders. Addressing these dysfunctions can enhance overall metabolic health and combat the increasing prevalence of these syndromes in society today.
Cardiovascular Implications
The cardiovascular system also bears the burden of mitochondrial dysfunction. Mitochondria are essential for heart muscle cells, providing the necessary energy to maintain normal heart function. Dysfunction in these organelles can lead to a range of cardiovascular diseases, including heart failure and ischemic heart disease. Moreover, mitochondrial dysfunction can contribute to increased oxidative stress and inflammation within the cardiovascular system, further exacerbating disease processes. Understanding how to prevent or reverse mitochondrial dysfunction could be key in developing effective treatments for heart disease and enhancing cardiovascular health.
Mitochondria in Cell Aging
Mitochondria are critical not just for energy production, but also for understanding the aging process at a cellular level. Their dysfunction has been increasingly linked to age-related decline in cellular functions. As cells age, the efficiency of mitochondria tends to decrease. This decline can have wider implications for overall health, contributing to various diseases and accelerated aging.
Theories of Aging
Several theories that try to explain aging often include mitochondrial dysfunction as a key factor. One prominent theory is the free radical theory, which suggests that byproducts of cellular respiration, particularly reactive oxygen species, damage cellular components over time. This damage accumulates and eventually leads to cell death. Another theory, the mitochondrial theory of aging, posits that as mitochondria become less efficient, their ability to provide energy decreases, resulting in reduced cellular function and survival. Thus, investigating these theories helps scientists understand how mitochondrial health relates to the aging process.
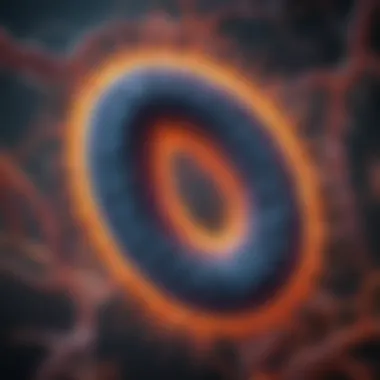
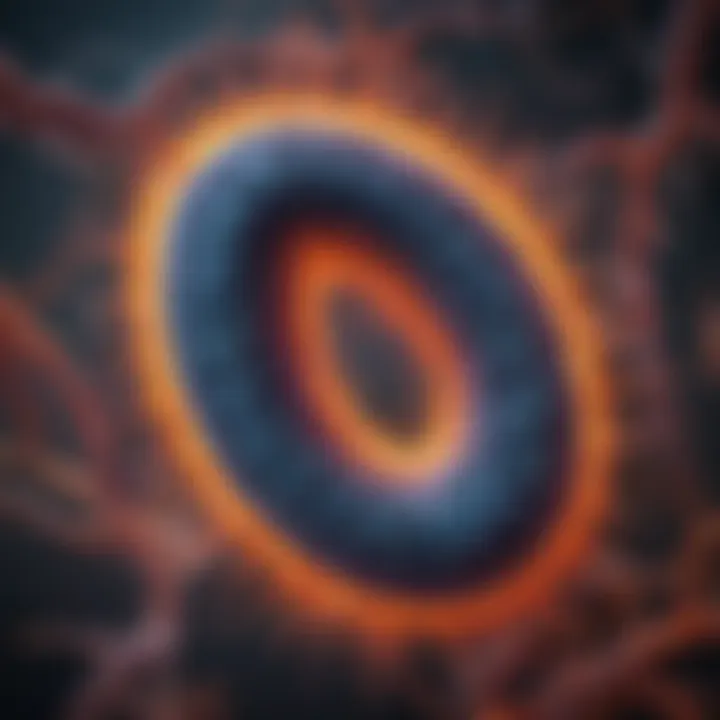
Mitochondrial Biogenesis
Mitochondrial biogenesis refers to the process by which cells increase their individual mitochondrial mass and activity. This process can be stimulated by various factors including exercise and proper nutrition. The regulatory proteins involved in mitochondrial biogenesis, such as PGC-1 alpha, play an essential role in maintaining mitochondria's health and functionality. Enhancing mitochondrial biogenesis is promising for improving metabolic health and possibly delaying aging. By understanding mitochondrial biogenesis, researchers can explore potential therapeutic strategies to counteract age-associated decline.
Impact of Lifestyle on Mitochondria
Lifestyle choices significantly affect mitochondrial function and health. Two main lifestyle factors are exercise and nutrition, each playing a distinct role in optimizing mitochondrial performance.
Exercise
Engaging in regular physical activity is key to promoting mitochondrial health. Exercise enhances the amount of mitochondria in cells, a process known as mitochondrial biogenesis. High-intensity interval training (HIIT) is particularly effective. It stimulates the production of more mitochondria, providing cells with greater energy reserves. The beneficial aspect of exercise is not only its immediate impact on mitochondrial performance but also its long-term benefits in enhancing endurance and metabolic rates. Therefore, exercise is essential for keeping mitochondria functioning well, especially as one ages.
Nutrition
Nutrition also plays a pivotal role in maintaining mitochondrial health. A diet rich in antioxidants can help to minimize oxidative stress, which is harmful to mitochondria. Nutrients such as omega-3 fatty acids, B vitamins, and Coenzyme Q10 are particularly beneficial for mitochondrial function. Additionally, a balanced diet supports optimal metabolic processes, ensuring a steady supply of energy. However, care should be taken because excessive caloric intake can lead to mitochondrial dysfunction. Thus, understanding the right nutrition is vital for sustaining mitochondrial health and thus promoting healthy aging.
Mitochondria not only produce energy but also play a crucial role in determining the cellular aging process. Monitoring and enhancing their health can significantly influence overall vitality as one ages.
Future Research Directions
Future research on mitochondria holds great potential to broaden our understanding of cellular function and related diseases. As research progresses, several areas emerge as significant for exploration. These areas include gene therapy approaches, strategies for targeting mitochondrial dysfunction, and the value of interdisciplinary studies. Each of these pathways offers a different angle to understand and potentially remedy the challenges that mitochondrial dysfunction brings to health.
Gene Therapy Approaches
Gene therapy presents a promising avenue for addressing mitochondrial diseases. The idea is to deliver mitochondrially-targeted genes to restore normal function. This can involve using vectors to introduce beneficial genes that can compensate for defective ones.
Mitochondrial gene therapy can potentially revolutionize treatment for inherited mitochondrial disorders. For instance, clinical trials are exploring approaches like mitochondrial replacement therapy (MRT), which allows the transfer of normal mitochondria into a cell that has defective mitochondria. The success of such therapies can significantly impact patient outcomes, providing new hope for individuals suffering from these conditions.
Targeting Mitochondrial Dysfunction
Targeting mitochondrial dysfunction involves identifying and utilizing specific compounds that can enhance mitochondrial function or protect them from damage. Research is focused on the development of small-molecule drugs designed to modulate mitochondrial pathways. One example is the drug metformin, which shows promising effects on enhancing mitochondrial biogenesis and improving metabolic functions.
Moreover, addressing oxidative stress is crucial. Compounds such as antioxidants have been studied for their potential abilities to neutralize reactive oxygen species produced by dysfunctional mitochondria. This approach aims to reduce damage and improve overall cellular health, targeting specific pathways that lead to disease symptoms.
Interdisciplinary Studies
Interdisciplinary studies offer a unique framework for advancing our knowledge of mitochondria and health. By combining insights from fields like biochemistry, genetics, and environmental sciences, researchers can create a more holistic picture of how mitochondria function within the broader context of health.
For instance, understanding the impact of environmental factors, such as pollution and nutrition, on mitochondrial performance can lead to preventive and therapeutic strategies. Engaging in interdisciplinary research can facilitate collaboration, pooling expertise from different domains to tackle complex issues related to mitochondrial dysfunction better.
"Mitochondria's role in cellular health extends beyond energy production. Engaging with various fields can lead to profound discoveries that enhance our understanding of these vital organelles."
Overall, the future of mitochondrial research looks promising. As we delve deeper into these future research directions, we pave the way for innovative therapies and a better understanding of the essential functions that mitochondria play in health and disease. Through these efforts, we can hope to address the challenges posed by mitochondrial disorders effectively.
Closure
In this article, the exploration of mitochondria reveals their essential role as powerhouses of cells. This conclusion synthesizes the key insights gathered throughout the discussion, emphasizing the multifaceted functions of mitochondria. Their primary responsibility is energy production, yet their influence extends beyond that foundational aspect. Mitochondria regulate cellular metabolism, manage apoptotic signals, and facilitate various crucial signaling pathways essential for maintaining cellular integrity.
Summary of Key Points
- Mitochondria are integral to ATP production through mechanisms like oxidative phosphorylation and substrate-level phosphorylation.
- Mitochondrial DNA plays a unique role in inheritance and genetic diseases, impacting both individual and population health.
- Mitochondrial dysfunction is linked to a range of pathological conditions, including neurological disorders and metabolic syndromes, underscoring their relevance in health and disease.
- Additionally, the current state of research highlights innovative approaches such as gene therapy and interdisciplinary studies aimed at addressing mitochondrial dysfunction.
Implications for Human Health
Understanding the functions of mitochondria carries significant implications for human health. Their role in energy metabolism connects to various lifestyle factors, including diet and exercise, which can influence mitochondrial performance. Improved mitochondrial health may lead to enhanced energy levels, better metabolic control, and a reduced risk of disease.
Moreover, exploring the impact of mitochondrial health on aging leads to fascinating avenues for research into age-related diseases. As scientists unravel these complex relationships, they reveal potential strategies for therapeutic intervention. Promoting mitochondrial function could thus become a pivotal point in preventing chronic illnesses and enhancing quality of life.
In summary, a comprehensive understanding of mitochondria intertwines cellular mechanisms with broader health outcomes, reinforcing their status as critical targets for future biomedical research.
"Mitochondria are not just the powerhouses of the cell; they are central players in cellular dynamics and health."
For deeper insights, please visit Wikipedia or explore more on Britannica.
Engaging further with the implications of mitochondrial behavior may lead researchers, educators, and health professionals to appreciate their profound impact on cellular biology and human health.