Exploring Flexible Sensors: Innovations & Applications

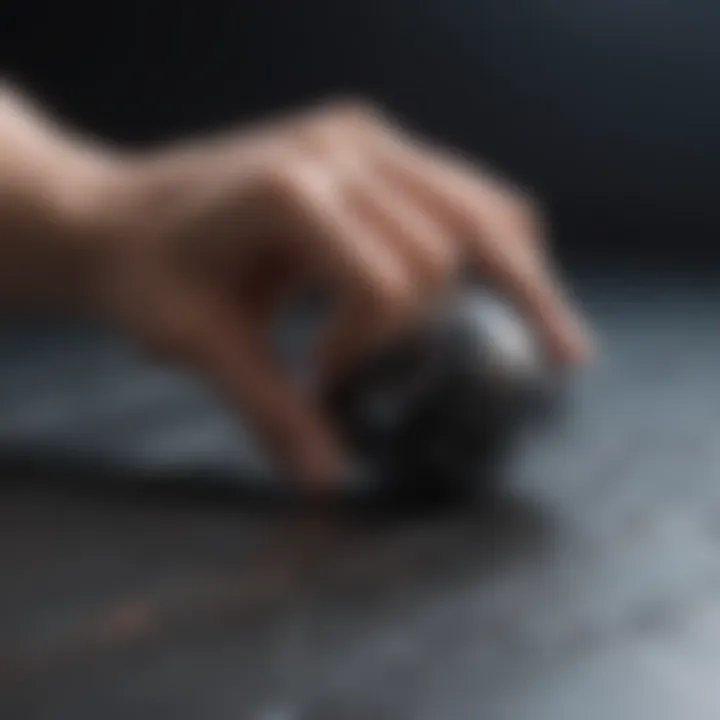
Intro
The rapid advancement in sensor technology is a hallmark of modern innovation. Amongst these developments, flexible sensors have emerged as a promising alternative to traditional rigid sensors. Their ability to conform to various shapes and surfaces opens up new possibilities in numerous fields, notably healthcare and wearable technology.
Unlike conventional sensors, flexible sensors can be integrated into different materials without compromising performance. This article explores the essential aspects of flexible sensors, diving into their operating principles, the materials utilized, and various fabrication techniques. The applications of these versatile sensors across different sectors will also be examined, alongside the challenges they face and the future directions they may take.
In this exploration, flexibility is not merely a physical characteristic. It represents a significant shift in how sensors interact with their environments. By embracing this adaptability, researchers and engineers have the opportunity to push boundaries, creating devices that can not only sense but can also integrate seamlessly into various applications.
The importance of flexible sensors cannot be understated. As we delve into their functionalities and potential, we aim to shed light on the innovations driving their development, ultimately inspiring progress in sensor technology.
Preamble to Flexible Sensors
Flexible sensors have swiftly emerged as a fundamental component in modern sensing technology. Their unique compatibility with various surfaces and scenarios contributes significantly to their growing relevance. The adaptability of flexible sensors allows them to function effectively in environments that traditional rigid sensors cannot accommodate. This section examines the importance of flexible sensors, elucidating their key features, benefits, and considerations that shape their role in various applications.
Definition and Overview
Flexible sensors are devices capable of detecting physical phenomena—such as pressure, temperature, or motion—while maintaining adaptability to non-flat surfaces. Unlike conventional sensors, which may be rigid and restricted to specific formats, flexible sensors can conform to different shapes. This distinctive property signifies a major leap in design versatility, which broadens their potential applications across numerous industries.
The working principle behind flexible sensors revolves around the materials and technologies applied in their construction. Such sensors typically employ conductive materials that can stretch or bend without losing functionality. With advancements in nanotechnology and materials science, the development of flexible sensors has progressed rapidly, resulting in sensors that are light, durable, and highly sensitive.
Historical Context
The conceptual development of flexible sensors dates back several decades, with the field gradually maturing as material technologies advanced. Initial research centered around piezoresistive devices in the late 20th century. However, their adoption was limited due to technological constraints and manufacturing complexities.
The introduction of conductive polymers in the 1990s marked a turning point, allowing for greater flexibility and a wider range of applications. Since then, ongoing research and innovative techniques have led to the proliferation of flexible sensors, driving their incorporation into diverse sectors, such as healthcare, consumer electronics, and environmental monitoring.
Today, the focus lies on refining fabrication processes and enhancing sensor performance, paving the way for more sophisticated applications. As the technical foundation of flexible sensors continues to evolve, it beckons a new era in how we interact with technology and collect data.
"The evolution of flexible sensors represents a pivotal advancement in smart technology design, encouraging interdisciplinary collaboration and problem-solving">
In summation, flexible sensors are more than mere technological novelties; they symbolize a significant shift in how we perceive and utilize sensor technologies in our daily lives. With their increasing importance, an in-depth understanding of their principles, materials, and applications is essential for students, researchers, educators, and professionals.
Fundamental Principles of Flexible Sensors
Flexible sensors have become an essential topic within the field of sensor technology due to their ability to conform to various surfaces without losing measurement fidelity. Understanding the fundamental principles behind these sensors is crucial for several reasons. It helps in grasping how flexible sensors can be effectively deployed in applications ranging from healthcare to robotics. Furthermore, the insights gathered from their operating mechanisms and material properties are foundational to their continuous innovations and advancements.
Basic Operating Mechanisms
Flexible sensors operate based on distinct mechanisms that are primarily focused on their physical properties.
- Mechanical Stretching: Many flexible sensors rely on the principle of mechanical deformation in response to external forces. This deformation results in a change in resistance, which can be measured and interpreted. The sensitivity of such sensors improves with the materials that demonstrate significant strain capabilities.
- Capacitance Variation: Other flexible sensors use capacitance variation as a mechanism. Here, the change in distance between conductive layers due to bending or stretching leads to a change in capacitance. This allows for precise measurements even in irregular or dynamic environments.
- Piezoelectric Effects: Some flexible sensors utilize piezoelectric materials that generate an electrical charge when mechanically stressed. This effect is highly sensitive and can be used to monitor vibrations or pressure changes with remarkable accuracy.
These mechanisms provide different advantages for flexible sensors, enabling their use in diverse applications. Each mechanism's choice often depends on the specific requirements such as sensitivity, range, and environmental conditions.
Material Properties
The performance of flexible sensors is significantly influenced by the material properties used in their construction. Key characteristics include:
- Conductivity: Conductive materials, such as silver nanowires or graphene, are often preferred due to their excellent electrical properties. High conductivity ensures accurate signal transmission.
- Elasticity: The ability to stretch and recover is vital for maintaining functionality while conforming to surfaces. Materials like elastomers are ideal here, as they combine flexibility with durability.
- Chemical Stability: Flexible sensors deployed in medical or outdoor applications require materials that resist chemical degradation. Materials like hydrogels or specially formulated polymers can retain functionality in various environments.
- Biocompatibility: For applications within healthcare, materials must be biocompatible to avoid adverse reactions when in contact with skin or bodily fluids.
Overall, a thorough understanding of the fundamental principles of flexible sensors guides researchers and developers in innovating new designs and optimizing existing technologies, ensuring that they meet the evolving demands of modern applications.
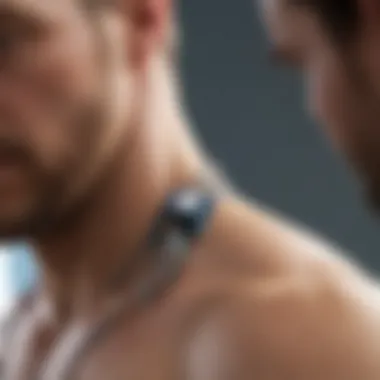
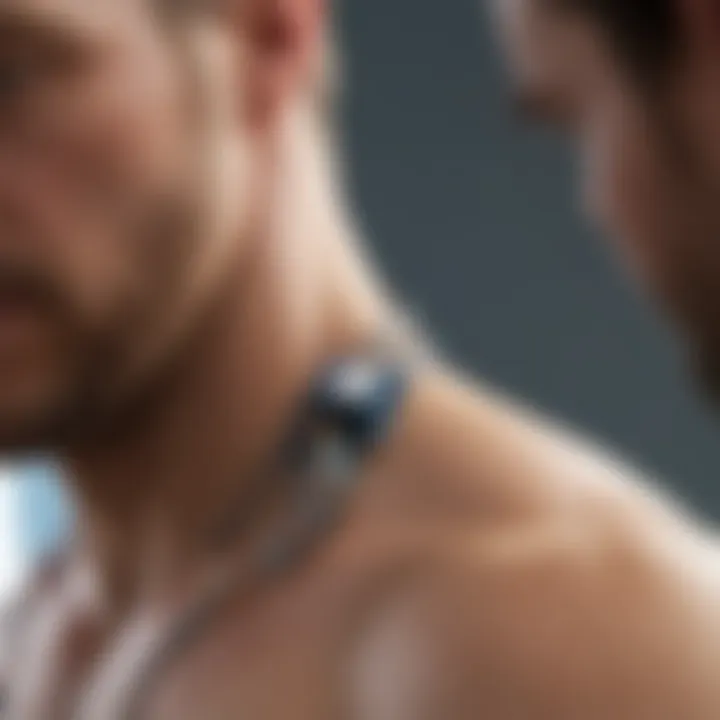
"Flexible sensors are not just about technology; they represent a paradigm shift in how we perceive and interact with our environment." - Unknown
Materials Used in Flexible Sensors
The materials employed in flexible sensors are pivotal in defining their functionality and performance. These materials directly impact the sensor's sensitivity and durability, as well as its adaptability to various applications. A thorough understanding of material properties is essential for the development of advanced sensors that can meet the growing demand in sectors such as healthcare, environmental monitoring and consumer electronics.
Conductive Polymers
Conductive polymers have garnered significant attention in the realm of flexible sensors due to their excellent electrical properties combined with mechanical flexibility. Polymers like polyaniline and poly(3,4-ethylenedioxythiophene) (PEDOT) are commonly used. Their lightweight and tailorable electrical characteristics make them suitable for various applications.
The application of conductive polymers can enhance the performance of sensors in wearable technology. For instance, they can monitor physiological parameters like heart rate and body temperature without hindering user comfort. Notably, they can be integrated into textiles, creating smart fabrics that respond to environmental changes.
Nanomaterials
Nanomaterials, including carbon nanotubes and graphene, represent a cutting-edge advancement in sensor technology. Their unique properties, such as high surface area and exceptional electrical conductivity, enable sensors to detect minute changes in environmental conditions or biological signals.
The integration of nanomaterials allows the development of sensors that are highly sensitive and selective. This means they can detect specific gases or biomolecules at lower concentrations than traditional materials might allow. Furthermore, their nanoscale dimensions help in reducing the overall weight of the sensors, making them more practical for portable applications.
Hydrogels
Hydrogels have emerged as a versatile material in the development of flexible sensors, particularly those for biomedical applications. These water-swollen networks can mimic biological tissues, providing a conducive environment for interaction with biological systems.
Hydrogels can respond to various stimuli, such as pH and temperature changes, making them ideal for sensors that monitor biochemical reactions. The biocompatibility of hydrogels is another advantage, allowing them to be used in wearable devices for continuous health monitoring without causing irritation to the skin.
In summary, the choice of materials in flexible sensors is critical to the enhancement of their performance. Conductive polymers, nanomaterials, and hydrogels each bring unique benefits that pave the way for innovative and practical applications in various fields.
Fabrication Techniques for Flexible Sensors
The realm of flexible sensors is marked not only by their innovative applications but also by the methods used to create them. Understanding fabrication techniques is crucial in enhancing their efficiency, versatility, and applicability. This section examines the primary techniques, each with unique advantages and considerations. The manufacturing process significantly affects the performance, durability, and integration of these sensors in real-world applications.
Print Technologies
Print technologies have revolutionized the fabrication of flexible sensors by introducing methods that are cost-effective and scalable. The techniques include inkjet printing, screen printing, and gravure printing. These methods allow for high throughput and can be utilized on various substrates, including textiles and plastics.
Key benefits of print technologies include:
- Customization: Easily change designs without significant overhead costs.
- Scalability: Efforts can be ramped up for mass production, reducing costs per unit.
- Material Efficiency: Less waste compared to traditional manufacturing processes.
In inkjet printing, conductive inks are sprayed through tiny nozzles, allowing for intricate design layouts. Screen printing uses stencils to apply thick layers of conductive materials, suitable for larger areas. Each method has its place depending on the application needs and material choices.
Layer-by-Layer Assembly
Layer-by-layer assembly is a technique that offers precision control over the sensor's structural composition. This method involves sequentially depositing layers of materials, which can include different types of conductive and non-conductive materials. It provides the opportunity for tailoring electrical, mechanical, and chemical properties.
This technique is significant for achieving:
- Enhanced Functionality: Combining materials with different properties leads to improved sensor performance.
- Customization: Designers can adjust the number and type of layers for specific applications.
- Integration of Components: Sensors can be combined with other electronic components within a flexible format.
The drawbacks primarily involve longer fabrication times and potential complexity in the process, which must be managed carefully.
Transfer Printing
Transfer printing emerges as an efficient method for fabricating flexible sensors. This technique enables the transfer of patterns or circuits from a rigid substrate onto a flexible one. It allows for high-resolution designs while maintaining the integrity of the materials used.
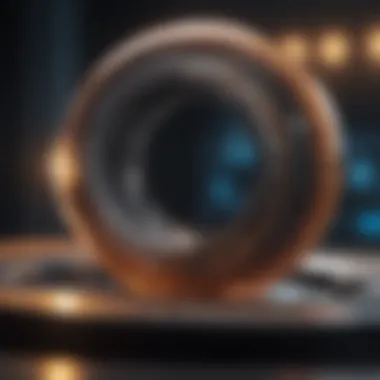
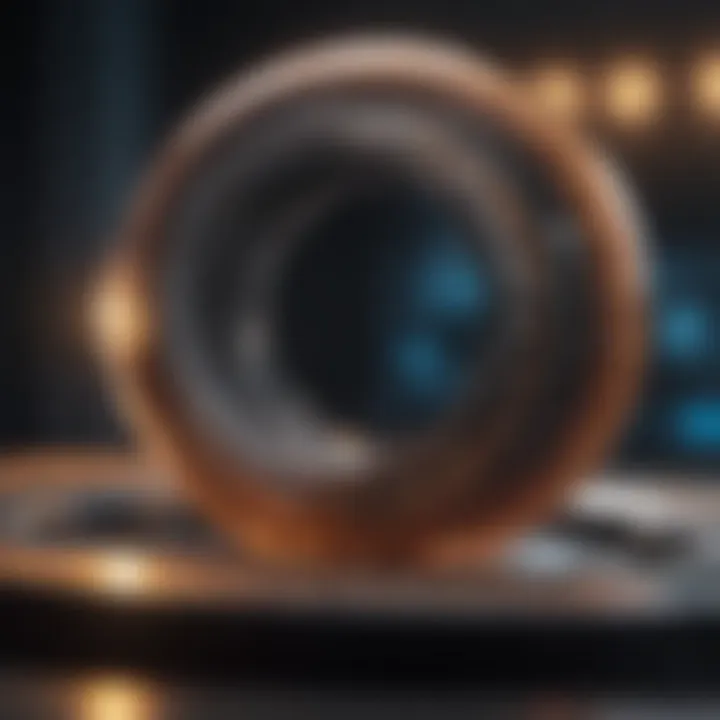
Benefits of transfer printing include:
- Precision: Facilitates fine details in sensor design.
- Flexibility: The ability to work with various flexible substrates is a major advantage.
- Reduced Stress: Minimizes mechanical stress on materials, improving longevity.
Transfer printing can involve multiple layers and materials, making it suitable for more complex sensor requirements. However, the initial setup costs and learning curve can be challenging for newcomers in the field.
"The choice of fabrication technique profoundly impacts the future capabilities of flexible sensors in countless applications."
Understanding these fabrication techniques is essential for students, researchers, and professionals in the field. It allows for better decision-making regarding design, cost, and materials suitable for specific applications.
Applications of Flexible Sensors
Flexible sensors hold significant promise across various fields, as their versatility allows for seamless integration into different applications. The ability to conform to irregular surfaces enables flexible sensors to capture data in ways that traditional rigid sensors cannot. This adaptability enhances user experience and opens the door to innovative uses in multiple areas.
Wearable Health Monitoring
Wearable health monitoring devices have rapidly gained traction in recent years due to increasing health awareness and technological advancements. Flexible sensors are pivotal in this shift. These sensors can be embedded in clothing or accessories to monitor vital signs like heart rate, respiratory rate, and skin temperature.
Such devices can also track physical activity levels, providing users and healthcare professionals with real-time insights. For instance, companies like Fitbit utilize flexible sensors to gather data about user movements. This capability not only aids in personal health management but also enhances rehabilitation efforts for patients recovering from health complications.
Environmental Sensing
Environmental sensing is another vital application of flexible sensors. These sensors play a critical role in monitoring air quality, humidity, and temperature by being strategically placed in urban environments or remote locations. The lightweight and flexible nature of these sensors allows for easy deployment in diverse settings, including hazardous areas.
For example, flexible sensors can be affixed to various surfaces, such as buildings or vehicles, to gather pertinent environmental data. The collected data helps in assessing pollution levels and contributes to efforts aimed at improving public health and environmental sustainability.
Smart Textiles
Smart textiles embody the essence of flexible sensor applications. These textiles integrate sensors to monitor various physical and environmental parameters. For apparel, this means that clothing can detect changes in body temperature, moisture levels, and even muscle activity.
Companies are experimenting with designs that allow the fabric to communicate with smartphones or other devices, ensuring that vital data is easily accessible. This versatility not only enhances functionality but also elevates user comfort and convenience. Furthermore, applications in sports and fashion trends benefit greatly from such technology.
Consumer Electronics
In consumer electronics, flexible sensors are redefining user interactions. Touchscreens on smartphones and tablets have evolved with flexible sensor technology, enabling increasingly responsive touch experiences. Beyond touch sensitivity, these sensors enhance device functionalities, such as gesture recognition and motion tracking.
As electronics continue to evolve towards thinner and more integrated designs, flexible sensors will play a crucial role. The market is now seeing advancements in flexible display technology, where sensors ensure that users can interact with their devices through gestures without physical contact. Such innovations enhance the overall user experience and increase the practicality of devices in daily life.
Challenges in Flexible Sensor Technology
The integration of flexible sensors into various applications presents multiple challenges that researchers and developers must address. Each challenge requires careful consideration to foster continued innovation in this rapidly evolving field. By understanding and overcoming these obstacles, stakeholders can enhance the functionality, durability, and wide adoption of flexible sensors.
Durability and Stability
Durability and stability are critical factors in the performance of flexible sensors. Unlike traditional sensors, which can operate effectively in fixed positions or under controlled conditions, flexible sensors must endure a range of dynamic environments. This includes bending, stretching, and exposure to varying temperatures and humidity levels.
Researchers focus on finding materials that can withstand mechanical stress without compromising performance. Advanced polymers and nanomaterials show promise, but their long-term stability under repetitive use remains a key concern. In some cases, protective coatings can be applied to mitigate environmental effects, but this might add complexity to the fabrication process. Property analysis methods can assist in evaluating these factors accurately.
"The development of highly durable materials is essential not just for the longevity of flexible sensors, but also for their reliability in real-world applications."
Scaling Production
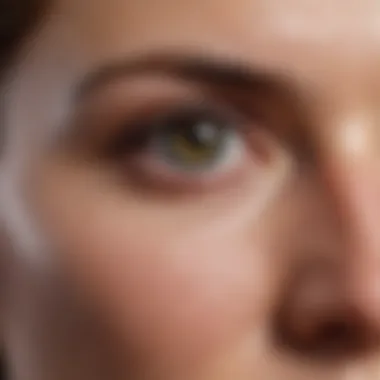
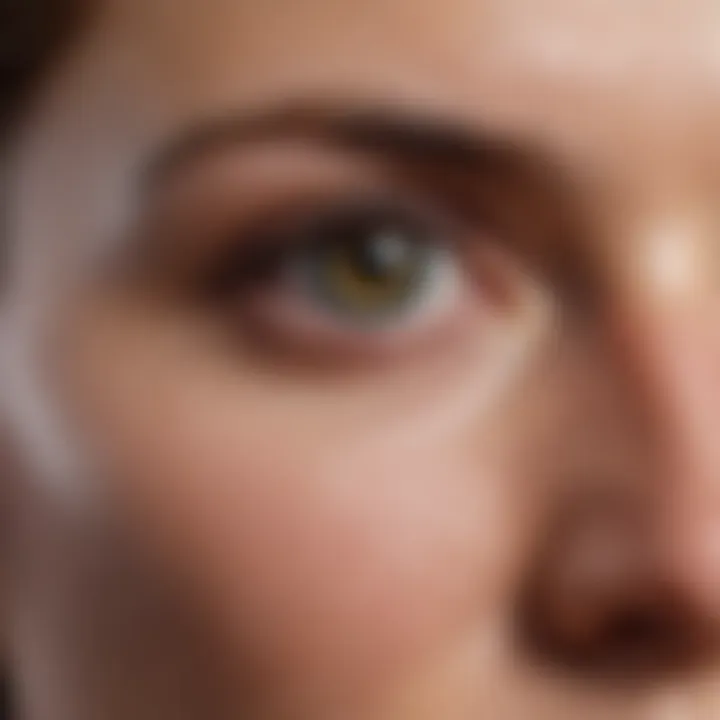
Scaling production of flexible sensors poses challenges that differ from traditional manufacturing. The unique materials involved—such as conductive polymers and nanomaterials—often require specialized processes for large-scale production.
Economies of scale are harder to achieve due to the necessity of maintaining precision in the fabrication techniques. For instance, print technologies may need to be adapted for high-volume manufacturing. Moreover, quality control becomes increasingly significant, as variations in production can lead to inconsistent sensor performance. Addressing these issues is essential for making flexible sensors more economically viable.
Data Integrity
Data integrity is another crucial challenge in flexible sensor technology. Ensuring accurate readings in various conditions is vital for applications in healthcare, environmental monitoring, and beyond.
Factors such as electromagnetic interference, noise, and sensor calibration can affect data reliability. As flexible sensors become integral to IoT devices, the importance of distinguishing between meaningful data and noise magnifies. Developing robust algorithms capable of filtering out inaccuracies is key. Enhancing data integrity will play a vital role in building user trust and expanding the use of flexible sensors across different sectors.
Future Directions in Flexible Sensor Research
The future of flexible sensors is a vast and significant area in technological advancement. This section will explore the emerging trends and directions for research in flexible sensors. Recognizing the potential impact of these innovations can enhance the development trajectories for industries reliant on sensor technologies. Specifically, we will delve into three key aspects: Integration with IoT, Advancements in Materials Science, and Emerging Applications. Each of these elements contributes to the enhanced functionality and versatility of flexible sensors.
Integration with IoT
The Internet of Things (IoT) represents a paradigm shift in how devices communicate and interact. For flexible sensors, integration with IoT is particularly beneficial. It allows for real-time data collection, analysis, and sharing, facilitating smarter systems capable of making data-driven decisions.
- Real-time Monitoring: Flexible sensors embedded in wearable devices can continuously gather health or environmental data, which can then be transmitted to IoT platforms.
- Improved User Interaction: With IoT, users can access data remotely via apps or dashboards, enhancing usability and engagement.
- Automation of Responses: Sensors can trigger automated actions based on data trends, optimizing processes across various industries.
This integration will be instrumental in creating a more connected ecosystem, where responsive and dynamic environments are a reality. By harnessing IoT capabilities, researchers can enhance the adaptability and effectiveness of flexible sensors significantly.
Advancements in Materials Science
Materials science plays a crucial role in the performance of flexible sensors. Continual advancements in this field are vital for creating sensors that not only meet but exceed current limitations.
- New Conductive Materials: Research into innovative conductive polymers and organic materials can lead to lighter and more efficient sensors.
- Enhanced Durability: Improvements can be made in terms of mechanical stretchability and resilience, ensuring longer-lasting sensors that can withstand various conditions.
- Multi-functionality: Exploring composite materials allows for the development of sensors that can detect multiple parameters simultaneously, broadening their application range.
These advancements can revolutionize how flexible sensors are designed and utilized. As researchers continue to innovate in materials science, the existing capabilities of flexible sensors will expand, fostering diverse applications across many fields.
Emerging Applications
As the technology matures, new applications for flexible sensors are appearing, promising to reshape various sectors. Some noteworthy examples include:
- Healthcare: Continuous monitoring of vital signs and environmental conditions can lead to proactive health management.
- Automotive: Integration into vehicle components for improved monitoring and safety features, such as tracking tire pressure or monitoring fatigue levels in drivers.
- Agriculture: Usage in smart farming techniques to monitor soil moisture, crop health, and environmental conditions to optimize yield.
- Smart Homes: Adoption in home automation systems to enhance security and energy efficiency by monitoring occupancy and environmental changes.
Ending
The conclusion of this article captures the essence of flexible sensors and their potential impact on various industries. The discussions throughout this piece underline the significance of understanding both the innovations and applications of these sensors. It emphasizes that flexible sensors are not merely technological advancements, but pivotal components in the evolution of modern sensing technology.
Summary of Findings
The key takeaways from our exploration include the following points:
- Material Diversity: The range of materials used in flexible sensors, such as conductive polymers, nanomaterials, and hydrogels, showcases the adaptability and innovation in design. Each material presents unique advantages that align with specific applications.
- Application Spectrum: Flexible sensors have found applications across multiple sectors, particularly in wearable health monitoring and smart textiles. These advancements highlight their versatility in addressing real-world problems effectively.
- Manufacturing Techniques: Techniques like print technologies, layer-by-layer assembly, and transfer printing enhance the scalability of producing flexible sensors, thus making them more accessible.
- Challenges Ahead: Many challenges remain, such as ensuring durability, maintaining data integrity, and scaling production efficiently. Addressing these issues is critical for the successful integration of flexible sensors into everyday technologies.
- Future Potential: The integration of flexible sensors with the Internet of Things (IoT) opens up new avenues for innovative applications. Research into advanced materials further supports the next generation of flexible electronics.
"The growth of flexible sensor technology promises to transform various industries, creating smarter and more responsive environments."
In summary, this article illustrates that understanding flexible sensors is crucial for advancing technologies that will shape our future. Emerging applications coupled with ongoing research signify a dynamic landscape, urging students, researchers, and professionals to remain engaged in this evolving field.
Citations of Relevant Literature
To ensure the integrity of this article, a variety of high-quality sources were consulted. These include academic journals like Sensors and Actuators A: Physical, which publishes research on sensor technology. Additionally, pertinent literature from conferences and symposiums aids in comprehending the latest advancements in flexible sensor applications and their implications.
Key references are presented below:
- Sensors and Actuators A: Physical: A journal focusing on sensor technology with articles about flexible sensor mechanisms and innovations.
- IEEE Sensors Journal: A top-tier publication that covers multidisciplinary sensor research.
- Nature Materials: This journal offers insight into advanced materials which play an essential role in developing flexible sensors.
Proper citations not only validate this article but also guide readers towards understanding the depth of the field. Each reference has been selected to enhance the discussion around flexible sensors and their diverse applications.