Exploring Proton Pencil Beams in Science and Medicine
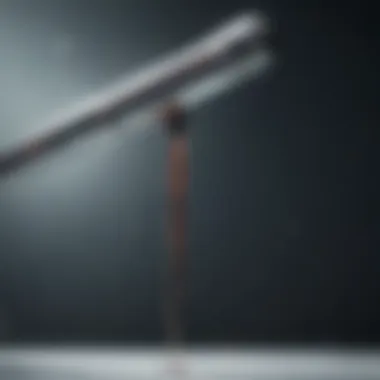
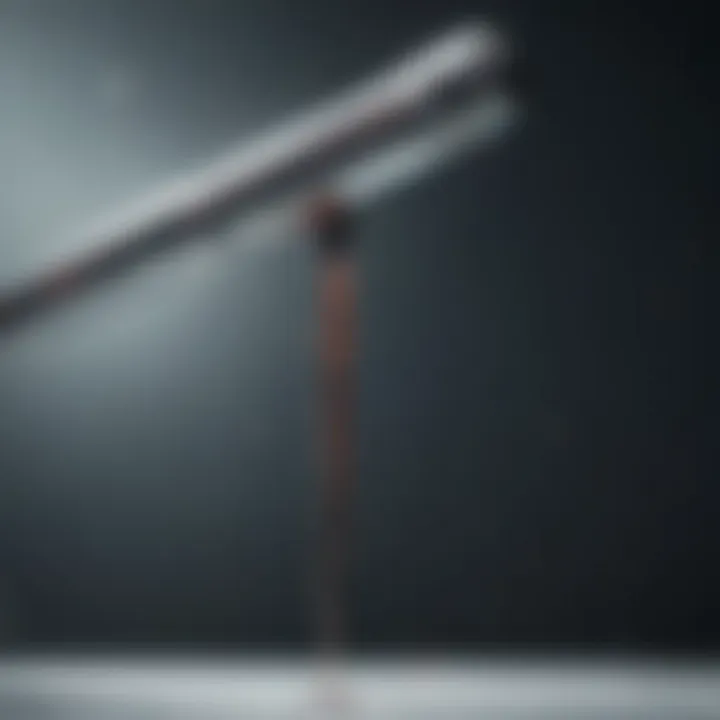
Intro
In the complex world of modern medicine and advanced technology, the use of proton pencil beams has emerged as a compelling subject of discussion. This technique, crucial to the field of particle therapy, focuses on the precise targeting of tumors, particularly in cases of cancer. As cancer treatment continues to evolve, understanding proton pencil beams' specifics—technological intricacies, clinical applications, and future prospects—becomes increasingly important for anyone involved in healthcare and research.
The fundamental principle behind proton therapy rests in the unique properties of protons. Unlike conventional x-rays that can affect surrounding healthy tissue, protons deliver their energy directly to the tumor, minimizing damage to adjacent structures. This characteristic allows proton pencil beams to offer a more refined approach in the fight against cancer, showcasing their growing prominence in oncology.
In this exploration, we will cover several key themes surrounding proton pencil beams, including their operational methodology, advantages over traditional therapies, emerging trends in research, and forthcoming challenges. Engaging with the multimodal implications of this technology lays the groundwork for a deeper comprehension of its relevance in contemporary science and clinical practice.
By drawing on various expertise areas, including physics, biology, and medical care, we illuminate the interconnections that make the study of proton pencil beams an illuminating endeavor. Let’s embark on this journey to unveil what makes proton therapy not just a treatment option but a potentially groundbreaking shift in therapeutic strategies.
Preface to Proton Pencil Beams
Understanding proton pencil beams is pivotal in the landscape of modern medical technology and cancer treatment. Proton pencil beams offer a targeted approach to therapy, minimizing damage to surrounding healthy tissues while maximizing the dose delivered to tumors. This specificity brings a significant advantage over conventional radiation methods, which often affect broader areas, potentially leading to severe side effects.
The reason this topic is so critical lies in the evolution of cancer treatment. As we delve into proton pencil beams, we also explore their journey from theoretical principles to real-world applications. This exploration provides insights into not just effectiveness in treatment but also factors like patient quality of life, which cannot be overlooked. Understanding these beams equips researchers and medical professionals with the knowledge to push boundaries and improve patient outcomes further.
In essence, the significance of proton pencil beams cannot be underestimated. From the intricacies of their definition to the historical milestones that shaped their use, every aspect sheds light on why these beams are hailed as a revolution in cancer treatment. In this exploration, we will unpack these elements and illustrate their broader implications for science and healthcare.
Defining Proton Pencil Beams
Proton pencil beams are precisely focused streams of protons that are used in radiation therapy to treat various forms of cancer. Unlike traditional x-ray beams that scatter over a wide area, a proton pencil beam is concentrated like a laser, intending to target tumors more effectively. The ability to modulate the energy and range of the protons enables clinicians to precisely direct the radiation to the tumor site, minimizing exposure to healthy surrounding tissues and reducing potential side effects.
What sets proton beams apart from standard radiation is their physical properties. Protons have mass, and their behavior involves a distinct interaction with matter. This results in a unique dose distribution that maximizes the radiation dose at the tumor site while tapering off before reaching surrounding healthy tissue.
Historical Context
The journey of proton therapy began in the latter half of the 20th century. Initial explorations into the use of protons for therapy started around the same time as advancements in particle physics were making significant strides. The first clinical trial using protons took place at the Harvard Cyclotron Laboratory in 1961, allowing the medical community to witness the potential benefits of targeted radiation for tumor treatment.
As research progressed, so did the technologies involved. By the late 1990s and into the early 2000s, the development of synchrotron particle accelerators enabled the production of high-intensity proton beams suitable for clinical use, making proton therapy more accessible.
In debate, some have questioned the cost-effectiveness of proton therapy compared to conventional treatments. However, as evidence mounted about its effectiveness for particular types of cancers, more facilities began to invest in this technology, changing the face of oncology treatments altogether.
"The ongoing evolution of proton pencil beams reflects not only technological advancement but a shift in the paradigm of cancer treatment that prioritizes patient well-being."
The historical significance of proton pencil beams signals a turning point in not just cancer therapy but also within the broader scope of medical physics, steering future research and development toward even more innovative and effective treatment options.
Fundamentals of Proton Therapy
Proton therapy holds a cornerstone position in the realm of advanced cancer treatments, standing out due to its precision and effectiveness. This section delves into the core principles that underpin proton therapy. Understanding these fundamentals is central to appreciating the ongoing advancements and challenges associated with proton pencil beams, particularly in the fight against cancer.
The essence of proton therapy lies in its ability to sparingly deliver radiation to cancer cells while minimizing the collateral damage to surrounding healthy tissue. This targeted approach is facilitated by the unique physical properties of protons, contrasting markedly with traditional x-ray radiation therapy. In proton therapy, protons are accelerated to high energies and aimed at tumors. As they penetrate the body, they deposit most of their energy at a specific depth, known as the Bragg peak. This phenomenon allows for the maximal dose being applied directly to the tumor, while significantly reducing the exposure to tissues that lie before and after the cancerous cells.
One significant aspect of proton therapy is its adaptability for treating various types of cancers. The flexibility in adjusting the energy levels of proton beams allows physicians to tailor treatment plans based on individual tumor characteristics. This personalization is crucial, as it optimizes the therapeutic effect while minimizing the potential for damage to healthy cells, which is paramount, particularly in pediatric oncology where young patients are more sensitive to radiation.
Physics of Proton Interaction
The physics behind proton interactions is fundamental to grasping how proton therapy operates. Unlike photons used in traditional radiation therapy, protons are positively charged particles. When these particles collide with atoms in biological tissues, they can cause ionization, leading to cellular damage. However, the interaction dynamics leave a smaller impact on healthy tissues compared to photons due to the ability to target tumors more precisely.
At the atomic level, when a proton pierces through the tissue, it effectively transfers energy to the electrons orbiting the nucleus. This energy transfer can lead to the ionization of atoms and the subsequent breakdown of molecular bonds, resulting in DNA damage within the cancerous cells. However, because protons have mass, they can be manipulated to deposit their energy exactly where needed, effectively stopping upon reaching the tumor and producing the aforementioned Bragg peak. This characteristic is what makes proton therapy so appealing, as it creates a favorable balance between treating malignancies while preserving healthy tissues.
Moreover, the energy levels of the protons can be varied based on the depth of the tumor, allowing for a customized treatment strategy.
"The strength of proton therapy stems from its ability to deliver precise radiation at a controlled depth, significantly reducing treatment side effects."
Comparison with Traditional Radiation Therapy
When weighing proton therapy against traditional radiation methods, several stark differences come to light.
Proton therapy typically boasts a sharp dose distribution, resulting in reduced dose to nearby healthy structures compared to conventional X-ray therapies, which release energy in a more scattered manner. The latter often leads to unintended side effects as healthy cells absorb higher doses of radiation than necessary.
Additionally, proton therapy is less likely to induce secondary cancers, a worrying risk often associated with higher doses from traditional therapies. In a biological context, minimizing exposure to radiation can have profound implications for long-term patient health.
In summary, the fundamentals of proton therapy show a clear advantage in terms of precision and patient safety. This section establishes the importance of understanding these principles, as they set the groundwork for deeper discussions on proton pencil beams and their innovative applications in cancer treatment.
Mechanics of Proton Pencil Beams
The mechanics behind proton pencil beams plays a vital role in understanding how these beams are generated, controlled, and utilized in various applications, especially in the field of medicine. The precision that proton pencil beams can offer in cancer treatment is unrivaled by traditional therapies. By delving into the intricate processes involved in generation and modulation, we can better appreciate the technology's potential and limitations.
Beam Generation Processes
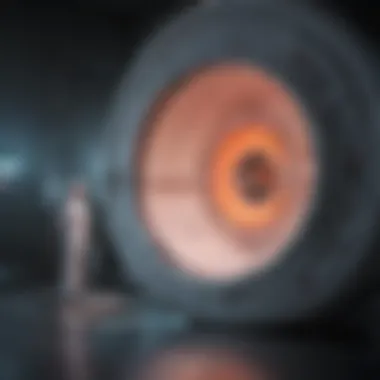
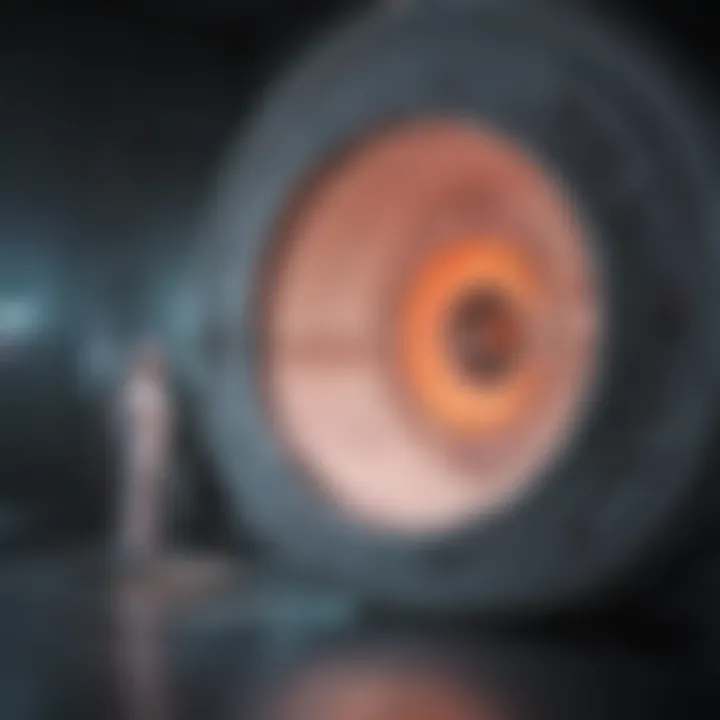
Generating a proton pencil beam is no small feat. It begins with the acceleration of protons, which are subatomic particles tightly bound in atomic nuclei. Proton accelerators, such as cyclotrons or synchrotrons, play a pivotal role here. These machines accelerate protons to high energies, often exceeding hundreds of millions of electron volts.
In a more straightforward manner, one could liken this process to a sprinting athlete. Just as the runner must gather speed before reaching maximum velocity, protons require careful acceleration to unleash their potential in treatment scenarios.
Once protons reach the desired energy, they must be finely focused into a pencil beam. This involves complex systems of magnetic fields and optical components that shape the beam's profile. The goal here is to create a narrow, concentrated beam that enhances targeted delivery while minimizing side effects on surrounding healthy tissue.
"The precision of proton pencil beams allows for a delivery of treatment while sparing healthy cells, a cornerstone of effective cancer therapy."
In practical application, various methods are utilized to achieve this concentration, such as a combination of passive and active beam shaping techniques. These processes help in adjusting the beam's energy and dose to match the contours of a tumor, providing a tailored approach to treatment.
Control and Modulation Techniques
To ensure that proton pencil beams deliver their full therapeutic potential, the control and modulation of these beams are critical. This process involves several factors, including the adjustment of beam intensity, energy, and direction.
Several techniques have been developed to control the proton beams effectively. One of the most effective methods is the use of collimators. These devices help shape the beam, allowing it to match the tumor precisely, akin to a skilled craftsman trimming wood to fit a delicate design.
Moreover, modulation techniques manipulate the energy distribution within the beam. Uniform and non-uniform modulations can be applied to ensure the right dosage reaches the intended area while minimizing exposure to healthy tissues. For instance, intensity-modulated proton therapy (IMPT) offers a sophisticated way to sharpen the dose delivery, particularly beneficial in treating complex shapes or moving targets like lung tumors.
In addition, integrating real-time imaging technologies allows clinicians to adjust the beam's path during the treatment. This adaptability highlights the dynamic nature of proton therapy. Overall, effective control and modulation techniques are instrumental in optimizing treatment outcomes, emphasizing the significant advancements in this field.
Clinical Applications of Proton Pencil Beams
Proton pencil beams have emerged as a transformative tool in cancer therapy. The precision associated with these beams enables healthcare professionals to target tumors more accurately than conventional methods. This minimizes damage to surrounding healthy tissues, which is a critical consideration in medical treatments. The clinical applications of proton pencil beams are vast, impacting a variety of cancer treatment protocols. Here, we delve into the specifics of their usage, particularly their advantages for cancer types, patient selection criteria, and unique applications in pediatric patient care.
Proton Therapy for Cancer Treatment
Types of Cancers Treated
Proton therapy has demonstrated great efficacy in treating several cancer types. Commonly treated cancers include
- prostate cancer
- brain tumors
- liver cancers
- lung cancer
- melanoma
One notable reason for its effectiveness in these cases is the Bragg peak, a phenomenon where protons deposit most of their energy directly at the tumor site. This unique feature allows for a highly localized treatment approach, which is beneficial since tumors can be situated near vital organs.
Given it spares normal tissues, proton pencil beams are particularly popular among those looking for alternatives to traditional radiation treatments that often come with significant side effects. The selective nature of proton therapy means patients can enjoy a better quality of life during and after treatments, which makes this method stand out in modern oncology.
Patient Selection Criteria
When considering patients for proton therapy, several factors come into play. This selection hinges on:
- Tumor size and location
- Age of the patient
- Previous treatments
For instance, tumors situated in critical areas, like near the spinal cord or optic nerves, may qualify for proton therapy primarily due to the high precision offered. This meticulous selection process ensures that patients most likely to benefit from proton treatments are those chosen. However, it is equally important to recognize that not all cancer types are ideal for this approach, which could limit options for some patients. Despite these considerations, the advances in proton therapy have bolstered its role in modern cancer treatment regimens.
Pediatric Applications
Benefits for Child Patients
Children often present unique challenges when it comes to cancer treatment, due to their developing bodies. Proton therapy offers distinct advantages for pediatric patients, which include:
- Reduced risk of long-term side effects
- Less damage to healthy growth tissue
- Enhanced treatment adherence
Considering that many childhood cancers occur in areas with a high density of critical structures, the gentle nature of proton therapy means that little to no collateral damage occurs. As parents search for the most informed options for their children, the security provided by treatments promising minimal long-term side effects is invaluable. By having the option to opt for proton pencil beams, families feel a sense of agency in guiding their treatment journey.
Long-term Outcomes
When evaluating the long-term outcomes of proton therapy in children, the data has been promising. With lower instances of secondary cancers and other late side effects compared to traditional methods, proton therapy presents a compelling case. Key factors influencing these outcomes include:
- Early treatment initiation
- Continuous monitoring
Parents can rest a little easier knowing that the statistical data supports favorable long-term survival rates. Proton therapy not only addresses immediate cancer concerns but also prioritizes these children’s futures with thoughtful care protocols. However, continued research remains vital for expanding knowledge in this area, as ongoing studies seek to fully understand how the long-term results evolve over time.
Understanding the clinical applications helps pave the way for innovative solutions by leveraging the specificity and safety of proton pencil beams in cancer treatments.
Advantages of Using Proton Pencil Beams
Proton pencil beams stand out in the realm of medical physics, particularly in oncology, due to their unique characteristics and advantages over traditional radiation methods. These beams offer significant benefits, especially in terms of treatment precision and patient safety. As cancer treatment evolves, understanding these advantages is crucial for both practitioners and patients alike.
Reduced Damage to Healthy Tissue
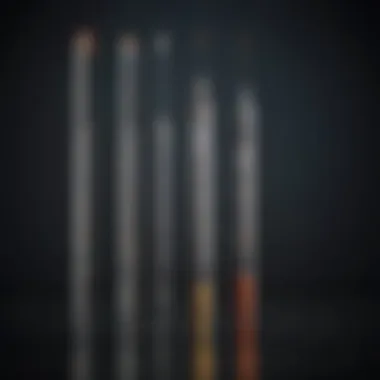
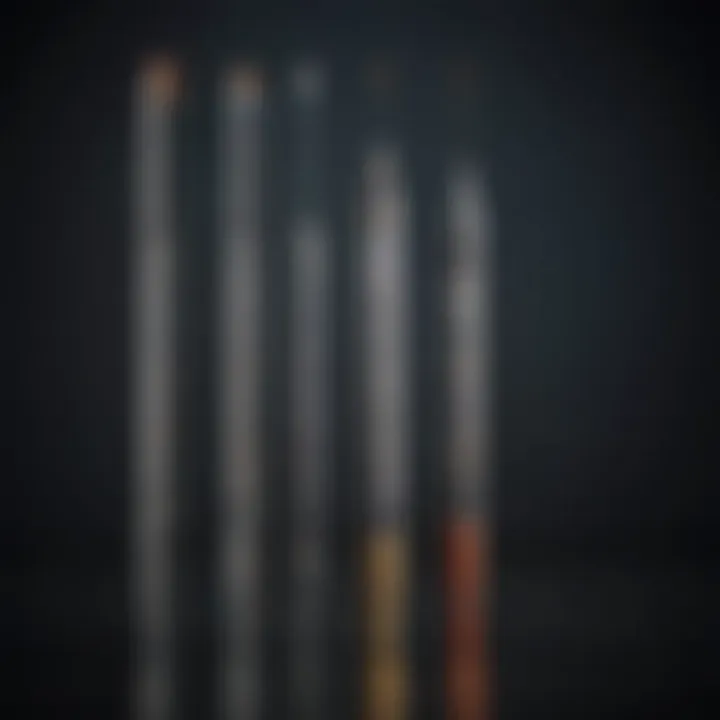
One of the primary advantages of utilizing proton pencil beams is their capacity to minimize collateral damage to surrounding healthy tissue. Traditional X-ray therapies tend to impart radiation both to the tumor and the healthy tissues situated in the path of the beam. In contrast, proton therapy exploits the physical properties of protons, which can deliver their maximum energy precisely at the tumor's location before coming to a halt. This behavior is known as the Bragg peak.
"By precisely targeting tumors and sparing healthy tissues, proton therapy transforms the treatment landscape, making it a beacon of hope for many patients."
- The ability to target tumors while preserving healthy tissues is especially instrumental when treating cancers located near critical organs, such as the brain or spinal cord.
- In pediatric medicine, where growing tissues are particularly vulnerable, this advantage becomes even more profound, reducing the likelihood of long-term complications.
- Furthermore, dose distribution via proton beams can be designed to fit the 3D shape of the tumor, yielding even more refined targeting compared to conventional methods.
In summary, the reduced damage to adjacent healthy tissue not only improves the quality of life post-treatment but also opens doors to more aggressive therapies for tumors that might otherwise be deemed inoperable.
Enhanced Dose Distribution
Complementing the significant reduction in healthy tissue damage, proton pencil beams offer enhanced dose distribution, allowing for precise control over the amount of radiation delivered to specific areas. This feature plays a pivotal role in optimizing treatment outcomes.
- Conformality: Proton therapy's proton pencil beams can be modulated to create a dose distribution that conforms tightly to the tumor's shape. This is invaluable when managing irregularly shaped tumors or those in complex anatomical sites.
- Intensity Modulated Proton Therapy (IMPT) takes this a step further by varying the energy levels of the protons, enhancing the dose escalation, thus increasing the radiation dose to the tumor without amplifying exposure to surrounding healthy tissues.
- Clinical studies have indicated that improved dose distribution translates to higher tumor control rates and better patient survival outcomes in specific cancers.
With the advancements in beam technology enabling more sophisticated treatment plans, it is clear that the enhanced dose distribution not only optimizes the therapeutic index but also aligns treatment with each patient's unique tumor characteristics. This precision ultimately leads to increased effectiveness in cancer treatment, affirming the role of proton pencil beams as a significant innovation in oncology.
Challenges in Proton Beam Therapy
The journey of integrating proton beam therapy into mainstream oncology comes with a multitude of hurdles that can't be ignored. While the benefits in cancer treatment are well-documented, the implementation of this advanced technology often faces practical challenges. These challenges not only influence the cost and accessibility of proton therapy but also impact the quality of care and treatment outcomes for patients. Understanding these obstacles is crucial for stakeholders aiming to optimize the delivery of this promising treatment modality.
Infrastructure Requirements
To deliver proton beam therapy effectively, specialized infrastructure is essential. Proton therapy facilities require significant investment in sophisticated machinery, including cyclotrons or synchrotrons for proton acceleration. Such equipment must be housed in specially designed bunkers due to the high levels of radiation produced during therapy.
Key considerations for infrastructure include:
- Location: Proton therapy centers are often large and can require substantial space. The prerequisites include adequate shielding to minimize radiation exposure to surrounding areas, making site selection critical.
- Maintenance and Upkeep: Regular maintenance of proton therapy equipment is necessary to ensure its efficiency and safety. This ongoing requirement can stretch budgets, particularly in facilities that are already financially constrained.
- Technological Integration: Seamlessly integrating advanced imaging technologies (like MRI and CT scans) with proton therapy is important. Each system must work together effectively for precise targeting, which adds another layer of complexity.
As such, establishing a proton therapy center is not just about purchasing equipment; it’s about the whole ecosystem of technology, training, and ongoing support.
Cost Implications
The financial burden associated with proton beam therapy cannot be understated. Starting a proton therapy program involves significant capital investment—often running into hundreds of millions of dollars. This includes not just the machinery, but also the construction of the facility and hiring of specialized personnel.
Considerations regarding costs include:
- Initial Investment: The upfront capital required for proton therapy machines is much higher than traditional radiotherapy. This high cost creates barriers for many healthcare institutions.
- Operational Costs: Beyond initial expenditure, operational costs, including personnel training, maintenance of equipment, and facility operation, must also be factored in.
- Insurance and Reimbursement: Depending on the region, insurance coverage for proton therapy may not be extensive. Many patients face out-of-pocket expenses, which can deter them from seeking this treatment.
In a world where healthcare budgets are often tight, the cost implications of proton beam therapy pose a serious challenge. Stakeholders need to explore creative funding solutions and demonstrate the long-term value and cost-effectiveness of such therapies.
Knowledge Gaps in Treatment Protocols
Despite advances in proton therapy technology, there's still a certain level of ambiguity regarding treatment protocols. This can lead to inconsistencies in patient care and outcomes. A unified and comprehensive understanding of how, when, and for whom proton therapy should be deployed is crucial for maximizing its therapeutic potential.
Key aspects of knowledge gaps include:
- Standardization of Practices: There is a lack of universally accepted guidelines for the application of proton therapy across different cancer types. This can create disparities in patient care and affect clinical trial results, complicating the advancement of knowledge in the field.
- Ongoing Training: Healthcare providers specializing in proton therapy must receive continual education to stay abreast of the latest research and best practices. However, the rapid evolution of the field can sometimes leave practitioners scrambling to catch up.
- Research Needs: More robust clinical studies are required to understand the ideal scenarios for proton therapy application. This is especially true for unique populations such as pediatric patients or individuals with rare cancers.
In summary, while proton beam therapy holds great promise, overcoming the challenges discussed is essential for its wider adoption and success in clinical practice. By addressing infrastructure needs, managing costs, and closing knowledge gaps, the future of proton therapy can indeed shine a little brighter.
Innovations in Proton Pencil Beam Technology
In the realm of cancer treatment, the emergence of proton pencil beams marks a substantial leap forward. As we explore the innovations in this technology, it becomes clear that their significance extends beyond mere medical applications; they are shaping how we understand and approach complex diseases. Advances in this field not only enhance the precision of treatments but also open new avenues for research and development.
Advancements in Proton Accelerators
Proton accelerators are the backbone of proton therapy systems, enabling the generation of high-energy proton beams essential for targeting tumors. Recent innovations in these accelerators have focused on several key areas:
- Increased Efficiency: Modern proton accelerators boast improved energy conservation, meaning that more energy can be directed toward the treatment itself, enhancing its effectiveness.
- Compact Designs: The advent of compact cyclotrons and synchrotrons has reduced the physical footprint of proton therapy facilities, making them more accessible to hospitals that may not have the space for traditional, larger setups.
- Real-Time Monitoring: Enhanced beam monitoring allows for real-time adjustments during therapy sessions. This precision ensures that the proton beam can be recalibrated to adapt to patient movement or changes in tumor positioning, further increasing treatment accuracy.
Technological advancements in proton accelerators are vital in not just improving existing treatment protocols but also facilitating the development of new methods that can be applied to novel cancer types or stages.
Emerging Research Directions
As proton pencil beam technology evolves, so does the landscape of research surrounding it. Several promising directions are emerging, reflecting the interdisciplinary nature of this field:
- Combination Therapies: Researchers are investigating how proton therapy can be effectively combined with other treatment modalities, such as immunotherapy and targeted drug therapies, to enhance overall patient outcomes. This integrated approach seeks to maximize tumor control while minimizing side effects.
- Personalized Medicine: Innovations in imaging and genomics are paving the way for more personalized proton therapy. By tailoring treatment plans based on individual tumor genetics or metabolic profiles, practitioners can optimize the therapeutic response.
- Novel Applications: Beyond traditional malignancies, scientists are exploring the potential of proton pencil beams in treating non-tumor conditions, such as certain neurological disorders. The precision afforded by proton beams might provide new, safe avenues for intervention in these cases.
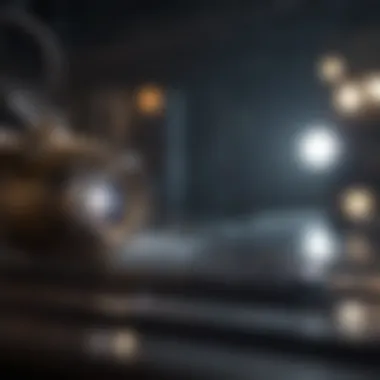
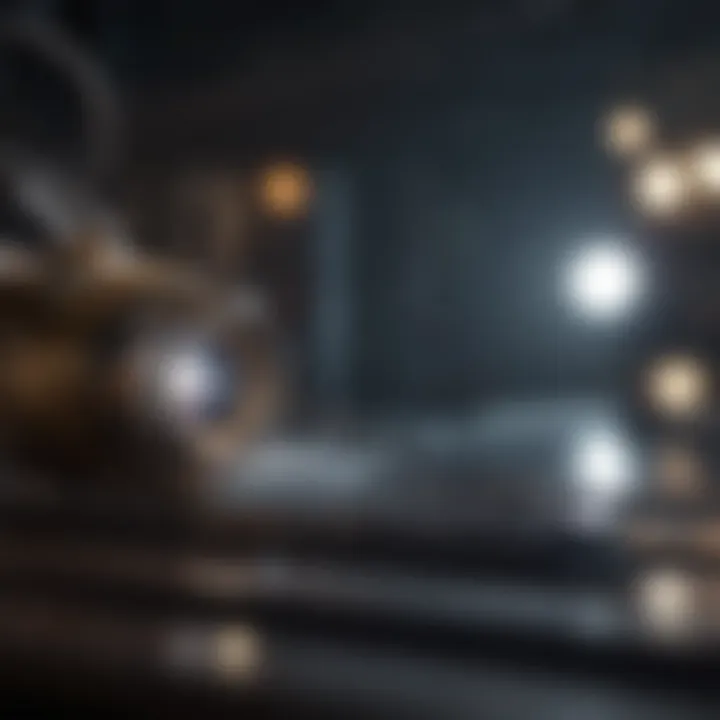
"The future of proton therapy lies in its adaptability. As we learn more about tumors and patient variability, we can refine our methods to achieve better outcomes."
While the exploration of these new directions is just beginning, it carries the promise of transforming not only how we treat cancer but also how we understand its biology, an endeavor that could have implications far beyond what is currently imagined.
With these advancements and research directions, the field of proton pencil beam technology stands at the cusp of a revolution—a revolution that carries the dual potential of providing hope to patients and opening new gateways for scientific inquiry.
Global Trends in Proton Therapy Research
The landscape of proton therapy is continually evolving, showcasing a dynamic interplay between technology, treatment methodologies, and research initiatives. As proton pencil beam therapy gains momentum in the medical community, understanding the global trends in research becomes imperative. This section dives into the significant elements of these trends, highlighting the benefits, pressing considerations, and their overall impact on the future of cancer treatment.
Increasing Accessibility
Accessibility to proton therapy remains a noteworthy factor in its expansion globally. Traditionally, such therapies have been confined to specialized institutions, limiting treatment options for many patients. However, recent studies indicate a shift toward making these therapies more widely available. This can be attributed to several factors:
- Emergence of Compact Equipment: Advancements in technology have led to the development of compact proton accelerators. These devices require less space and infrastructure, making it feasible for smaller hospitals or clinics to offer proton therapy services.
- Insurance Coverage Expansion: Growing acknowledgment of proton therapy's effectiveness has prompted insurance providers to broaden their coverage. More patients are now able to afford this advanced treatment, bridging the gap between care and accessibility.
- Telemedicine Integration: Many institutions are utilizing telehealth solutions to increase patient outreach and education, guiding potential candidates through the treatment logistics. This helps patients understand the benefits and potential of proton therapy, encouraging their engagement in clinical pathways.
"The bridge to effective treatment lies not just in technology, but in accessibility as well."
This focus on accessibility is fostering a more equitable health landscape, where more patients—regardless of geographical limitations—can benefit from advanced cancer therapies.
International Collaborative Studies
Research in proton therapy is not confined to individual countries; rather, there exists a growing trend toward international collaboration. Collaborative research offers a wide array of advantages:
- Shared Knowledge and Expertise: By pooling resources, countries can share insights and findings, thus accelerating research timelines. For instance, ongoing trials involving multiple institutions enable researchers to analyze larger patient populations, yielding more robust data.
- Harmonization of Treatment Protocols: Collaborations can help standardize treatment approaches. This is especially important in pediatric applications, where protocols may differ widely. Developing unified guidelines enhances treatment effectiveness and patient safety across borders.
- Funding Opportunities: Joint projects often attract more extensive funding from governmental and non-governmental organizations. This financial backing allows for conducting high-stakes studies that would be unfeasible for individual institutions.
As these collaborations unfold, they pave the way for innovative treatments and groundbreaking discoveries, ultimately enhancing the efficacy of proton therapy on a global scale.
Future Perspectives on Proton Pencil Beam Applications
The horizon for proton pencil beams in medicine, especially oncology, looks promising as researchers and medical professionals explore untapped applications. As the technology evolves, the importance of investigating future prospects becomes increasingly pertinent. The implications for more effective treatments and enhanced patient outcomes could be revolutionary.
Potential New Clinical Uses
While proton pencil beams currently shine in treating certain cancers, emerging research hints at potential new clinical uses that might expand their utility in broader aspects of healthcare. Areas such as neuroscience and cardiac therapies are on the table. For instance, proton therapy may be adapted for neurosurgery, whereby targeted doses could treat brain tumors while preserving surrounding healthy brain tissue. This precision could minimize side effects for patients, allowing a better quality of life post-treatment.
In addition to oncology, there’s growing interest in utilizing proton beams for treating heart conditions. With the capability of delivering radiation more accurately, there's potential for repairing damaged cardiac tissue or addressing arrhythmias through precise intervention.
The growth of personalized medicine also plays a role. As we begin to understand the unique genetic makeup of tumors, proton beams may be harnessed to target specifically identified mutations, further enhancing treatment efficacy—an approach that aligns seamlessly with current healthcare trends toward individualized therapies.
"The future of proton pencil beams isn’t just about treating cancer; it's about reshaping how we view precision medicine across various fields.”
Predicted Market Growth
As research continues to unveil the many benefits and applications of proton pencil beams, the market for proton therapy is poised for substantial growth. Analysts predict that the advancements in technology, coupled with increasing awareness and acceptance from healthcare providers, will spur demand.
Data indicates a compound annual growth rate of nearly 15% over the next decade for the proton therapy market. Factors fueling this growth include:
- Technological Innovations: Newer, more efficient accelerator designs are coming to fruition. These innovations reduce costs and enhance the feasibility of establishing treatment centers.
- Rising Cancer Incidence: As cancer rates climb globally, the need for effective treatment modalities will drive more patients towards facilities offering proton therapy.
- Government and Institutional Support: Increased investment from government bodies and healthcare institutions looking to stay on the cutting edge of treatment options will foster expansion and integration into established medical practices.
As prostate cancer, breast cancer, and even pediatric applications gain traction, we may witness proton pencil beam therapy evolving into standard practice in various treatment protocols over the coming years.
In summary, the confluence of emerging applications and market dynamics suggests that proton pencil beams are not merely a fleeting trend; they represent a substantial shift in the landscape of targeted therapies within the medical community.
End
In concluding our exploration of proton pencil beams, it becomes clear that the implications of this technology transcend mere scientific curiosity. This article shines a light on several critical factors that define the relevance and applicability of proton pencil beams in the field of medicine, particularly cancer treatment. Despite being a newer addition to the medical landscape, proton therapy using pencil beams offers a nuanced approach to targeting tumors while minimizing collateral damage to healthy tissues. This precision is indispensable, especially in cases where traditional radiation methods might pose risks of significant side effects.
Summarizing Key Insights
As we’ve dissected throughout the article, key insights emerge regarding the multifaceted role of proton pencil beams. Not only have we discussed their historical development and operational principles, but we’ve also highlighted the advantages they offer over conventional radiotherapy methods. Here are some crucial takeaways:
- Targeted Treatment: Proton pencil beams allow for precise localization of tumors, thus sparing the surrounding healthy tissues and organs.
- Adaptive Capabilities: The ability to modulate dose distribution is a game changer in radiation treatment, enabling healthcare providers to tailor therapies to individual patient needs.
- Safety for Pediatric Patients: The lower risk of long-term effects makes proton therapy particularly appealing for treating children, where the stakes are notably high.
The importance of these insights cannot be overstated; they underscore a transformative shift in oncology approaches.
Call for Continued Research
Despite the advantages, it’s paramount that the field does not remain stagnant. Continuing research in proton pencil beam technology is vital for realizing its full potential. Enhanced understanding can bolster the clinical efficacy of proton therapy. Several key areas warrant further exploration:
- Longitudinal Studies: More extensive data spanning longer periods could provide better insights into the long-term outcomes of patients treated with proton pencil beams.
- Cost-Effectiveness Analyses: Given the high costs associated with proton therapy facilities, it’s essential to conduct rigorous evaluations to determine when and how proton therapy is most beneficial economically.
- Broader Applications: Investigating the potential use of proton pencil beams outside oncology, such as in treating other medical conditions or even in veterinary practices, could lead to wider adoption and advancements.
Continued investment in research and technology will not only enhance the efficacy of proton therapy but also expand its accessibility and application.
In summary, while proton pencil beams are already making waves in the treatment landscape, the call for ongoing inquiry and innovative practices must remain a priority. Through sustained effort and collaboration, the future of this technology looks promising in enhancing patient care and redefining current treatment methodologies.