Exploring Solar Cell Materials: Efficiency and Sustainability

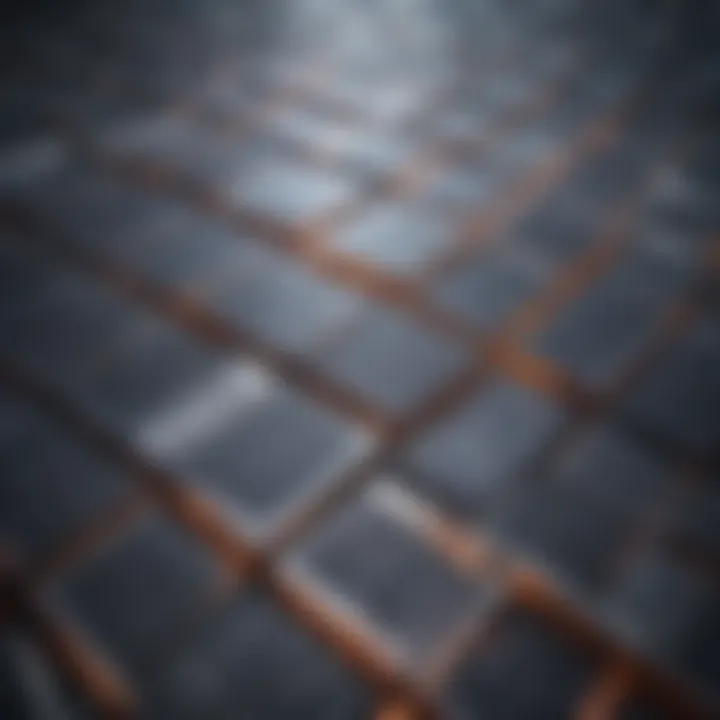
Intro
The transition towards sustainable energy has accelerated discussions surrounding solar cell technology. With an ever-increasing demand for renewable sources of power, understanding the composition of solar cells becomes paramount. These devices, which harness sunlight and convert it into electricity, are not merely products of technology but intricate systems built on a variety of materials and innovative methods.
In this article, we will dissect the core components of solar cells, scrutinizing the materials used in their manufacture, how these materials influence efficiency, and what this all means for the future sustainability of energy generation.
Methodology
Overview of research methods used
To formulate a comprehensive guide on solar cells, a blend of qualitative and quantitative research was conducted. The research encapsulated:
- Literature reviews from peer-reviewed journals.
- Case studies of current solar cell production techniques.
- Analysis of material properties through experimental data where available.
This multipronged approach afforded deeper insights into both traditional silicon-based cells and newer technological developments.
Data collection techniques
Data was collected through various methods, ensuring a robust pool of information. These included:
- Surveys and questionnaires distributed to manufacturers and researchers in the field.
- Interviews with key figures in solar technology research.
- Field studies observing solar cell installations and their performance metrics.
Through these avenues, we gathered critical insights that shaped this analysis.
Materials in Solar Cell Production
The backbone of any solar cell lies in its materials. Predominantly, these can be categorized into three groups:
- Silicon-based materials:
- Thin-film technologies:
- Emerging materials:
- Crystalline silicon: Known for its high efficiency and longevity.
- Amorphous silicon: Offers flexibility but often less efficiency.
- Cadmium Telluride (CdTe): Cost-effective and efficient, particularly in large-scale installations.
- Copper Indium Gallium Selenide (CIGS): Known for its high efficiency in a lightweight format.
- Perovskite solar cells: Gaining traction for their ease of production and high efficiency.
- Organic photovoltaics: While still in early development stages, they present exciting possibilities for flexibility and lower manufacturing costs.
Each of these material classes carries unique properties influencing their respective efficiencies, potential applications, and sustainability implications.
Implications for Efficiency and Sustainability
The composition of solar cells directly impacts their efficiency - a critical factor in energy generation. Research suggests that materials like crystalline silicon tend to offer higher efficiency rates, at least 15-22%, whereas some thin-film options may hover around 10-12%.
Sustainability is equally important, as the production process and material selection can dictate environmental impacts. For instance:
- Silicon extraction can be energy-intensive, raising concerns about its ecological footprint.
- Conversely, thin-film technologies often utilize less material, posing less of a burden on natural resources.
The comprehensive examination of materials and processes in solar cell technology not only shapes their efficiency but also plays a crucial role in meeting global energy demands sustainably.
Future Directions
Upcoming trends in research
Research is constantly evolving. The following trends are emerging:
- Advancements in perovskite materials: Exploring stability and commercial viability.
- Recycling methods for old solar panels to reduce waste and promote a circular economy.
Areas requiring further investigation
While strides have been made, gaps still exist in:
- Understanding long-term performance reliability of new materials.
- Tailoring materials for specific climates and use-case scenarios.
Prelude to Solar Cells
The topic of solar cells holds significant relevance in today’s energy discussions. These technologies represent a shift toward sustainable energy solutions, addressing not just the demand for energy, but also the environmental impact of energy consumption. Understanding all that solar cells entail provides a necessary piece of the puzzle in the larger picture of renewable energy. It's not just about having a cleaner way to collect energy; it’s a robust response to the summit of global warming and finite fossil fuel resources.
Defining Solar Cells
Solar cells, also referred to as photovoltaic cells, harness sunlight and convert it into electricity. This transformation occurs through the photovoltaic effect, where semiconductor materials engage with photons, dislodging electrons and creating an electric current. The basic structure often consists of layers — typically involving silicon, which acts as the primary material. Having a basic grasp of this concept is crucial as it lays the foundation for understanding various types of solar cells and their applications.
The essence of solar cells can be highlighted by several key characteristics:
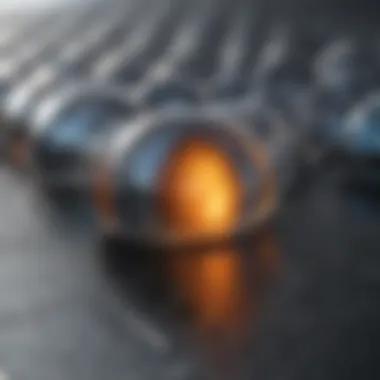
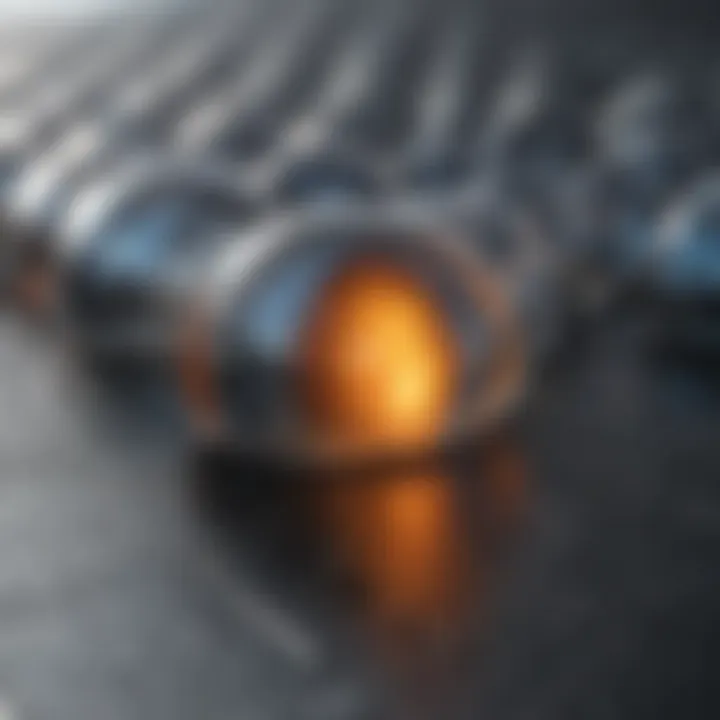
- Conversion Efficiency: This refers to how effectively solar energy is converted into usable electricity.
- Lifespan: Most solar cells are designed to last several decades, making them a long-term investment.
- Cost-Effectiveness: As manufacturing processes improve, the costs associated with producing solar cells continue to decline, making them more accessible.
Historical Context of Solar Cell Development
The journey of solar cells stretches back over a century. The first observed instance of the photovoltaic effect dates to the 1830s when Alexander Edmond Becquerel documented his findings. Fast forward to the 1950s, when researchers at Bell Labs engineered the first practical silicon solar cell, boasting an efficiency level around six percent. This pivotal moment paved the way for an explosion in research and application.
Post-1960s, as space missions grew more ambitious, the demand for reliable power sources in satellites skyrocketed, fueling further advancements in solar technology. During the oil crisis of the 1970s, interest surged in alternative energy, and the race for efficiency began.
Today, ongoing innovations focus not just on silicon but also on various emerging materials and technologies. Such advancements address rising performance demands while considering ecological impacts associated with production methods.
"The development of solar cell technology is but a glimpse into how human ingenuity can reshape our energy narrative."
From this historical context, it can be observed that each technological leap, whether driven by necessity or opportunity, has led to the more complex, efficient, and affordable solar cells we have today. Understanding these backstories and milestones is essential, as they inform both the present landscape of solar energy and its tantalizing future.
Types of Solar Cells
Understanding the various types of solar cells is crucial for grasping the breadth and depth of solar technology. Each category of solar cell comes with its unique materials, efficiencies, manufacturing processes, and applications. By focusing on these distinct types, this article can illuminate the nuances that make solar cell technology both diverse and essential for renewable energy solutions. Investment in solar technology relies significantly on these intricacies, as they define performance, costs, and environmental impacts. Let’s explore these categories in detail, starting with the most widely known options.
Monocrystalline Silicon Solar Cells
Characteristics of Monocrystalline Silicon
Monocrystalline silicon solar cells are often regarded as the gold standard in solar technology due to their high efficiency and longevity. The primary characteristic that sets these cells apart is their construction, which involves a single crystal structure. This uniformity enhances their ability to convert sunlight into electricity. The ideal purity of the silicon used fosters improved electron mobility, enabling significant energy generation even on relatively small panel sizes.
One particularly beneficial aspect of monocrystalline cells is their space efficiency. Because they produce more power per square meter compared to other types, they are invaluable for installations in areas with limited space. However, the manufacturing process is energy-intensive, and that raises certain environmental concerns which are worth considering.
Efficiency Levels Achieved
When it comes to efficiency, monocrystalline solar cells generally boast an impressive range, often achieving efficiencies around 15 to 22%. These levels are significant for both residential and commercial applications, making them a widely popular choice among consumers.
An important thing to note is that efficiency often correlates with cost. While monocrystalline panels may require a heftier upfront investment, the energy yield and durability can justify that expense over time. Their unique feature—better temperature coefficient—allows them to maintain performance in hotter conditions, thus providing reliability in diverse climates.
Polycrystalline Silicon Solar Cells
Manufacturing Process
Polycrystalline silicon solar cells are formed from multiple silicon crystals melted together. This manufacturing process is generally less energy-intensive than that of monocrystalline cells. The cost-effectiveness of producing polycrystalline cells is often appreciated in wider applications, contributing to their popularity in large-scale solar farms.
However, the crystalline structure introduces imperfections, which may lead to slightly diminished efficiency levels compared to their monocrystalline counterpart. A fascinating aspect of their production is the simplicity of melting and cooling—making it a more straightforward process, yet one that doesn't compromise the fundamental capability of harnessing solar energy.
Performance Comparison with Monocrystalline
In the performance spectrum, polycrystalline solar cells tend to clock in at about 13 to 16% efficiency. Though slightly lower than monocrystalline cells, they remain a solid choice based on their affordability. Particularly in scenarios where large surface areas are available and budget is a concern, polycrystalline options offer a better value.
It's essential to recognize that while they may not outperform monocrystalline cells on paper, the cost-to-benefit ratio often attracts consumers who prioritize economical solutions without completely sacrificing performance.
Thin-Film Solar Cells
Materials Used in Thin-Film Technology
Thin-film solar cells utilize a variety of materials, with cadmium telluride and amorphous silicon being among the most common. This technology involves layering thin- film materials onto flexible substrates, giving it an advantage in adaptability and installation versatility.
One of the standout features of thin-film cells is their lightweight nature, which allows for integration into diverse applications, including building-integrated photovoltaics. Their efficiency, however, typically ranges from 10 to 13%, a factor that can deter some users. The unique characteristic of these cells is their ability to perform well in low-light conditions, making them suitable for areas with inconsistent sunlight.
Applications and Limitations
Thin-film solar cells find use in a variety of applications, from consumer electronics to larger solar installations. Their flexibility permits innovative designs, contributing to aesthetic integration within buildings.
Yet, the overall efficiency and space requirements represent a limitation when compared to crystalline options. Users might find themselves needing a larger area to achieve the same energy output, which could influence site selection and design.
Emerging Solar Cell Technologies
Perovskite Solar Cells
Perovskite solar cells have emerged as a promising new player in the solar industry, attracting attention for their rapid efficiency advancements, potentially reaching over 25%. Their unique mineral structure has found its way into solar technology, demonstrating an intriguing ability to absorb sunlight efficiently.
One of the appealing features of perovskites is their relatively easy fabrication process, which could lower the costs substantially compared to traditional silicon cells. However, concerns about their long-term stability and environmental impact, especially related to lead content, require further investigation.
Organic Photovoltaic Cells
Organic photovoltaic cells utilize organic compounds to convert sunlight into electricity. Offering flexibility and lightweight properties, these cells open the door to less conventional applications, such as portable electronics and building-integrated systems.
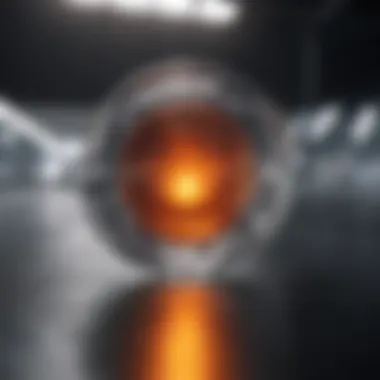
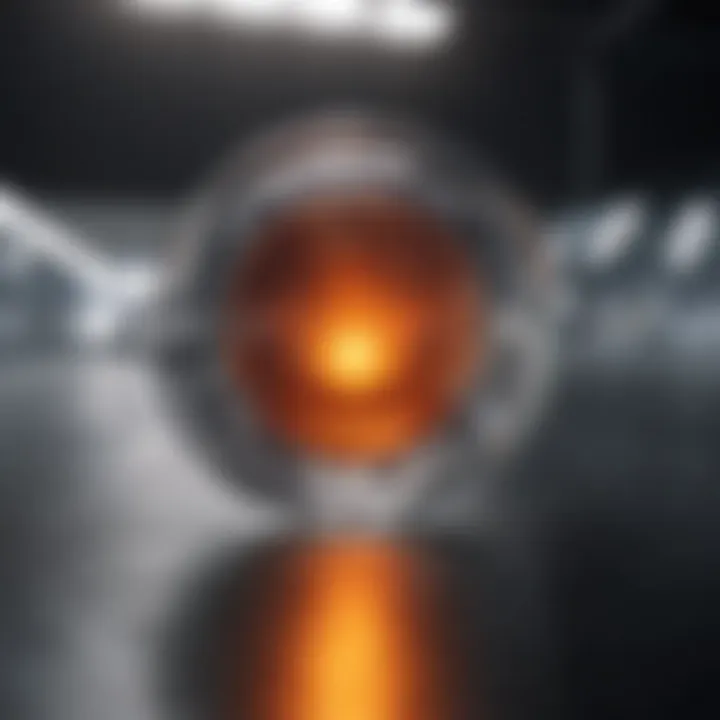
Despite their potential flexibility in application, the efficiency of organic cells generally lags behind silicon-based options, often falling below 10%. Their unique selling proposition hinges on the prospects of being produced with less energy compared to traditional methods. However, as the technology is still in its infancy, more research and development are essential to tackling issues like lifespan and efficiency to propel their broader adoption in the market.
The solar industry is witnessing continuous evolution, highlighting the importance of staying informed about each cell type's advantages and disadvantages—ultimately guiding informed decision-making in renewable energy solutions.
Materials Used in Solar Cells
Understanding the materials used in solar cells is crucial for grasping how these technologies harness sunlight into usable energy. The choice of materials directly influences the efficiency, cost-effectiveness, and sustainability of solar technology. Without getting into the nuts and bolts, we can say that each material brings its own set of benefits and challenges. As we dive into the specifics here, you’ll see how various components either lift or weigh down the potential of solar cells.
Silicon as a Primary Material
Silicon has become the reigning champion in the realm of solar cell materials. It’s like the bread-and-butter of the solar industry.
Cultivation and Purification Processes
The cultivation and purification processes for silicon are pivotal. This method involves extracting silicon from sand or quartz, which is then subjected to high temperatures and chemical treatments. This dual-stage approach ensures that the purity levels reach over 99%, which is essential for optimal solar cell performance.
A key characteristic of this step is efficiency. High-quality silicon enhances energy conversion, enabling cells to capture and transform sunlight more effectively. Silicon, in its polycrystalline or monocrystalline forms, stands as a popular choice for both large-scale solar farms and rooftop installations. The unique feature of the cultivation phase is its potential scalability; it can be ramped up as demand for solar power grows, providing affordability and availability. However, this process does have a downside: it can be energy-intensive, raising concerns about environmental sustainability.
Role of Doping Agents
Doping agents play a significant role in refining the electrical properties of solar-grade silicon. By introducing elements like phosphorus or boron, the aim is to enhance conductivity, and therein lies the beauty of doping: it transforms basic silicon into a semiconductor capable of efficient energy capture.
What makes doping advantageous is its ability to enable a semiconductor's customizability, essentially tailoring it to specific energy outputs desired from solar cells. The intrinsic feature here is that specific dopant concentrations can manipulate how cells behave under different light conditions, which means adaptability. However, the concern arises from the potential risks associated with toxic dopants, warranting a reevaluation of safety protocols in processing.
Cadmium Telluride and Other Compounds
Cadmium telluride has emerged as a viable alternative to silicon, particularly in thin-film solar cells.
Manufacturing Techniques
The manufacturing techniques for cadmium telluride involve simple processes compared to crystalline silicon. For instance, it can be deposited directly onto glass, which speeds up production and reduces costs drastically. This makes it an attractive option for large-scale manufacturing.
A significant characteristic here is the film’s lightweight property, which allows for easy installation and has extensive applications in various settings—from urban rooftops to vast solar farms. The unique aspect is the reduced material use, creating a lower cost per watt compared to traditional silicon cells. Still, this comes with limitations; for example, the efficiency is generally lower than that of silicon cells, and cadmium’s toxic nature raises environmental red flags.
Environmental Impacts
When discussing cadmium telluride, we cannot ignore its environmental implications. The extraction of cadmium during the production process can pose risks to both human health and the environment.
What stands out in this context is the scrutiny this material faces due to its toxicity. It’s a double-edged sword; while cadmium telluride provides a cheaper and efficient alternative, the handling of cadmium involves strict regulations to mitigate risk. The unique feature of evaluating these impacts can lead to better strategies in waste management and recycling of old solar panels, yet skepticism remains about long-term sustainability.
Innovative Materials Under Research
In recent years, there’s been a buzz in the air about innovative materials that promise to reshape the landscape of solar technology.
Graphene and its Potential
Graphene, a one-atom-thick layer of carbon atoms arranged in a hexagonal lattice, presents a game-changing potential for solar cells. Its high conductivity and light absorption make it a subject of intense research.
One intriguing aspect of graphene is its promise to enable flexible solar cells, which will allow for integration into a wider variety of surfaces. This adaptability could expand solar technology’s reach into markets previously thought impractical. However, the challenges persist concerning large-scale manufacturing and cost-effectiveness, which need to be addressed before graphene can be mainstreamed into solar cell production.
Nanostructured Photovoltaics
Nanostructured photovoltaics dive into the realm of manipulating light at the nanoscale to boost solar cell efficiency. By using materials engineered at the nanoscale, these solar cells can harness more of the solar spectrum, enhancing performance.
The pivotal characteristic here is the enhancement of light absorption due to the nanostructuring, which allows for thinner designs without sacrificing performance. However, developing these technologies gears towards complexities in fabrication and the costs involved. If manufacturers can streamline these processes, it may pave the way for a new era in solar energy capture, yet that remains to be seen as research is still in the early stages.
"The materials chosen for solar cells are fundamental to their operation, influencing efficiency, sustainability, and potential applications in a rapidly evolving energy landscape."
In summary, as we unravel the materials utilized in solar cells, we understand that each component—be it silicon, cadmium telluride, or innovative alternatives like graphene—holds unique attributes that contribute to the overall effectiveness and sustainability of solar technologies. These considerations deliver insight into how future developments might continue to shape this critical resource in response to our growing energy needs.
Solar Cell Efficiency Factors
The efficiency of solar cells is a pivotal topic in understanding their performance and adoption in renewable energy. Efficiency factors affect both the output of energy produced from photovoltaic systems and their economic viability. As the world looks for sustainable energy solutions, grasping the intricate details of solar cell efficiency becomes essential. Multiple elements contribute to determining how effectively a solar cell converts sunlight into electricity, which can lead to significant implications for both environmental sustainability and cost-effectiveness.
The Role of Material Properties
Band Gap Energy
Band gap energy refers to the energy difference between the top of the valence band and the bottom of the conduction band in semiconductors. It's a central element concerning how solar cells operate. Materials with the right band gap energy can absorb sunlight effectively and convert that energy into electricity. Generally, a band gap of about 1.1 to 1.5 eV is considered optimal for silicon solar cells.
A key characteristic of band gap energy is its ability to determine which wavelengths of light are absorbed by the material. This characteristic makes it a crucial element to discuss in the article. The beneficial aspect of having an ideal band gap is that it allows solar cells to harness a larger portion of the solar spectrum. However, there's a trade-off; materials with a band gap too wide could miss critical wavelengths, leading to reduced efficiency.
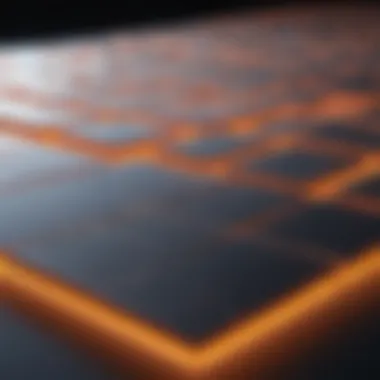
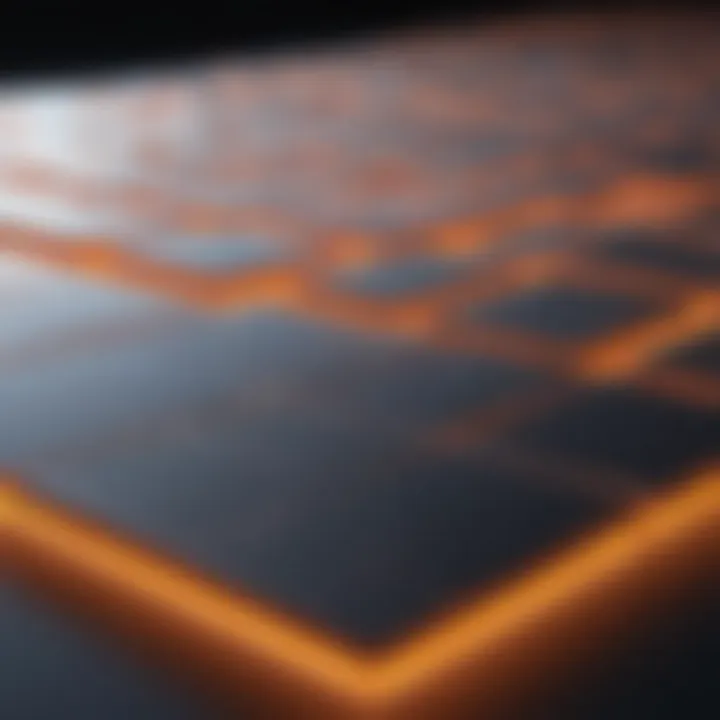
Understanding band gap energy provides insight into what kinds of materials may serve best in future innovations in solar technology, allowing researchers to prioritize and develop them effectively.
Thermal Stability
Thermal stability is another essential aspect that significantly influences the performance and longevity of solar cells. This refers to the material's ability to maintain its properties and efficiency under varying temperatures. High thermal stability is particularly important in areas where solar cells will face temperature fluctuations.
What makes thermal stability a beneficial focus in this article is its direct impact on the operational lifespan of solar cells. If a material fumbles under high heat, it might degrade quicker, leading to increased costs and wasted resources. An interesting aspect is that thermal stability can influence efficiency; a well-designed solar cell can maintain higher performance levels even as temperature rises, allowing for efficient energy production year-round.
Thus, not only does thermal stability enhance durability, but it also contributes to more reliable energy output.
Impact of Design and Configuration
Surface Texturing
Surface texturing involves modifying the surface of solar cells to increase light capture. The principal idea is to reduce the amount of light that reflects off the surface; every little bit helps when collecting sunlight for conversion. A key characteristic of surface texturing is its ability to increase the effective area of light absorption, thereby enhancing overall efficiency.
This technique is a beneficial topic for discussion because when properly implemented, surface texturing can lead to substantial improvements in energy conversion. A unique feature is that different textures can be used depending on the material; for instance, monocrystalline cells can be given a pyramidal texture which dramatically reduces reflection.
However, it is essential to note that while texturing can boost performance, it requires careful engineering to ensure that it doesn't create weaknesses in the structure or impede the manufacturing process.
Layering Techniques
Layering techniques in constructing solar cells allow for multiple thin films to be stacked, which can capture a broader spectrum of light. This approach can effectively increase the efficiency of solar cells beyond what single-layer configurations typically achieve. The notable feature of layering is its ability to optimize performance across various lighting conditions. For example, tandem solar cells made of multiple materials enable better absorption from different parts of the solar spectrum.
The reason layering techniques are worth highlighting in this article is their potential to take solar technology to the next level. However, one must consider that these techniques add complexity and may increase the cost of production.
Sustainability and Environmental Considerations
Sustainability in solar cell technology is not just a buzzword; it's a fundamental aspect that shapes how we view energy production and consumption in today's world. As the demand for renewable energy sources rises, a thorough understanding of solar cells’ environmental impact becomes increasingly vital. The focus isn't merely on harnessing solar energy effectively but also on how these technologies contribute to a sustainable environment. Achieving a balance between energy needs and environmental health is the overarching goal of this examination.
Lifecycle Analysis of Solar Cells
Resource Extraction
The lifecyle of solar cells begins with resource extraction, a critical phase that involves obtaining raw materials like silicon, cadmium, telluride, and various metals. This is where the crux lies; while these materials are essential for solar cell production, their extraction can lead to significant environmental challenges. The environmental cost of mining silcon, for example, includes habitat destruction and water pollution. However, this process is deemed necessary as silicon is abundant and primarily responsible for the efficiency of solar cells. Its celestial abundance makes it a popular option in the solar industry.
Unique in its feature, silicon's purity directly impacts solar cell performance, making meticulous purification processes essential. Yet, these processes can be energy-intensive and have their own environmental repercussions. Balancing the extraction of valuable resources with the potential harm to ecosystems is a dilemma faced by the industry.
End-of-Life Management
As solar technology evolves, managing the end-of-life phase of solar cells has become equally important. When solar panels reach their operational limits—typically around 25 years—their disposal poses another challenge. This aspect of End-of-Life Management deals with how to efficiently handle outdated solar panels to minimize environmental impact.
The key characteristic here is the recycling of materials which allows valuable elements like silicon and metals to be recovered and reused. This strategy benefits the environment as it decreases waste and reduces the need for new raw materials. However, the process still faces hurdles, such as economic viability and the technological capability to recycle complex multi-layered panels. Simplifying the disassembly and recovery process could create significant advancements in sustainability within the solar industry.
Recycling and Material Recovery Strategies
Current Technologies in Recycling
In recent years, recycling technologies have made notable advancements; however, they still have a long way to go. Some current technologies are capable of recovering approximately 90% of silicon used in solar cells, which demonstrates a positive trend. These techniques include mechanical processing and chemical methods to reclaim valuable materials.
The main selling point of these technologies is their potential to create a closed-loop system, effectively reducing the need for new resource extraction. Still, the scalability and cost-effectiveness of such recycling processes remain concerns. Moreover, while solutions exist, the widespread implementation of these techniques hasn’t become mainstream yet, keeping a large volume of waste intact in landfills.
Future Directions in Sustainable Practices
Looking ahead, future directions in sustainable practices within solar technology reflect innovative thinking. There’s a push towards creating solar cells that are easier to recycle from the outset—designing panels with fewer materials or choosing recyclable alternatives altogether. Additionally, research is focused on developing more efficient methods of resource recovery that could significantly reduce the ecological footprint of solar energy.
An emphasis on circular economy principles is becoming evident, where the concept is not just about recycling, but entirely reshaping how solar cells are designed, produced, and disposed of. While many of these ideas are still in developmental stages, their unique features could pave the way for a more sustainable future in solar cell technology. Being proactive in adopting these future sustainable practices could alleviate the environmental pressures caused by traditional methods, thus securing a green alternative for energy production.
"The future of solar energy represents not only a shift in the way we harness power but also a critical evolution in our approach to sustainability."
The End
The conclusion of this article brings to light a number of vital points concerning solar cells, emphasizing their significance in current and future energy solutions. Through various sections, we have traced the journey of solar technology, examining different types of solar cells, the materials that compose them, and the driving factors behind their efficiency. In this fast-paced world, it’s crucial to understand how solar energy can bridge the gap between traditional methods of energy production and the burgeoning need for sustainable solutions.
Summary of Key Findings
In reviewing the body of the article, we can distill several key takeaways:
- Diverse Materials: The materials used in solar cell production are both varied and specialized, ranging from silicon to thin-film compounds. Each has its own set of properties and efficiencies.
- Efficiency Variability: Different solar cell types exhibit distinct efficiency levels that are determined by their composition and design. For example, monocrystalline cells are generally more efficient than their polycrystalline counterparts but may come at a higher cost.
- Environmental Considerations: Lifecycle assessments reveal that while solar cells provide a green energy source, their manufacturing and disposal come with environmental challenges that require ongoing research and innovative recycling strategies.
- Emerging Technologies: There remains a bright prospect for the future with emerging technologies such as perovskite and organic photovoltaic cells, which promise to deliver high efficiencies and lower production costs.
Overall, the findings underscore the importance of understanding the intricate balance between efficiency, sustainability, and technological innovation in the evolution of solar cells.
Future Prospects of Solar Cell Development
As we look ahead, the future of solar cell development appears particularly promising. Key facets that could shape this landscape include:
- Research Advancements: Continued investment in research and development aimed at enhancing materials will likely yield next-generation solar technologies. Researchers are exploring new compounds that offer better efficiency and environmental safety.
- Integration with Technology: The emergence of smart technologies can pave the way for more integrated solar solutions, enabling homes and businesses to utilize solar energy more efficiently.
- Global Energy Transition: As nations grapple with climate change, solar power stands out as a feasible alternative. Policies supportive of renewable energy could expedite solar adoption, paving the way for a renewable energy revolution.
- Cost Reduction: Innovations in manufacturing and scaling up processes are expected to drive down production costs, making solar energy more accessible.