Exploring the Unique Properties of Oxide Ceramics
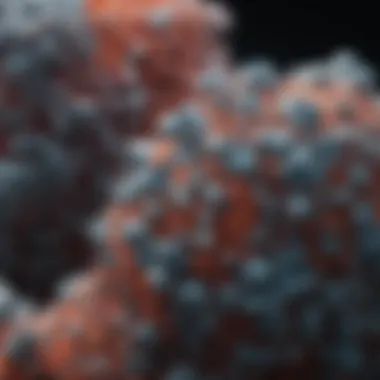
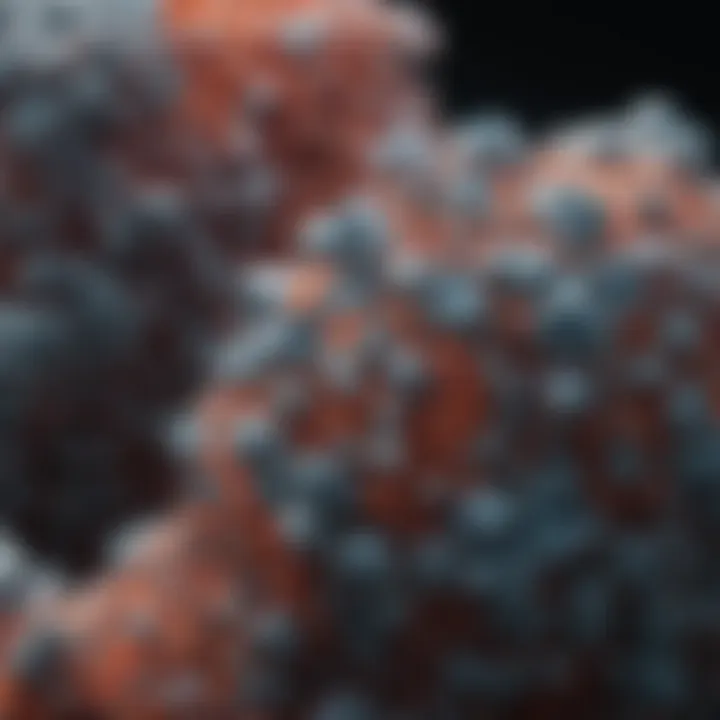
Intro
Oxide ceramics are an essential class of materials that exhibit a range of significant properties beneficial for various applications in technology and engineering. This category includes materials like alumina, zirconia, and titania, each bringing its own unique characteristics to the table. Understanding oxide ceramics is crucial for students, researchers, educators, and professionals seeking to navigate the complex landscape of modern material science.
The significance of oxide ceramics spans multiple disciplines, including electronics, structural engineering, and material sciences. These materials are valued not just for their mechanical strength and thermal stability, but also for their electrical properties. From capacitors in electronic devices to components in aerospace structures, the applications are vast.
This article aims to dissect the multiple facets of oxide ceramics, making it a comprehensive guide. The exploration includes their synthesis methods, properties, and the latest advancements in research. By delving into both theoretical frameworks and practical implications, the article seeks to illuminate the evolving role oxide ceramics play in contemporary technology.
Methodology
Overview of research methods used
Research in the area of oxide ceramics often employs a variety of methodologies to gain insights into their properties and applications. Experimental approaches include synthesis techniques such as solid-state reactions, sol-gel processes, and chemical vapor deposition. Each method has its own specific advantages and challenges.
Data collection techniques
Data collection in this field also varies based on the focus of the study. Common techniques include:
- X-ray diffraction (XRD) for phase analysis.
- Scanning electron microscopy (SEM) for morphological characterization.
- Differential thermal analysis (DTA) and thermogravimetric analysis (TGA) for thermal properties.
These methods provide vital information that feeds into understanding the structural and functional performance of oxide ceramics.
Future Directions
Upcoming trends in research
The future of research on oxide ceramics is promising, with several exciting trends emerging. Advances in nanotechnology are leading to the development of nano-oxide ceramics that exhibit superior properties compared to their bulk counterparts. Researchers are also exploring the possibilities of hybrid systems that integrate oxide ceramics with other materials to enhance performance further.
Areas requiring further investigation
While significant progress has been made, several areas need further investigation. For example:
- Investigation into the long-term stability of oxide ceramics in harsh environments.
- Understanding the influence of microstructure on the properties of oxide ceramics.
- Development of novel synthesis methods to lower production costs.
Each of these areas presents an opportunity for significant contributions to the field, potentially leading to novel applications and advancements in technology.
"The exploration of oxide ceramics represents a blend of traditional methodologies and innovative approaches that shape the future of material science."
Preface to Oxide Ceramics
Oxide ceramics play a crucial role in various technological and engineering fields. Their importance stems from unique properties such as high thermal stability, excellent dielectric characteristics, and notable mechanical strength. These attributes position oxide ceramics as essential materials in electronics, structural applications, and bioceramics. Understanding these components fosters greater insight into how they contribute to modern technology and innovation.
Definition and Overview
Oxide ceramics are defined as inorganic, non-metallic materials that are composed primarily of oxides. Common compositions include aluminum oxide, zirconium oxide, and titanium oxide. These materials are typically characterized by their hardness and resistance to wear, making them ideal for a host of applications. In many cases, oxide ceramics are synthetized through various production methods, allowing for considerable control over their microstructure and properties. The versatility of oxide ceramics leads to applications ranging from insulators in electronics to structural components in engineering.
Historical Context
The historical trajectory of oxide ceramics dates back thousands of years. Ancient civilizations, such as the Chinese and Greeks, utilized clay and other oxide-rich materials for pottery and construction. The modern era witnesses a significant evolution in applications, particularly during the industrial revolution when advancements in material science catalyzed the development of high-performance ceramics. As the demand for advanced materials has surged in contemporary technology, oxide ceramics have seen a marked increase in utilization across multiple sectors. This historical context is vital for understanding the present-day relevance and future directions of oxide ceramics in various applications.
Chemical Composition of Oxide Ceramics
Understanding the chemical composition of oxide ceramics is crucial for several reasons. The specific elements within these materials dictate their physical properties, performance in various applications, and overall durability. From electronic devices to structural components, the attributes derived from chemical composition have profound implications. This section will break down the main oxide components that form oxide ceramics and discuss the effects of impurities.
Main Oxide Components
The foundation of oxide ceramics lies in their primary oxide constituents. Key components typically include:
- Alumina (Al2O3): Widely recognized for its excellent hardness and thermal stability, alumina is often utilized in structural applications as well as in insulators.
- Zirconia (ZrO2): Known for its toughness and thermal insulation properties, zirconia undergoes phase transformations that enhance its mechanical strength, making it valuable for various uses.
- Silica (SiO2): Commonly used in glass and ceramics, silica contributes to the overall chemical durability and structural integrity of oxide ceramics.
These components interact in complex ways, influencing the microstructure and consequently the standing performance of the ceramics. The careful selection and balance of these oxides can help meet specific engineering and scientific requirements.
Impurity Effects
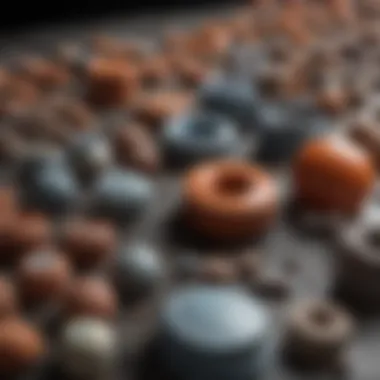
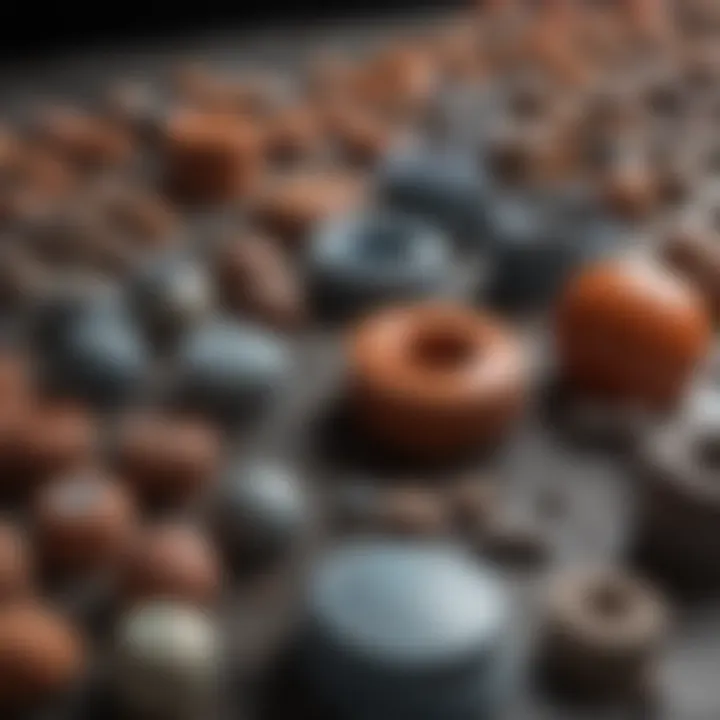
The presence of impurities in oxide ceramics is a double-edged sword. While certain impurities can enhance properties, others may lead to potential degradation.
- Positive Impacts: Some impurities can improve the mechanical properties. For example, small quantities of magnesium oxide (MgO) can stabilize certain phases in zirconia, leading to increased toughness.
- Negative Impacts: Conversely, contaminants can induce undesirable effects such as cracking or thermal shock susceptibility. The incorporation of iron oxides, for instance, can affect the color and electron transport mechanisms, possibly rendering the ceramics less conductive.
Overall, the influence of impurities highlights the importance of maintaining strict quality control during the synthesis of oxide ceramics. A thorough understanding of both the desired oxide components and the potential effects of impurities significantly enhances the material's usability in advanced applications.
"Chemical composition is not just about gathering materials; it is about engineering a precise balance that meets functional demands in varying environments."
By focusing on both main components and the implications of impurities, one can appreciate the intricate relationship within oxide ceramics that drives their performance in countless applications.
Physical Properties of Oxide Ceramics
Understanding the physical properties of oxide ceramics is essential, as these characteristics greatly influence their applications and functionalities. Oxide ceramics are known for their robustness in various demanding environments. This section will elucidate critical aspects, such as mechanical strength, thermal stability, and electrical conductivity, providing a comprehensive overview relevant to researchers, educators, and industry professionals.
Mechanical Strength
Mechanical strength is one of the hallmark features of oxide ceramics. This property pertains to the material's ability to withstand mechanical stresses without fracturing. Oxide ceramics, such as alumina (Al2O3) and zirconia (ZrO2), exhibit high hardness and compressive strength, making them suitable for applications in structural components and cutting tools.
The strength of these ceramics often depends on their microstructure, including grain size and porosity. A finer grain size typically results in improved mechanical properties. Importantly, the brittleness of oxide ceramics should not be overlooked. Unlike metals, which can deform, ceramics often fail by cracking, which necessitates careful consideration during design and application.
Thermal Stability
Thermal stability is another critical physical property of oxide ceramics. It indicates the material's ability to maintain structural integrity and performance at elevated temperatures. Many oxide ceramics can endure extreme temperatures, making them ideal for applications in environments where high thermal resistance is crucial, such as aerospace components and kiln linings.
One key factor in thermal stability is the phase behavior of ceramics. For instance, zirconia can undergo phase transformations at high temperatures, affecting its mechanical performance. Therefore, enhanced thermal stability can be achieved by adding stabilizers, such as yttria, to improve durability.
Electrical Conductivity
Electrical conductivity in oxide ceramics can vary widely, from insulators to semiconductors. For instance, materials like alumina are excellent insulators, whereas others, like tin oxide (SnO2), show notable semiconductor properties. Understanding the electrical behavior of these ceramics is vital in electronics and sensor applications.
The ability to manipulate dopants allows for tuning the conductivity of oxide ceramics. Factors such as temperature and atmosphere also play significant roles in determining conductivity. In electronic applications, high electrical resistivity is often desired, whereas in sensing applications, moderate conductivity may be beneficial.
In summary, the physical properties of oxide ceramics—mechanical strength, thermal stability, and electrical conductivity—are interlinked and significantly impact their functionality in real-world applications. The optimization of these properties is crucial for developing advanced materials that meet the challenges of modern engineering.
Synthesis and Fabrication Techniques
The synthesis and fabrication of oxide ceramics play a crucial role in determining their properties and usability. Understanding these methods is essential for researchers and engineers focused on optimizing material performance for various applications. This section elaborates on the prominent techniques used for synthesizing oxide ceramics, each presenting unique advantages and challenges.
Solid-State Synthesis
Solid-state synthesis is one of the most traditional methods for producing oxide ceramics. In this technique, fine powders of the desired oxides are mixed together, followed by a series of heat treatments. The process involves high temperatures that facilitate the reaction between the components, leading to the formation of the final ceramic phase.
- Advantages:
- Challenges:
- Cost-effective: This method usually requires less specialized equipment, making it accessible for many laboratories.
- Scalability: It is suitable for large-scale production due to its relatively straightforward process.
- Homogeneity: Achieving a uniform chemical composition can be difficult, which may lead to variations in properties.
- Particle Size Control: The final product's performance can be influenced by the initial particle sizes of the reactants.
The solid-state method remains a mainstay in the fabrication of oxide ceramics, especially in the production of ceramics used in structural applications and insulators.
Sol-Gel Process
The sol-gel process is a versatile technique that enables the controlled synthesis of oxide ceramics at lower temperatures compared to solid-state synthesis. This method involves the transition of a solution (sol) into a solid (gel) phase, allowing for the incorporation of various dopants or compounds at the molecular level.
- Advantages:
- Challenges:
- Fine Microstructure: The sol-gel process can produce materials with a high surface area and fine microstructure, enhancing their mechanical properties.
- Uniform Distribution: It allows for a thorough mixing at the molecular level, which is essential for uniformly doped ceramics.
- Processing Time: The gel formation and drying processes can be time-consuming, affecting overall production efficiency.
- Handling of Precursors: The chemical precursors used may require careful handling due to potential toxicity.
Because of its versatility, the sol-gel process is widely used for creating functional coatings and nanostructured ceramics.
Chemical Vapor Deposition
Chemical vapor deposition (CVD) is an advanced technique used to synthesize high-purity oxide ceramics. In this process, gaseous reactants interact on a substrate to form a solid oxide layer or component. CVD can be tailored for various material requirements, responding to the needs of modern applications.
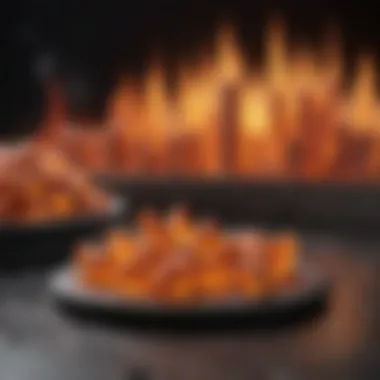
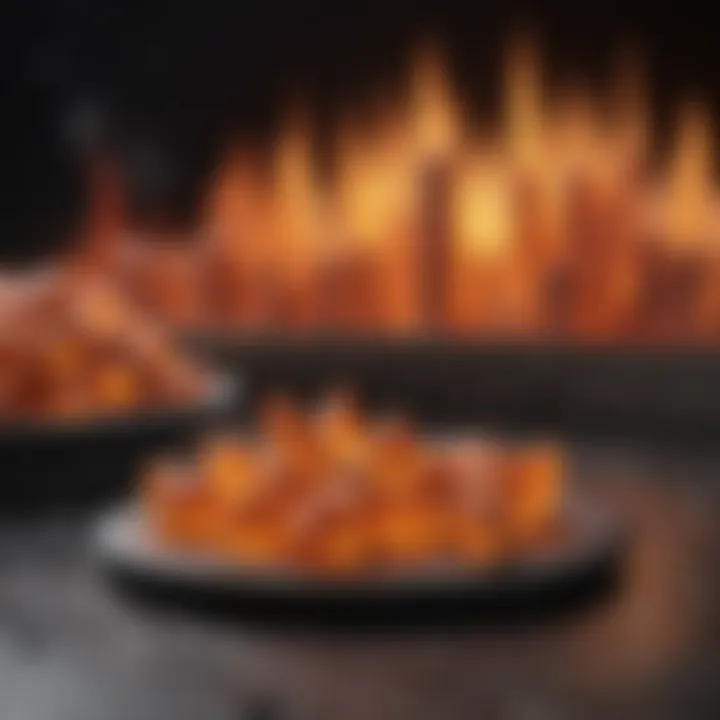
- Advantages:
- Challenges:
- High Purity Layers: This method can produce layers with exceptional purity and adhesion, critical for electronic applications.
- Control Over Composition: Precise control over the composition allows for the creation of complex oxide ceramics tailored for specific functionalities.
- Equipment Cost: The CVD process requires specialized equipment, which may elevate production costs.
- Complex Process: The operational parameters need to be carefully monitored and controlled for successful results, making it more complex to optimize.
CVD continues to gain traction in advanced technology sectors, especially in producing materials for semiconductors and various electronic devices.
In summary, understanding the synthesis and fabrication techniques of oxide ceramics is fundamental to tailoring their properties for diverse applications. Each method presents its own sets of benefits and challenges, warranting careful consideration based on the intended application and desired material characteristics.
Characterization Methods for Oxide Ceramics
Characterization methods are essential in understanding the properties and structures of oxide ceramics. The effectiveness of these materials in their applications often depends on their inherent features, which can be effectively assessed through precise analytical techniques. By using various characterization methods, researchers can gain insights into the material's crystallinity, surface morphology, and compositional analysis, aiding in the optimization of synthesis methods and the development of novel applications. The significance of this area of study cannot be overstated, as it directly influences the performance and reliability of oxide ceramics in diverse fields such as electronics and engineering.
X-Ray Diffraction Analysis
X-Ray Diffraction (XRD) is a crucial technique used for characterizing the crystalline structure of oxide ceramics. The method works by directing X-rays onto a sample and measuring the resulting diffraction patterns. This provides detailed information about the lattice parameters and phase identification of the material.
A clear advantage of XRD is its ability to ascertain phase purity and detect any secondary phases that may be present due to impurities or incomplete reactions during synthesis. Analysis from XRD can yield insights about the preferred orientation of crystallites, which is vital for optimizing the mechanical properties of ceramics. Furthermore, the quantification of crystalline size can inform researchers about the potential performance of ceramics under various conditions.
"XRD is instrumental in revealing the underlying structural characteristics of oxide ceramics, thus paving the way for specific application environments."
XRD is widely utilized because it is a non-destructive method and can apply to a broad range of ceramics. Its versatility is beneficial for both fundamental research and industrial applications.
Scanning Electron Microscopy
Scanning Electron Microscopy (SEM) serves as another fundamental technique in the characterization of oxide ceramics. SEM leverages focused beams of electrons to generate high-resolution images of a material's surface. This method allows researchers to analyze microstructural details such as grain size, surface morphology, and fracture mechanisms.
The capability of SEM to provide three-dimensional representations at a microscopic level is a notable strength. It enables the observation of surface defects, which can significantly influence the mechanical properties of ceramics. This information is crucial for ensuring the durability and reliability of oxide ceramic components in practical applications.
Additionally, SEM can often be paired with Energy Dispersive X-ray Spectroscopy (EDS), allowing for the determination of the elemental composition of the surface. This synergy gives comprehensive insight into the correlation between microstructural features and chemical composition.
Transmission Electron Microscopy
Transmission Electron Microscopy (TEM) is an advanced characterization method that provides even greater detail compared to SEM. This technique involves transmitting electrons through a thin sample, allowing for the visualization of internal structures at atomic resolution. TEM can elucidate information about lattice defects, dislocations, and phase boundaries in oxide ceramics.
The high-resolution images produced by TEM are invaluable for studying phenomena such as phase transformations and crystallographic relationships in materials. Furthermore, it helps in understanding how these features affect overall material properties and functionalities.
One of the primary challenges with TEM is that samples must be extremely thin, which can complicate sample preparation. However, the depth of structural insight gained often justifies these challenges, especially when working with nanostructured or complex oxide ceramic systems.
Applications of Oxide Ceramics
Oxide ceramics are critical materials widely used across various industries due to their distinctive properties. Their applications span electronics, structural engineering, and biomedical fields. Understanding these applications reveals the significance of oxide ceramics in technological advancements and everyday products. This section delves into three primary areas where oxide ceramics play a crucial role: electronics and insulators, structural applications, and bioceramics.
Electronics and Insulators
In the realm of electronics, oxide ceramics are indispensable. They exhibit excellent dielectric properties, making them ideal for capacitors, insulators, and substrates in electronic devices. Materials like barium titanate and alumina are commonly used due to their high dielectric strength and low electrical conductivity. These characteristics are essential in ensuring the reliability of electronic components, as they minimize energy loss and enhance performance.
Furthermore, oxide ceramics are often utilized in high-frequency applications, such as microwave devices, due to their stability and endurance under various temperatures. This reliability is crucial for components in communications, aerospace, and automotive industries. The demand for lightweight and miniaturized devices has led to a push for innovative oxide ceramic materials that can maintain performance while reducing size.
Structural Applications
Oxide ceramics demonstrate exceptional mechanical strength and hardness, making them suitable for structural applications. They are increasingly used in building materials, aerospace components, and automotive parts. For example, zirconia is favored for its toughness and wear resistance, making it a viable choice for cutting tools and engine components.
The ability to withstand extreme environmental conditions also adds to their appeal. Oxide ceramics can resist corrosion, oxidation, and thermal shocks, enhancing the durability of structures and machinery. As industries evolve, the focus on lightweight yet strong materials has increased, pushing oxide ceramics to the forefront of structural innovation.
Bioceramics
Bioceramics represent a specialized application of oxide ceramics, primarily in the medical field. These materials are biocompatible and can be integrated into the human body without causing adverse reactions. Hydroxyapatite and bioactive glass are examples of bioceramics used in dental implants and orthopedic applications.
The benefits of using bioceramics include the ability to promote bone ingrowth and support tissue regeneration. This functionality makes them highly effective for surgeries involving bone defects and dental replacements. The growing demand for advanced medical materials places bioceramics in a pivotal position for future research and development in regenerative medicine.
"The unique properties of oxide ceramics make them vital in a wide range of applications, influencing industries from electronics to biomedicine."
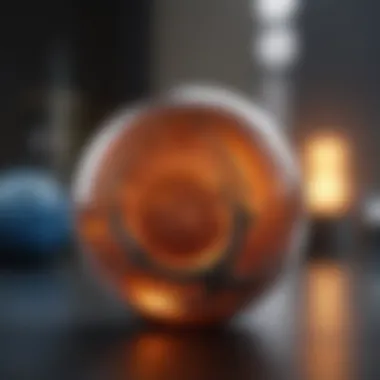
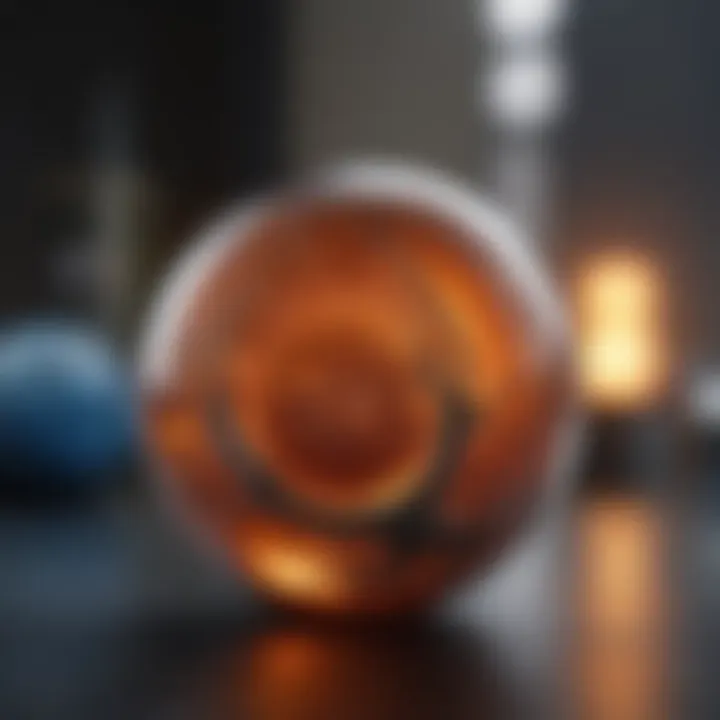
Environmental Impact of Oxide Ceramics
The environmental impact of oxide ceramics is an important subject to consider. As industries increasingly rely on these materials, understanding how they affect the environment becomes crucial. Oxide ceramics are known for their stability and durability, but their production and eventual disposal raise several environmental questions. In recent years, the focus has shifted towards developing more sustainable practices in the manufacturing and use of these materials.
Recycling Challenges
Recycling oxide ceramics presents distinct challenges. One critical issue arises from the strong bonding nature of ceramic materials. Their tightly packed atomic structure makes it hard to break them down into their original components. When ceramics are broken, they often do not melt; instead, they shatter into small, unusable pieces.
- Limited methods: Conventional recycling processes like those used for metals or plastics do not effectively apply to ceramics. This leads to an accumulation of ceramic waste in landfills, which is problematic given their long lifespan.
- Contaminants: In addition, recycled ceramics may contain additives or impurities that complicate the recycling process. This raises concerns regarding the quality and purity of recycled end-products.
Being aware of these challenges is essential in addressing the sustainability of oxide ceramics.
Sustainability Practices
To navigate the environmental concerns associated with oxide ceramics, researchers and manufacturers are exploring various sustainability practices. The goal is to reduce waste and enhance the life cycle of ceramic materials.
- Alternative materials: One approach involves the use of alternative raw materials that are more environmentally friendly. For example, using naturally occurring minerals can reduce the carbon footprint compared to processed materials.
- Energy-efficient processes: Developing energy-efficient manufacturing processes also plays a role. Techniques that require lower temperatures or less energy in the production phase can minimize environmental damage.
Implementing such practices not only supports environmental conservation but also aligns with global efforts to promote sustainability in materials science.
"The focus on sustainability in oxide ceramics aims to harmonize usage without compromising the health of our planet."
Recent Advances in Oxide Ceramics Research
Recent advances in oxide ceramics research signify a crucial turning point in the field of materials science and engineering. These developments are expanding the applications of oxide ceramics in various industries, impacting everything from electronics to biomedicine. Innovations in synthesis techniques, characterization methods, and understanding of material properties have led to significant improvements in performance and functionality. This section highlights two major areas of progress: nanostructured ceramics and functional coatings, exploring their implications and benefits.
Nanostructured Ceramics
Nanostructured ceramics represent a promising frontier in oxide ceramic research. At the nanoscale, materials exhibit unique properties that are not visible at the macro level. These properties often include enhanced mechanical strength, improved thermal stability, and increased electrical conductivity. The reduction in grain size contributes to these enhancements. By employing methods such as sol-gel processing and hydrothermal synthesis, researchers can create ceramics with controlled nanostructures.
The benefits of nanostructured ceramics are particularly evident in electronic applications, where miniaturization is crucial. For instance, they can be used in capacitors and resistors that require high dielectric strength. By optimizing the nanostructuring process, manufacturers can produce components with superior performance. Additionally, nanostructured ceramics are desirable in bioceramics due to their increased surface area which promotes cell attachment and growth, making them ideal for medical implants.
Functional Coatings
Functional coatings have emerged as another area of significant interest in oxide ceramics research. These coatings involve applying thin layers of oxide ceramic materials on various substrates. This approach enhances the properties of the underlying material while imparting new functionalities. Functional coatings can provide benefits such as corrosion resistance, thermal barrier protection, and wear resistance.
Applications of functional coatings vary widely, from aerospace to automotive industries. For example, thermal barrier coatings made from zirconia-based ceramics can improve the efficiency of gas turbines by protecting components from extreme heat. The development of these coatings also emphasizes the need for precise deposition techniques, such as chemical vapor deposition (CVD) and plasma spraying. These techniques allow for better control over the coating characteristics, improving the reliability and performance of the final product.
Advances in the understanding of oxide ceramics at the nanoscale and through functional coatings underscore their importance in modern technology.
Future Prospects of Oxide Ceramics
The future of oxide ceramics seems promising, marked by continual growth and development in various sectors. Their inherent properties, such as high thermal stability and electrical insulation, make them ideal for futuristic applications. As industries seek materials that can withstand extreme environments and perform reliably, oxide ceramics will play a crucial role. Understanding the elements driving these advancements is essential for stakeholders, from policymakers to researchers and engineers.
Emerging Technologies
Advancements in technology have catalyzed new uses for oxide ceramics. One notable innovation is in the realm of energy storage and conversion. For example, ceramics that are ion conductive are being integrated into solid-state batteries.
Another area of interest is biomedical applications. Oxide ceramics, such as zirconia, are being explored for their biocompatibility, making them suitable for implants and prosthetic devices. The combination of these materials’ properties enhances their performance in biomedical environments.
A key factor in the growth of oxide ceramics will incorporate nanotechnology. Developing nanostructured oxide ceramics may lead to improved performance in various applications. These materials can potentially exhibit unique characteristics when engineered at a nanoscale, thus expanding their utility.
"The adaptability of oxide ceramics allows them to meet the challenges of tomorrow's technological demands."
Market Trends
The market for oxide ceramics is influenced by multiple trends. First, the demand in renewable energy solutions is on the rise. Oxide ceramics are being used in photovoltaic cells and fuel cells, making them integral to sustainable energy initiatives.
Second, the electronics sector is expanding. Oxide ceramics are crucial in the production of semiconductors and insulators, supporting the growing technology ecosystem. As electronic devices become ubiquitous, the need for reliable materials like oxide ceramics will only increase.
Moreover, global competition is pushing companies to innovate continuously. This results in more research and development focused on oxide ceramics, further enhancing their properties and applications. Companies investing in these materials are likely to gain competitive advantages, driving market growth.
End
The significance of the conclusion in this article is multi-faceted. Firstly, it serves as a synthesis of the extensive discussions about oxide ceramics, wrapping up the insights gained throughout various sections. The conclusion reiterates the importance of oxide ceramics in contemporary material science, particularly in their applications across electronics, structural engineering, and biomedical fields. By summarizing the key aspects explored, it reinforces the relevance of understanding the chemical composition, synthesis methods, and environmental impacts associated with these materials.
Moreover, it highlights the potential for future research and innovation in oxide ceramics. This is crucial as advancements in technologies can lead to the development of novel applications that we may not yet fully appreciate. Therefore, the conclusion not only encapsulates the existing knowledge but also encourages further inquiry and exploration.
Additionally, considerations regarding the sustainability of oxide ceramics are prominent. With industries increasingly facing pressure to maintain eco-friendly practices, the conclusion emphasizes the need for recycling and sustainable production methods, which are integral to the future landscape of materials science.
"In an era of rapid technological advancement, oxide ceramics emerge as pivotal materials that bridge traditional methods and innovative applications."
Key Takeaways
- Oxide ceramics are essential materials with significant roles in various industries including electronics and biomedicine.
- The understanding of their chemical and mechanical properties is critical for their effective application.
- Synthesis techniques vary and influence the final product characteristics, necessitating careful selection based on desired outcomes.
- Environmental impact and sustainability practices are increasingly important in the development and use of oxide ceramics.
- Future trends indicate a move towards advanced applications and technologies involving oxide ceramics, indicating a need for ongoing research and development.