Understanding Fly Vectors in Genetic Engineering
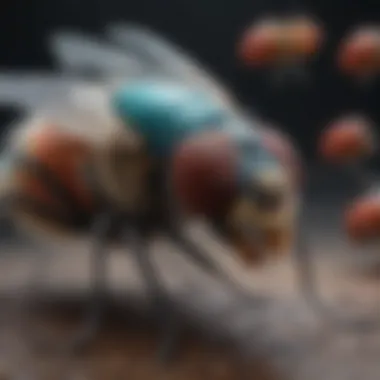
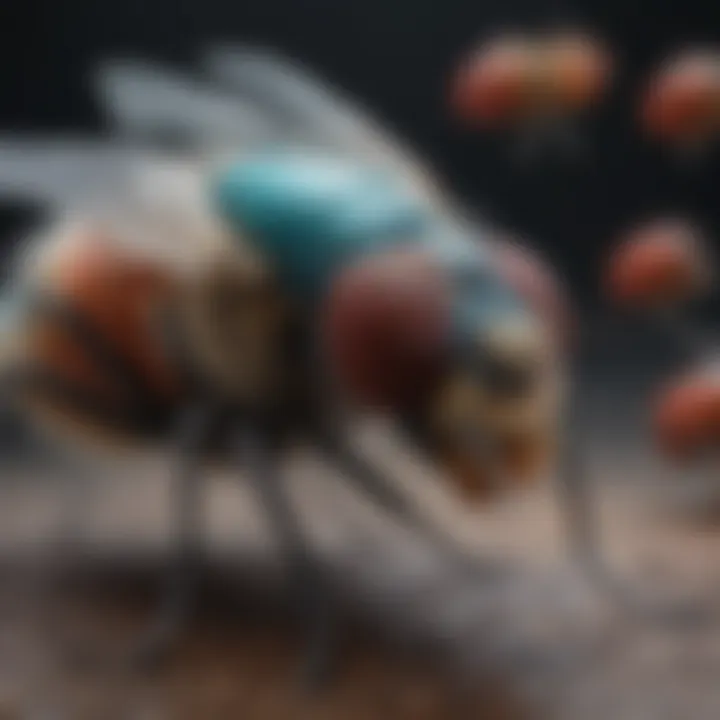
Intro
Genetic engineering often relies on vectors for the transfer and manipulation of genetic material. Among these, fly vectors, particularly those derived from Drosophila melanogaster, have established themselves as foundational tools in the field. Understanding their significance is crucial for grasping advancements in molecular biology.
Historical Context
Drosophila melanogaster has served as a powerful model organism since the early 20th century. With its relatively simple genome and short life cycle, it offers unique advantages for genetic study. Fly vectors emerged as essential tools for manipulating genes within this organism, enabling researchers to explore gene function, regulation, and interactions.
Types of Fly Vectors
There are several types of fly vectors commonly used in genetic engineering. These include:
- P-element vectors: One of the earliest and most widely used vectors, known for their stability and ability to insert transgenes into the fly genome.
- Minicircle vectors: These small vectors provide efficiencies in gene expression and have fewer integration concerns compared to larger constructs.
- AttP/attB systems: These systems enable site-specific integration, allowing for predictable and controlled insertion of genetic material.
Understanding these various types allows researchers to choose the most appropriate vector for their specific objectives.
Mechanisms of Action
Fly vectors typically utilize transposons, sequences of DNA that can change positions within the genome. This transpositional ability is what enables insertion of new genes. Additionally, they can employ various promoter systems to ensure appropriate expression levels of the transgenes. The choice of vector and associated mechanisms greatly influences the outcomes of the experiments conducted.
Advantages and Limitations
Fly vectors carry distinct perks that make them appealing for genetic research. Advantages include:
- High throughput: These vectors allow for large-scale genetic screens.
- Ease of use: Advanced techniques have simplified the process of creating and manipulating vectors.
- Robustness: They can express proteins efficiently in the Drosophila system.
However, limitations also exist. Some potential drawbacks are:
- Insertion site variability, which can lead to unpredictable gene expression consequences.
- Potential for gene silencing in certain genomic contexts.
The successful application of fly vectors hinges on understanding both their capabilities and limitations, making them both powerful and complex tools in genetic engineering.
Applications in Gene Therapy
Fly vectors play a pivotal role in gene therapy research. By analyzing their interaction with specific genes in Drosophila, researchers gather insights that can influence therapeutic approaches in higher organisms. The translational journey of findings from fly models to human disease settings is ongoing, especially in conditions where gene function can be manipulated.
Ethical Considerations
As with any genetic manipulation, ethical concerns arise. Issues such as gene editing's potential long-term impacts and the implications for biodiversity are critical points of discussion among researchers and ethicists alike. Ensuring responsible use of fly vectors is fundamental for the integrity of genetic research.
Methodology
Overview of Research Methods Used
To investigate the significance and application of fly vectors, a multi-faceted approach is often employed. This includes experimental research, computational analyses, and literature reviews.
Data Collection Techniques
Data is collected through various means:
- Laboratory experiments focusing on transgene expression and stability.
- Analysis of genetic screens to identify successful integrations.
- Surveys and interviews with researchers utilizing fly vectors in their projects.
Future Directions
Upcoming Trends in Research
Future research involves enhancing the precision of fly vectors and improving the specificity of gene integration. Developments in CRISPR technology may likely play a role in refining how these vectors are utilized.
Areas Requiring Further Investigation
There remains a pressing need to explore the long-term effects of fly vector-derived modifications. Understanding how these changes perpetuate through generations of flies can illuminate broader biological principles.
Intro to Fly Vectors
In the realm of genetic engineering, fly vectors hold a pivotal role. They are indispensable tools that facilitate the manipulation of genetic material, contributing to both basic research and advanced applications. In particular, fly vectors derived from Drosophila melanogaster, commonly known as the fruit fly, provide insight into the complexities of genetic functions and interactions. Understanding these vectors is crucial for anyone engaged in molecular biology, as they bridge the gap between fundamental biology and practical applications.
Fly vectors serve various functions. They allow scientists to introduce genes into Drosophila and study the resultant phenotypic changes. This study extends to understanding gene regulation, cellular processes, and developmental biology. The ability to modify genetic material using fly vectors embodies a significant advantage in experimental setups.
The considerations surrounding fly vectors extend beyond their immediate utility. They highlight the sophistication involved in genetic manipulation. Researchers must be aware of the vector’s properties, potential for integration, and implications for gene expression. Consequently, a well-rounded knowledge assists in the selection of the appropriate vector for specific experiments, ensuring reproducibility and reliability.
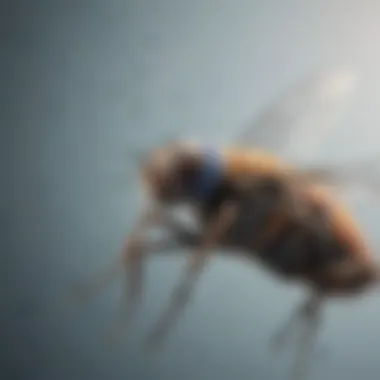
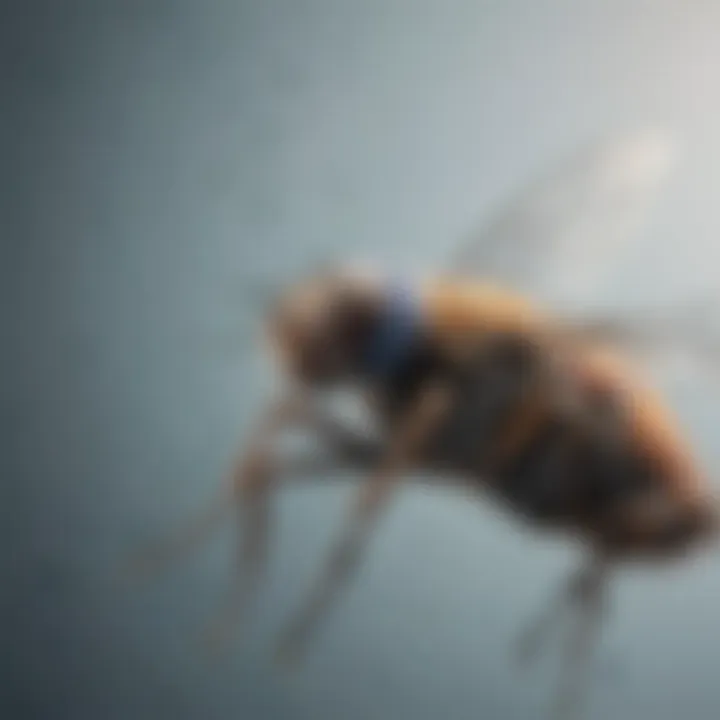
Definition and Purpose
Fly vectors are specific genetic constructs designed to facilitate the transfer of genetic material into a host organism, primarily Drosophila melanogaster. They aid in gene expression, making it possible to analyze the function of genes and genetic regulatory elements. Their purpose ranges from analyzing gene function to producing transgenic organisms for studies in development and behavior.
These vectors are crucial for assays where gene function needs to be observed within a living organism. They enable researchers to insert genes of interest, manipulate regulatory regions, and track gene expression patterns. By doing so, researchers can derive conclusions about the role of specific genes in biological processes, providing clarity to mechanistic pathways.
Historical Context
The use of fly vectors in genetic research has evolved significantly since the early days of molecular biology. Drosophila melanogaster was established as a model organism in the early 20th century. Pioneering work by Thomas Morgan laid the foundation for genetic cross-breeding techniques, providing the initial understanding of heredity.
As molecular biology progressed, so did vector technology. The first fly vectors were simple plasmids introduced to study gene functions. Over time, more sophisticated vectors, including those derived from viruses or transposable elements, came into play. Each iteration improved the functionality of genetic tools available to researchers.
Understanding Genetic Engineering
Genetic engineering stands as a cornerstone of modern biological research, significantly enhancing our understanding of genetics and its applications. Within the realm of genetic engineering, fly vectors, particularly those derived from Drosophila melanogaster, offer unique advantages. These vectors serve as essential tools, facilitating the modification of organismal genomes with unprecedented precision. By manipulating genetic material, researchers can elucidate molecular mechanisms underlying various biological processes, offering insights into developmental biology, disease mechanisms, and potential therapeutic strategies.
As we explore this topic, it is essential to recognize the multifaceted benefits provided by genetic engineering. One of the most profound aspects of this discipline is the ability to study gene function through gain-of-function and loss-of-function experiments. These experimental frameworks allow scientists to dissect the contributions of specific genes in both model organisms and human cells, thereby fostering a deeper comprehension of genetic pathways and interactions.
Furthermore, genetic engineering enables the creation of genetically modified organisms (GMOs), which can have applications in agriculture, biomedical research, and conservation efforts. The implications of these applications reverberate throughout various sectors, making the study of genetic engineering a critical focus for professionals in biology, medicine, and environmental science.
Fundamental Concepts
Genetic engineering involves several key concepts which are foundational to understanding the field. Central to this process is the concept of recombinant DNA technology. This technique involves the insertion of DNA fragments into a host organism's genome, allowing for the expression of desired traits. Scientists commonly utilize various techniques such as restriction enzymes, ligases, and vectors for this purpose.
Vectors are particularly important as they serve as vehicles to carry the foreign DNA into the host organism. Fly vectors, often using Drosophila as a host, exemplify effective usage of these tools due to the organism's well-characterized genetics and short life cycle. The CRISPR-Cas9 technique also has revolutionized genetic engineering by enabling precise genome editing, further enhancing the capabilities of genetic manipulation.
Other fundamental concepts include gene expression control, where various types of promoters and enhancers regulate when and where genes are activated. Understanding these mechanisms is critical to tailoring genetic engineering applications for specific outcomes.
Applications in Research
The applications of genetic engineering in scientific research are vast and diverse. Fly vectors have become indispensable in understanding complex biological systems due to several reasons:
- Model Organisms: Drosophila provides a simple, yet powerful, model for genetic studies. Researchers can study developmental processes, behavior, and disease models similar to humans.
- Functional Genomics: Fly vectors enable large-scale functional genomics studies, allowing researchers to analyze hundreds or thousands of genes systematically.
- Disease Research: By introducing specific mutations found in human diseases into Drosophila, scientists can study the resulting phenotypes and gain insights into disease mechanisms.
In the context of gene therapy, the methodologies developed using fly vectors propel advancements in clinical applications. Technologies arising from this field hold promise for systematic gene replacement strategies that may address genetic disorders in humans.
"The utilization of fly vectors continues to transform our understanding of genetics, showcasing their versatility in multiple research domains."
By grasping these applications, one can appreciate how fly vectors are not merely tools, but pivotal players shaping the landscape of genetic research and therapy.
Types of Fly Vectors
Understanding the types of fly vectors is fundamental in the discipline of genetic engineering. Fly vectors serve as vehicles for transferring genetic material into specific target organisms. The choice of vector can significantly influence the success of genetic manipulation and research outcomes. The primary categorization of fly vectors includes viral vectors, plasmid vectors, and transposable element vectors, each offering unique advantages and challenges. This section delves into these types, considering their significance in advancing genetic research and engineering in organisms like Drosophila melanogaster.
Viral Vectors
Viral vectors are essential tools in genetic engineering due to their high efficiency in transmitting genetic material. These vectors utilize naturally occurring viruses to deliver DNA or RNA into host cells. The advantage of viral vectors lies in their ability to penetrate host cells effectively, leading to higher rates of gene expression compared to other vector types.
Commonly used viral vectors include those derived from adenoviruses, lentiviruses, and retroviruses. Each type has its distinct properties:
- Adenoviral Vectors: Known for their ability to infect both dividing and non-dividing cells. They can accommodate larger DNA inserts, making them suitable for various applications.
- Lentiviral Vectors: These can integrate their genetic material into the host genome, allowing for stable expression of the transgene.
- Retroviral Vectors: Typically used in gene therapy, these vectors also integrate into the host genome but have to infect dividing cells.
While viral vectors are powerful, their use may raise concerns, including potential pathogenic effects and the risk of insertional mutagenesis. Moreover, their production can be complex and resource-intensive.
Plasmid Vectors
Plasmid vectors are circular DNA molecules that replicate independently of chromosomal DNA within a cell. They are widely used because of their simplicity and versatility. Plasmids can be easily manipulated to carry specific genes or regulatory sequences, allowing researchers to control the expression of the inserted genes.
Several characteristics make plasmid vectors attractive for genetic engineering:
- Ease of Manipulation: Genetic engineering techniques such as restriction enzyme digestion, ligation, and transformation can easily modify plasmids.
- High Copy Number: Many plasmids can replicate in the host cell, resulting in high yield of the desired protein or RNA.
- Selectable Markers: Researchers can incorporate antibiotic resistance genes, enabling the selection of successfully transformed cells.
However, plasmids can be limited in their ability to efficiently transfer large genes or multiple genes simultaneously, which can be a significant drawback in certain applications.
Transposable Element Vectors
Transposable element vectors, also known as jumping genes, are segments of DNA that can move within and between genomes. These vectors offer a unique mechanism for gene delivery. They leverage the natural transposition process to integrate genes into the host genome.
The key advantages of using transposable element vectors include:
- Site-Specific Integration: Unlike random integration with other types of vectors, transposable elements can insert genes at specific chromosomal locations, which can lead to more predictable outcomes.
- Stability: Once integrated, transposable elements can be inherited by subsequent generations, ensuring the transgene is maintained in the genetic lineage.
- Multiplexing Capability: They can facilitate the insertion of multiple genes at once, which is particularly useful in generating complex genetic constructs.
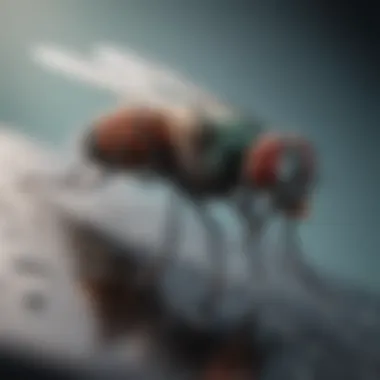
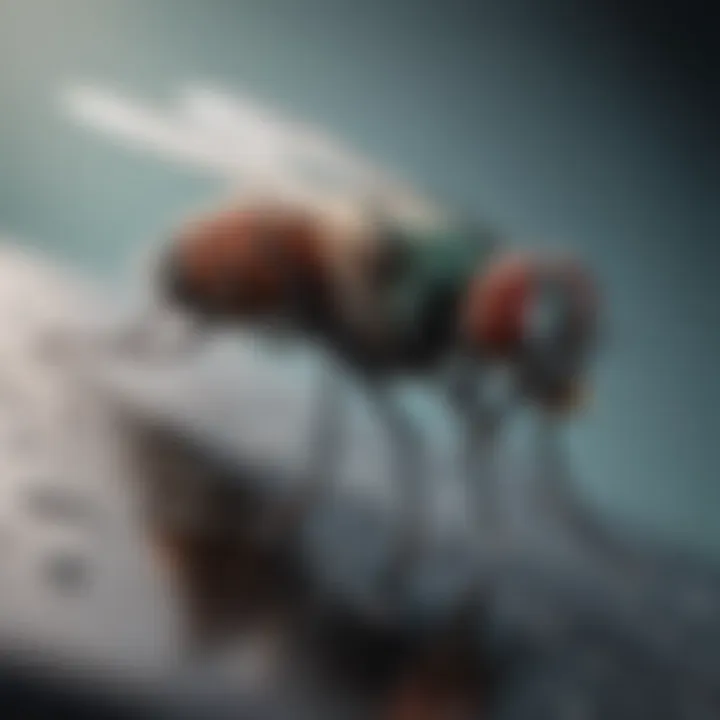
However, the reliance on the host's transposition machinery can create variability in expression levels and may trigger unpredicted effects in the host organism.
Understanding the differences among viral vectors, plasmid vectors, and transposable element vectors is key for researchers looking to optimize genetic engineering approaches in Drosophila melanogaster and other organisms. Each vector type has its specific use cases, strengths, and weaknesses that must be carefully considered in the context of the desired outcome.
Mechanisms of Fly Vectors
The mechanisms of fly vectors play a pivotal role in genetic engineering. Understanding these mechanisms is essential for researchers who utilize these vectors as tools in molecular biology. Fly vectors, particularly those derived from Drosophila melanogaster, have unique functions that facilitate gene transfer into host organisms. This section will delve into two critical mechanisms: transfection processes and the integration of these vectors into the host genome.
Transfection Processes
Transfection processes are the initial step in using fly vectors for genetic engineering. They describe how genetic material is introduced into cells. This is vital for manipulating genes, studying gene function, and developing gene therapies. Several methods can achieve transfection, including chemical methods, physical methods, and biological approaches.
- Chemical Methods: These often involve substances like calcium phosphate or lipofection agents that assist in the uptake of DNA into the cell. These methods can be efficient but may cause toxicity in certain cells.
- Physical Methods: Techniques such as electroporation or microinjection are employed as well. They work by applying an electrical field or using a needle to introduce DNA directly into cells. Though precise, these methods can be labor-intensive and require skilled manipulation.
- Biological Methods: These include the use of viral vectors. Viral vectors are engineered to deliver genetic material and often demonstrate high efficiency in infecting host cells. Fly-derived viral vectors are particularly noteworthy for their ability to target specific cell types effectively.
The choice of transfection method remains a critical consideration. Each method has its advantages and challenges, impacting the efficiency and outcome of the intended genetic modifications.
Integration into Host Genome
After successful transfection, the next significant process is the integration of the fly vector's genetic material into the host genome. This integration is critical for achieving stable expression of the introduced genes. Several pathways facilitate this integration, leading to permanent modifications in the host organism.
Integration typically occurs through a process known as homology-directed repair. In this mechanism, the transfected DNA serves as a template for repairing breaks in the host DNA. This allows the foreign genetic material to be seamlessly incorporated into specific locations within the genome.
**Key Points on Integration:
- Stable Expression**: Integrated genes can be stably expressed across generations, making fly vectors a powerful tool for heritable traits studies.
- Mutagenesis Risks: There are potential risks, including insertional mutagenesis, which can disrupt essential genes.
- Applications in Gene Therapy: Correct integrations in gene therapy can lead to effective treatments, improving patient outcomes.
In summary, the mechanisms of fly vectors—transfection processes and integration into the host genome—constitute the foundation of their efficacy in genetic engineering. Understanding these processes allows researchers to optimize their approaches to gene delivery, ensuring precise manipulation of genetic information.
Advantages of Using Fly Vectors
The utilization of fly vectors in genetic engineering has grown significantly, primarily because of their numerous advantages that facilitate various research and therapeutic applications. Understanding these benefits is crucial as they underscore the relevance of fly vectors in the evolving field of molecular biology. Each advantage offers distinct contributions to researchers and applications, making fly vectors an important focus in genetic engineering.
High Efficiency in Gene Delivery
One of the primary advantages of fly vectors is their high efficiency in gene delivery. This is particularly true in using Drosophila melanogaster as a model organism. Fly vectors can easily introduce specific genes into cells. This process occurs through several methods, including transfection, which results in a higher success rate compared to other commonly used vectors. High performance in gene transfer is essential for ensuring that the desired genetic materials integrate effectively into the host genome, leading to reliable experimental outcomes.
Moreover, the ability of fly vectors to efficiently deliver genes enhances their suitability for both basic research applications and complex therapeutic interventions. The high efficiency in gene delivery not only accelerates the pace of research but also raises the potential for innovation in genetic engineering strategies.
Ease of Manipulation
Fly vectors offer significant ease of manipulation, which is crucial for both educators and researchers. The construction and modification of these vectors can often be accomplished in a relatively straightforward manner. This flexibility allows scientists to customize vectors for specific purposes, tailoring gene expression according to the experimental needs.
Such manipulation is facilitated by simple molecular biology techniques. The modular design of many fly vectors allows for quick alterations. Researchers can easily use cloning tools to insert or modify coding sequences, regulatory elements, and other genetic components with minimal effort. The simplicity in manipulating fly vectors helps bridge the gap between conceptual ideas and practical applications, making them versatile tools in laboratories.
Cost-Effectiveness
The cost-effectiveness of using fly vectors is evident in various experimental setups. Compared to other vector systems, fly vectors require fewer resources for both production and usage. This affordability makes them accessible for a broader range of research applications, particularly in academic settings where budgets may be limited.
In addition, the efficiency associated with fly vectors often leads to lower overall costs in terms of time and materials. When experiments yield reliable results quickly, researchers can reduce waste while maximizing output, further enhancing the cost-effectiveness of their work. Such economic advantages are vital, especially in intensive research projects requiring large-scale testing and validation of genetic modifications.
"The integration of fly vectors into genetic engineering practices not only enhances research efficiency but also drives innovation through accessible and adaptable tools.”
In summary, the advantages of high efficiency in gene delivery, ease of manipulation, and cost-effectiveness solidify the position of fly vectors as integral components in the field of genetic engineering. These benefits highlight how fly vectors facilitate the progress of research, paving the way for new discoveries and applications in molecular biology.
Limitations and Challenges
Understanding the limitations and challenges associated with fly vectors is crucial. While they play a significant role in genetic engineering, it is also important to recognize the hurdles they present. These hurdles can affect both research outcomes and practical applications. Here, we will explore two major issues: the potential for insertional mutagenesis and regulatory hurdles.
Potential for Insertional Mutagenesis
Insertional mutagenesis refers to the disruption of normal genomic functions caused by the insertion of a foreign DNA sequence. Fly vectors, during the process of integrating into the host genome, can unintentionally insert near or within essential genes. This could lead to alterations in the expression of these genes, thereby causing unintended consequences. For instance, a fly model may develop a completely new phenotype that researchers did not anticipate.
Additionally, the consequences of insertional mutagenesis can extend beyond a single experiment. If the transformed fly is used in further studies, these unintended mutations can propagate. Thus, it raises questions about the reliability of results derived from such models. Researchers must scrutinize the potential for these mutations when designing experiments and interpreting outcomes.
"Although fly vectors are powerful tools, their unexpected integration can lead to significant challenges in interpretation of data and results."
Regulatory Hurdles
Regulatory hurdles present a substantial challenge in the deployment of fly vectors. Different countries have varying regulatory frameworks for genetic engineering, which can complicate research and application processes. Before conducting research or applying fly vectors in clinical practices, researchers must often navigate a maze of legal requirements. This can include obtaining permits and demonstrating compliance with safety standards.
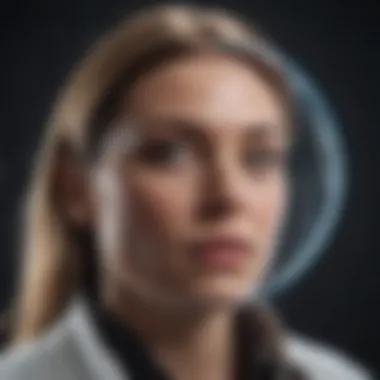
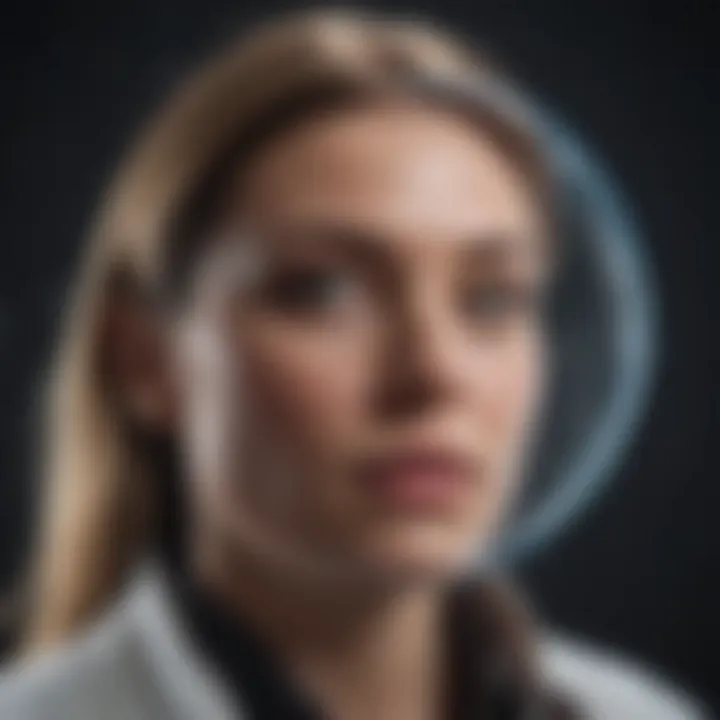
Moreover, regulatory bodies may require extensive documentation and evidence supporting the safety and efficacy of the genetic modifications. Gathering this information can be resource-intensive and time-consuming.
These hurdles can stifle innovation in the field. The need for adherence to regulations can slow down the development of promising applications using fly vectors, especially when collaboration extends across international borders. Understanding and preparing for these regulatory landscapes is essential for organizations seeking to leverage fly vectors effectively in their work.
Fly Vectors in Gene Therapy
Gene therapy represents a groundbreaking approach in modern medicine. Fly vectors are at the forefront of this revolution, particularly in the use of Drosophila melanogaster. This model organism offers a unique platform for investigating gene transfer mechanisms and therapeutic techniques. The simplicity in manipulating the Drosophila genome using these vectors allows for significant advancements in understanding genetic diseases and developing potential treatments.
Therapeutic Applications
The therapeutic applications of fly vectors extend into numerous areas of medicine. One primary use is in the modeling and understanding of human diseases. Researchers utilize fly vectors to introduce and express human genes linked to various conditions. This method helps in understanding disease mechanisms and testing potential therapies.
Moreover, fly vectors are instrumental in scouting for gene therapy approaches for neurological disorders. With the capacity to drive gene expression in specific tissues or at particular developmental stages, Drosophila models can closely mimic human pathophysiology. For instance, they have been used to study the pathology of neurodegenerative diseases such as Alzheimer’s and Parkinson’s. The rapid reproduction cycle of flies accelerates the testing of therapies, reducing the time needed to evaluate gene delivery systems.
Additionally, researchers have explored using fly vectors to deliver therapeutic genes that can combat specific genetic disorders. The use of plasmid and viral vectors in Drosophila allows the expression of genes that encode for products essential for cellular function. This approach mimics gene therapy applied in mammalian cells but is more efficient and less costly in fly models.
Success Stories in Research
Numerous successful applications of fly vectors illustrate their potential in gene therapy. For example, a collaborative study highlighted the use of Drosophila to investigate the efficacy of gene edits using CRISPR technology. In this research, specific genes were targeted, resulting in observable changes in phenotypes. This has paved the way for further exploration in human cells, showcasing the model’s effectiveness in preliminary screening.
"Using Drosophila vectors has allowed researchers to quickly test the functionality of gene therapies before advancing to more complex organisms."
Another success story involves the targeted treatment of genetic defects. Researchers managed to correct genetic mutations responsible for diseases in fly models, demonstrating the proof-of-concept for potential human applications. Such work underscores the vital role that fly vectors play in translational medicine, transitioning from laboratory findings to actual therapeutic applications.
Future Directions in Fly Vector Technology
The journey of fly vectors in genetic engineering does not pause at their current applications; rather, it progressively evolves. Future advancements in fly vector technology promise to unveil new horizons in genetic manipulations. Addressing inefficiencies and enhancing applications in various fields remains paramount. By focusing on state-of-the-art innovations, researchers aim to develop vectors that increase precision in genetic targeting and reduce potential off-target effects, thus ensuring accountability in experimental outcomes.
Innovations on the Horizon
Emerging trends and technologies are likely to reshape the landscape of fly vectors significantly. With advances in CRISPR technology, the integration of fly vectors could become more efficient. The potential for precise gene editing using these vectors might transform traditional methods. Consider the possibilities:
- Next-Generation Sequencing: Enhanced sequencing options will allow for better characterization of fly vectors, thereby ensuring their effectiveness and safety in various applications.
- Synthetic Biology: The merging of synthetic biology with fly vector systems could lead to the creation of custom-designed vectors tailored for specific research purposes.
- Improved Delivery Mechanisms: Innovations in nanotechnology may lead to novel delivery systems for fly vectors, enhancing transfection rates and reducing potential side effects.
The path toward precision genetics is paved with continuous efforts to enhance and refine fly vector technology.
These innovations hold the potential to make significant impacts, paving the way for more reliable applications in genetic research and therapy.
Interdisciplinary Collaborations
The complexity of genetic engineering challenges often calls for interdisciplinary approaches. Future developments in fly vector technology will benefit from collaborations across diverse fields:
- Biotechnology and Robotics: Integrating robotics can streamline the processes involved in vector design, testing, and application.
- Computational Biology: Algorithms and simulations may analyze the behavior of fly vectors in various environments, leading to better predictions and outcomes in genetic modifications.
- Ethics and Policy Frameworks: Engaging ethicists and policymakers early in research can shape the responsible application of fly vectors. This collaborative effort ensures that scientific endeavors align with ethical considerations and societal impacts.
As researchers seek to leverage the strengths of various disciplines, the field can expect more innovative solutions. Such collaborations not only enhance scientific discovery but also help mitigate risks associated with genetic engineering.
Ethical Considerations
The topic of ethical considerations in genetic engineering, particularly concerning fly vectors, is critical for understanding the broader implications of this technology. The use of fly vectors in research and gene therapy raises several ethical questions. Addressing these issues ensures that scientific advancements align with societal values and accept norms.
Debates on Genetic Modification
Genetic modification, especially in fly models like Drosophila melanogaster, sparks extensive debates within the scientific community and society at large. Critics argue that modifying the genetic makeup of organisms could lead to unforeseen consequences in ecosystems and biodiversity. On the other hand, proponents highlight the potential benefits, such as disease resistance and enhanced physiological traits. This dual perspective is vital for discussion as it underscores the need for careful consideration of moral implications in research.
One specific area of concern in these debates is the potential for unintended consequences in modified organisms. For example, there may be risks associated with gene introductions that could disrupt existing biological networks. Additionally, public perception of genetic engineering influences funding and policy decisions. It can either hinder or advance research efforts, thus emphasizing the need for ongoing dialogues about the ethical landscape surrounding these technologies.
Regulatory Framework and Compliance
The regulatory landscape governing fly vectors and genetic engineering is elaborate and crucial. Agencies such as the U.S. Food and Drug Administration (FDA) and the Environmental Protection Agency (EPA) establish guidelines to ensure safe research practices and health standards. Compliance with these regulations is mandatory for laboratories working with genetically modified organisms, as it helps mitigate potential risks to health and environment.
A well-structured regulatory framework not only protects public health but also fosters responsible innovation. It sets a clear pathway for researchers who seek to explore the applications of fly vectors for therapeutic purposes. This framework requires stringent safety assessments and detailed reporting to evaluate the impacts of genetic modifications.
Ensuring regulatory compliance is essential and reflects a commitment to ethical research practices.
Finale
In the realm of genetic engineering, the significance of fly vectors cannot be overstated. Drosophila melanogaster, as a model organism, offers extensive insights due to its unique genetic composition. Fly vectors serve as critical tools for gene manipulation, enabling researchers to explore complex biological processes.
Summary of Main Points
- Fly vectors, especially those derived from Drosophila, provide a reliable means for gene delivery and manipulation.
- Different types of fly vectors, including viral vectors, plasmid vectors, and transposable elements, each come with distinct advantages suited for specific applications in research and therapy.
- Understanding the mechanisms of transfection and genome integration is essential for optimizing the effectiveness of these vectors.
- While fly vectors have demonstrated remarkable efficiency and cost-effectiveness, they also pose potential challenges, such as insertional mutagenesis and regulatory complexities.
"The applications of fly vectors extend beyond basic research into more therapeutic frontiers, showcasing their versatility and transformative potential in modern science."
Final Thoughts on Fly Vectors
The future of fly vectors in genetic engineering appears promising. Their use in gene therapy and personalized medicine is evolving. Continuous advancements in technology aim to enhance their efficiency and specificity. As ethical considerations are increasingly at the forefront of scientific exploration, the responsible application of fly vectors becomes crucial. Fostering interdisciplinary collaborations can further unlock their capabilities. Overall, fly vectors represent an indispensable asset in the toolbox of genetic engineering, enabling significant progress in our understanding of genetics and the potential treatment of genetic disorders.