Exploring Carbon Capture from Flue Gas Mechanisms and Technologies
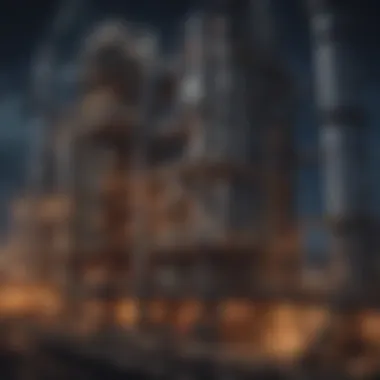
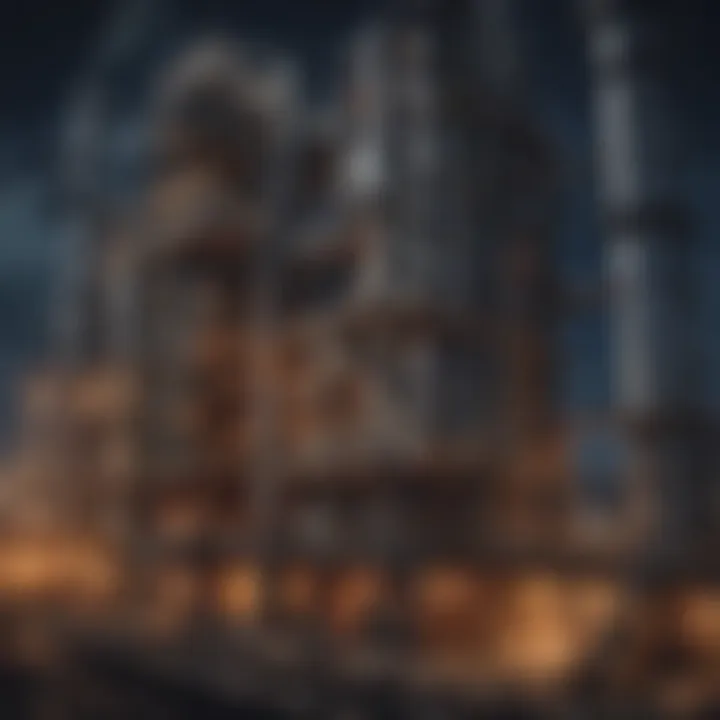
Intro
Carbon capture plays a crucial role in addressing climate change. With increasing global temperatures, the need to reduce carbon dioxide emissions has never been more urgent. Flue gas, produced by industrial and power plants during combustion processes, is a significant source of CO2 emissions. Understanding how to capture carbon from flue gas is vital for large-scale implementation of this technology.
This article will explore the mechanisms and technologies associated with carbon capture from flue gas. It will focus on their effectiveness, operational challenges, and broader implications for climate policy and sustainability. By examining this subject, we aim to underline its importance in mitigating the adverse effects of greenhouse gases on the environment.
Methodology
Overview of research methods used
To understand carbon capture technologies comprehensively, a combination of qualitative and quantitative research methods were employed. Literature reviews were conducted to gather existing data on various technologies, case studies, and empirical research results. This provides a foundational understanding of current practices and innovations. Interviews and discussions with experts in the field also contribute valuable perspectives on operational challenges and advancements in the industry.
Data collection techniques
Data collection involved several techniques, including:
- Document analysis: Reviewing scientific journals, technical reports, and industry publications helped establish a baseline of knowledge.
- Case studies: Examination of successful carbon capture projects showcased practical applications and effectiveness of specific technologies.
- Expert interviews: Engaging with professionals in the sector facilitated insight into the latest trends and potential future developments.
This mix of methods ensured a well-rounded exploration of carbon capture from flue gas, allowing us to assess not just technological aspects but also their real-world implications.
Technologies in Carbon Capture
While several technologies exist for carbon capture, the most prominent include:
- Post-combustion capture: This method captures CO2 from flue gases after combustion. Common technologies in this category include amine scrubbing and solvent-based systems.
- Pre-combustion capture: In this approach, fuel is treated before combustion, which helps separate hydrogen and CO2 effectively. Technologies such as gasification and reforming are typically used.
- Oxy-fuel combustion: This method uses pure oxygen instead of air, resulting in a flue gas that consists mainly of CO2 and water vapour. This simplifies the capture process.
Each technology has its unique mechanisms, advantages, and challenges. Factors like energy requirements, cost, and scalability influence their deployment in industrial settings.
Future Directions
Upcoming trends in research
Research into carbon capture technologies is rapidly evolving. Some key trends include:
- Innovative materials: Development of new absorbents and membranes to enhance capture efficiency and reduce costs.
- Integration with renewable energy: Combining carbon capture with renewable energy sources to minimize emissions effectively.
- Direct air capture technology: Investing in technologies that capture CO2 directly from the atmosphere, opening new avenues for removal of greenhouse gases.
Areas requiring further investigation
There are still knowledge gaps and challenges that warrant further exploration, such as:
- Long-term storage solutions: Finding safe and effective means to store captured CO2 to prevent it from re-entering the atmosphere.
- Lifecycle analysis: Understanding the overall environmental impact of various carbon capture technologies, ensuring they provide genuine benefits.
- Regulatory frameworks: Establishing guidelines that encourage investment and development of carbon capture technologies while ensuring public safety and environmental protection.
By addressing these areas, the field of carbon capture can advance effectively, making significant strides in fighting climate change.
Prolusion to Carbon Capture
Carbon capture is an essential component of strategies aimed at reducing greenhouse gas emissions. As industries continue to expand, so does the urgency to find effective methods to manage and mitigate the carbon dioxide they release into the atmosphere. This section will outline the basic understanding of carbon capture and its critical function in climate change efforts.
Definition of Carbon Capture
Carbon capture refers to the process of capturing carbon dioxide emissions produced from the use of fossil fuels in electricity generation and various industrial processes. Through these technologies, carbon dioxide is collected before it can enter the atmosphere, where it typically contributes to global warming. The captured carbon dioxide can then be stored underground or used in various industrial applications.
The uptake of carbon capture technologies is vital for achieving climate targets set by global agreements. Various methods are employed to capture carbon dioxide, each with its own advantages and challenges. For instance, chemical absorption and membrane separation are common techniques, and understanding these can help in selecting the best approach for specific scenarios.
Importance in Climate Change Mitigation
The importance of carbon capture in climate change mitigation cannot be understated. First, it allows for continued economic growth and energy production while addressing environmental concerns. By integrating carbon capture systems into existing infrastructure, industries can minimize their carbon footprints, leading to significant reductions in overall emissions.
Moreover, carbon capture supports the transition towards a circular economy. Rather than treating carbon dioxide simply as waste, utilizing captured CO2 can stimulate innovation and new business models. Businesses can explore avenues such as enhanced oil recovery or the production of synthetic fuels.
"Carbon capture is not a standalone solution, but part of a broader strategy that involves energy efficiency and renewable energy."
Flue Gas Composition and Sources
Understanding the composition and sources of flue gas is crucial to appreciating the challenges faced in carbon capture and the overall effectiveness of the technologies available. Flue gas, generated by combustion processes, is a complex mixture of various gases and particulate matter. By dissecting its components, one can identify not only the potential pollutant elements but also the underlying opportunities for effective carbon capture.
Definition and Sources of Flue Gas
Flue gas is a byproduct emitted from the combustion of fossil fuels or other organic materials in industrial processes, power plants, and residential heating systems. It typically results from the burning of coal, natural gas, oil, or biomass. Facilities like coal-fired power plants, cement manufacturing plants, and waste-to-energy plants are common sources of flue gas emissions. These gases must be carefully managed due to environmental regulations and growing concerns regarding climate change.
Key Components of Flue Gas
The primary constituents of flue gas include nitrogen, carbon dioxide, oxygen, and water vapor. However, it may also contain trace levels of carbon monoxide, sulfur dioxide, nitrogen oxides, and particulate matter. Each of these components contributes to the environmental impact of flue gas. Understanding their proportions is essential for optimizing carbon capture techniques.
- Carbon Dioxide (CO2): The dominant greenhouse gas in flue gas, accounting for a significant portion of the emissions.
- Nitrogen (N2): Usually makes up the bulk of flue gas due to the abundance of nitrogen in the atmosphere.
- Water Vapor (O): Important for thermodynamic processes associated with capture technologies.
- Sulfur Dioxide (SO2) and Nitrogen Oxides (NOx): These pollutants can adversely affect air quality and contribute to acid rain.
Role of Carbon Dioxide in Flue Gas
Carbon dioxide plays a critical role in the context of flue gas as its concentration serves as a primary target for capture technologies. With the increasing recognition of CO2's contribution to global warming, the focus has shifted toward reducing its emission at the source. The carbon dioxide in flue gas can originate from the combustion of carbon-rich fuels, making it integral to coal and natural gas power plants.
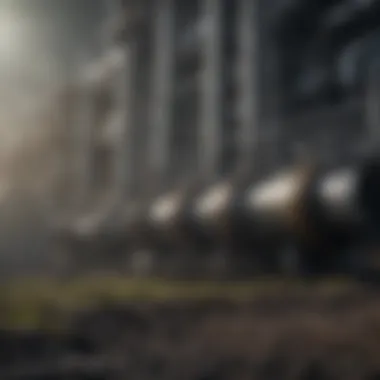
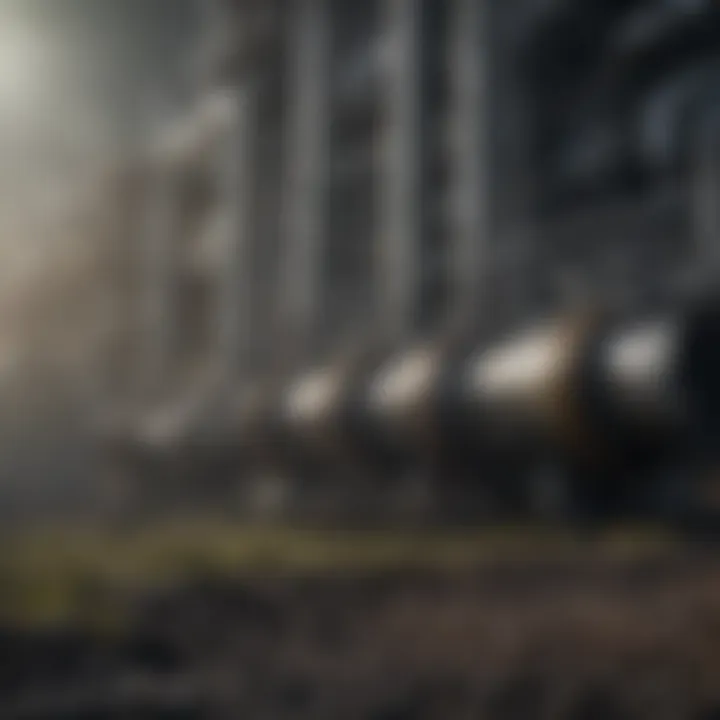
Capturing CO2 from flue gas not only aids in lowering atmospheric concentrations but also provides an opportunity to sequester it for practical applications, such as enhanced oil recovery, or to convert it into usable products. Future strategies in carbon capture must consider the specific composition of flue gas while aiming for reductions in both CO2 and other harmful pollutants.
"The ability to effectively capture CO2 from flue gas will significantly influence global climate strategies and industry standards in the years to come."
To summarize, the understanding of flue gas composition and its sources is foundational for advancing carbon capture technologies. Identifying what comprises flue gas allows for targeted approaches in mitigation efforts that can enhance environmental sustainability.
Technologies for Carbon Capture
Technologies for carbon capture play a crucial role in the larger context of mitigating climate change. The essence of these technologies lies in their ability to reduce carbon dioxide emissions from flue gas generated during industrial processes. This section will explore various approaches to carbon capture, including absorption, adsorption, membrane separation, and cryogenic distillation. Each method has its own attributes, advantages, and potential drawbacks. Understanding these technologies helps identify the most efficient and effective methods.
Absorption Techniques
Absorption techniques are among the most common methods of capturing carbon dioxide from flue gas. The basic principle involves a solvent that selectively absorbs the carbon dioxide, allowing it to be removed from the gas stream.
Chemical Absorption
Chemical absorption is widely recognized for its effectiveness in capturing carbon dioxide. In this technique, a chemical reaction occurs between the absorbing solvent and carbon dioxide. One key characteristic of chemical absorption is the use of amine solutions, which react with CO2 to form unstable carbamate compounds.
This method is popular because it provides high efficiency at low concentrations of carbon dioxide, making it ideal for flue gas applications. The unique feature of chemical absorption lies in its high selectivity toward CO2, which allows for the separation of carbon dioxide from other gas components. However, the disadvantages include the energy requirements for regenerating the solvent and potential degradation over time.
Physical Absorption
Physical absorption differs from chemical absorption in that it relies on physical interactions rather than chemical reactions. This process involves the dissolution of carbon dioxide in a solvent under high pressure. A significant advantage of physical absorption is its lower energy consumption compared to chemical absorption. It is effective for capturing larger volumes of CO2 at high pressures.
However, physical absorption can be less effective at low concentrations, making it less suitable for raw flue gas streams that might have a low CO2 content. Additionally, the choice of solvent can impact the overall effectiveness.
Adsorption Technologies
Adsorption technologies utilize solid materials to capture carbon dioxide from gas streams. This approach takes advantage of surface interactions between the adsorbent and the gas molecules, promoting a more efficient separation process.
Zeolites
Zeolites are a class of microporous materials known for their ability to selectively adsorb larger molecules. They have a key characteristic of offering a high surface area, which makes them effective for carbon dioxide adsorption when integrated into carbon capture systems. Zeolites can selectively capture CO2 due to their tunable pore sizes.
The unique feature of zeolites is their regenerative capacity, allowing them to be reused multiple times after regeneration with heat or pressure reduction. However, there can be limitations in their performance under varying humidity conditions, which may affect their effectiveness in industrial applications.
Activated Carbon
Activated carbon is another prominent material used in adsorption technologies. Its high porosity and surface area facilitate efficient CO2 adsorption. One of the main advantages of activated carbon is its flexibility in design, which can be tailored to specific flue gas compositions.
A distinct characteristic is the economic feasibility of using activated carbon, compared to other more specialized adsorbents. However, activated carbon can become saturated relatively quickly, requiring periodic replacement or regeneration, which can impact overall efficiency.
Membrane Separation
Membrane separation is a technology that utilizes selective barriers to allow only certain gas molecules to pass through. This method is becoming increasingly relevant in carbon capture due to its lower energy demands.
Membranes can be designed to preferentially allow carbon dioxide to permeate while blocking other gases, which can help in effective separation. The main advantage is that membrane systems can be compact and require lower operational costs than traditional absorption methods. However, there may be challenges in achieving high selectivity and permeability concurrently, which must be overcome for enhanced performance.
Cryogenic Distillation
Cryogenic distillation is a separation technique that involves cooling gas streams to very low temperatures, allowing it to be condensed. When the temperature is lowered, carbon dioxide can be easily separated from other gases due to differences in boiling points.
This technology offers the benefit of high purity for the captured carbon dioxide, suitable for various applications including enhanced oil recovery. The main consideration is the significant energy requirement to achieve the low temperatures needed for effective separation, which can pose challenges in terms of overall sustainability.
Overall, the exploration of various technologies for carbon capture offers substantial insight into effective methods of reducing carbon emissions. Each technology presents its own blend of benefits and challenges, contributing to the ongoing efforts to combat climate change.
Mechanisms of Capture
Understanding the mechanisms of capture is fundamental to the success of carbon capture technologies. These mechanisms dictate how carbon dioxide can be effectively separated from flue gas, allowing for its safe storage or use. The various interactions involved in the capturing processes profoundly affect the overall efficiency and practicality of carbon capture systems.
Fundamentals of Physical and Chemical Interactions
Carbon capture relies heavily on both physical and chemical interactions to separate carbon dioxide from other gaseous substances in flue gas. Physical interactions mainly involve processes such as adsorption and absorption. In adsorption, molecules of carbon dioxide adhere to the surface of solid materials. This can take place in zeolites or activated carbon, where the porous nature of the material increases surface interaction, optimizing the capture process.
On the other hand, chemical interactions are critical in processes like chemical absorption. Here, carbon dioxide chemically reacts with a solvent, such as amines, leading to the formation of a new compound. This method is highly effective, as it can selectively capture CO2 even at low concentrations. However, it often requires more energy to regenerate the solvent for reuse.
Both interaction types demonstrate distinct advantages. The physical processes may be simpler and less energy-intensive, whereas chemical processes can achieve higher capture rates, albeit with higher operational costs and energy demands. Understanding these interactions guides the selection of suitable capture technologies for specific industrial applications.
Kinetics and Thermodynamics in Capture Processes
Kinetics and thermodynamics play crucial roles in the efficiency of carbon capture mechanisms. Kinetics refers to the rate at which carbon dioxide is absorbed or adsorbed, influenced by factors like temperature, pressure, and the properties of the capturing agent. For instance, higher temperatures can increase the kinetic energy of molecules, which might enhance the capture rate for certain physical absorption processes.
Thermodynamics, meanwhile, examines the energy changes and the feasibility of the capture processes. Each carbon capture method has an associated energy profile. For example, chemical absorption is generally an exothermic reaction, meaning it releases heat, which could help in enhancing the absorption capacity under specific conditions.
"A deep understanding of kinetics and thermodynamics is essential for optimizing carbon capture technologies and achieving significant reductions in greenhouse gas emissions."
When evaluating capture processes, it is vital to consider both kinetics and thermodynamics. This ensures not only that CO2 is captured effectively but also that the processes employed are economically viable. Continuous research is needed to develop more efficient capture methods that leverage these principles, driving the advancement of carbon capture technologies.
Effectiveness and Efficiency of Carbon Capture
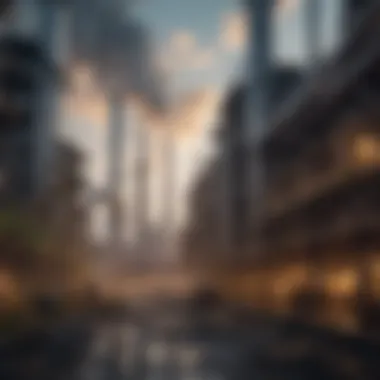
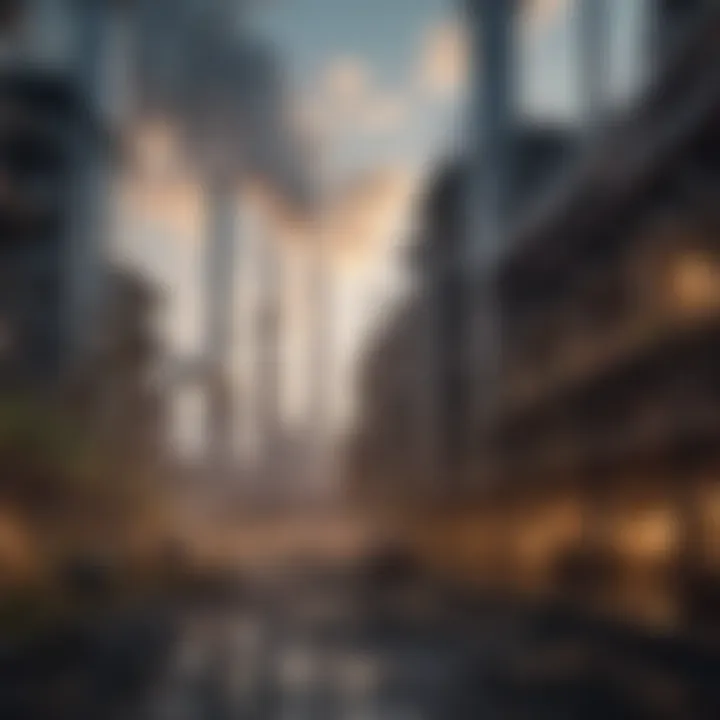
The success of carbon capture technologies lies not only in their ability to extract carbon dioxide from flue gas but also in their effectiveness and efficiency. This section examines both aspects, providing insights into how various factors and performance metrics impact the overall success of these systems.
Factors Affecting Capture Efficiency
Several factors influence the efficiency of carbon capture technologies when processing flue gas. These factors include:
- Gas Composition: The presence of impurities and other gases in flue gas can interfere with capture efficiency. For example, high concentrations of nitrogen oxides or sulfur oxides can hinder the capture process.
- Temperature and Pressure: Optimal operating conditions are crucial for efficient capture. Each technology may perform better under specific temperature and pressure conditions. For instance, some absorption solvents may work more effectively at elevated temperatures, whereas others might require colder settings.
- Contact Time: The duration that the flue gas interacts with the capture medium significantly affects efficiency. Longer contact times can enhance the absorption of carbon dioxide but may also lead to diminishing returns in system throughput.
- Operational Parameters: Factors such as flow rates, humidity, and regeneration cycles for capture materials also play a vital role in determining how effectively COโ is captured from flue gas.
Understanding these factors is essential for optimizing carbon capture systems and improving their operational viability.
Performance Metrics for Technologies
To evaluate the performance of carbon capture technologies, several key metrics are commonly used:
- Capture Rate: This metric refers to the percentage of COโ effectively removed from flue gas. A high capture rate indicates a more efficient system.
- Cost per Ton of COโ Captured: This economic metric provides insight into the financial sustainability of the technology. It totals both capital and operating expenses, revealing the economic viability over time.
- Energy Consumption: The energy requirements for operating carbon capture systems are significant. Reducing energy consumption per ton of COโ captured is critical for enhancing overall efficiency and sustainability.
- Reusability of Capture Medium: The lifespan and regeneration potential of materials used for capturing COโ are crucial for long-term operational efficiency. Materials that can be regenerated multiple times without significant loss in performance contribute to reduced overall costs.
Performance metrics not only quantify the operational efficiency but also impact the economic feasibility of carbon capture technologies.
By examining these performance metrics and the factors affecting capture efficiency, stakeholders can better assess the readiness and role of carbon capture technologies in mitigating carbon emissions effectively.
Cost Analysis of Carbon Capture Technologies
The financial implications of carbon capture technologies are critical for their adoption and scalability. Understanding the costs involved in implementing such systems can significantly influence stakeholders' decisions, from governments to private industries. The costs are not just monetary; they encompass initial investments, operational expenses, and the overall economic feasibility of the technology. With growing urgency to address climate change issues, the cost analysis of carbon capture is paramount in propelling forward feasible solutions that contribute positively toward environmental sustainability.
Initial Investment and Operational Costs
The initial investment in carbon capture technology varies considerably depending on the specific method used and the scale of implementation. Factors that play a role in these costs include:
- Infrastructure Development: Creating the necessary facilities involves significant expenditure, from construction to integration with existing processes.
- Equipment and Technology: The procurement of capture systems, whether they involve chemical absorption or membrane technologies, can be costly. It is critical to choose efficient methods that align with the plant's operations.
- Land and Location: Geographical considerations can also affect the initial setup. Places with available infrastructure might have lower costs compared to more remote locations.
Operating costs follow the initial investment and include energy consumption, maintenance, labor, and consumables. Energy costs are especially significant since carbon capture systems require considerable energy to function efficiently. Reducing these operational expenses is essential for the sustainability of carbon capture.
"Cost-effective carbon capture is crucial for mitigating climate change."
Cost Reduction Strategies
To enhance the economic viability of carbon capture technologies, several strategies can be employed:
- Process Optimization: Improving the efficiency of capture processes helps in lowering operational costs. This includes utilizing advanced monitoring and control systems to manage energy consumption effectively.
- Economies of Scale: Large-scale implementations can reduce the per-unit cost of carbon capture. Encouraging collective projects where infrastructure is shared can lead to significant cost savings.
- Innovative Technologies: Investing in research and development for new materials and methods can decrease both initial and operational costs. For example, exploring more effective solvents or sorbents for capturing CO2 can yield better results at lower costs.
- Government Incentives: Policy support is crucial. Governments can provide tax credits, grants, or subsidies to companies that invest in carbon capture. These incentives can offset initial investments, encouraging greater adoption of these technologies.
By analyzing costs associated with carbon capture technologies, stakeholders can make informed decisions. The advancements in cost-reducing innovations and measures can promote the widespread implementation of these essential systems, ultimately supporting global efforts to mitigate climate change.
Policy and Regulatory Framework
The policy and regulatory framework surrounding carbon capture technology is critical. It creates the environment for investment, innovation, and wider adoption of these technologies. Policymakers must understand the significance of carbon capture from flue gas as part of a broader strategy to combat climate change. An effective and supportive regulatory framework can not only incentivize the development of carbon capture methods but also ensure that they are deployed effectively and ethically in various industries.
International Agreements and Treaties
International agreements play a crucial role in shaping carbon capture initiatives globally. Notable treaties, such as the Paris Agreement, emphasize the necessity to reduce greenhouse gas emissions. These agreements often include specific targets for emission reductions, pushing countries to explore advanced technologies like carbon capture. Compliance with these international obligations can lead nations to invest in carbon capture methods as a means to meet their commitments while also fostering sustainable economic growth.
"International cooperation is vital for reducing carbon emissions, and carbon capture technologies are critical components of that strategy."
Countries partaking in such agreements may offer funding or incentives for research and innovation in carbon capture technology. This international perspective promotes collaboration and sharing of knowledge across borders, advancing the progress and implementation of related technologies.
National Policies on Carbon Emissions Reduction
National policies significantly influence how carbon capture technologies are integrated into various sectors. Many countries have set specific goals or targets for reducing carbon emissions, often embedded in broader environmental strategies. These national frameworks can include regulations, subsidies, or tax incentives to encourage industries to adopt carbon capture solutions.
For instance, national policies may mandate emission reductions from fossil fuel industries while providing financial aids for implementing carbon capture infrastructure. This creates a favorable investment climate and encourages industries to prioritize the adoption of these technologies. Furthermore, transparency and accountability in reporting emissions can help track progress and promote responsible practices.
National policies are, therefore, integral to bridging the gap between carbon capture technologies and their practical implementation in various sectors including power generation, cement production, and steel manufacturing. In summary, a robust policy landscape not only drives technological advancement but is also instrumental in achieving climate goals.
Challenges in Implementation
The implementation of carbon capture technologies poses a significant challenge across several dimensions. This section highlights the core technical difficulties, economic barriers, and the influence of public perception that can impact the successful deployment of carbon capture from flue gas. Each challenge not only affects the effectiveness of carbon capture but also plays a crucial role in shaping policies and investments in this field.
Technical Challenges
Technical challenges encompass the complexities involved in capturing carbon dioxide effectively from flue gas. An issue that emerges frequently is the specific design of capture systems. Existing technologies often require substantial modification to accommodate varying flue gas compositions from different processes, such as power generation and industrial manufacturing. The variability in flow rates and concentrations of carbon dioxide can hinder the optimization of these systems, leading to inefficiencies.
Moreover, integrating these capture technologies into existing industrial infrastructures presents a formidable barrier. For instance, fitting large absorption columns or adsorption units into pre-existing facilities may require significant retrofitting, which can result in operational downtime and increase costs.
Another aspect is the energy requirement for the capture processes. Many established methods consume considerable amounts of energy, further complicating sustainability efforts. Finding solutions to enhance energy efficiency in these systems is crucial yet remains a technical hurdle. The development of innovative capture technologies that minimize these energy demands is essential for broader adoption.
Economic Barriers
Economic considerations represent a fundamental obstacle to the advancement of carbon capture technologies. Initial investment requirements are often substantial. Facilities seeking to implement these systems must allocate significant resources for technology purchase and installation, which can deter many companies. Economic feasibility often hinges on perceived return on investment, which can be uncertain in the early stages.
Operational costs further compound this issue. Maintaining and running carbon capture systems incurs ongoing expenses, such as electricity, maintenance, and personnel training. If operational costs remain high, companies may resist implementing these technologies, particularly in industries where profit margins are thin.
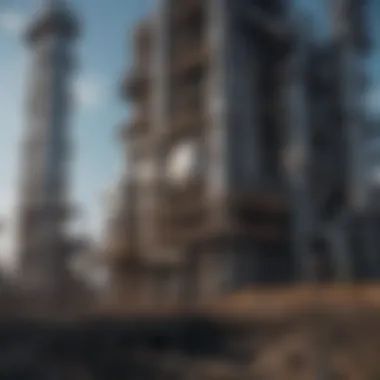
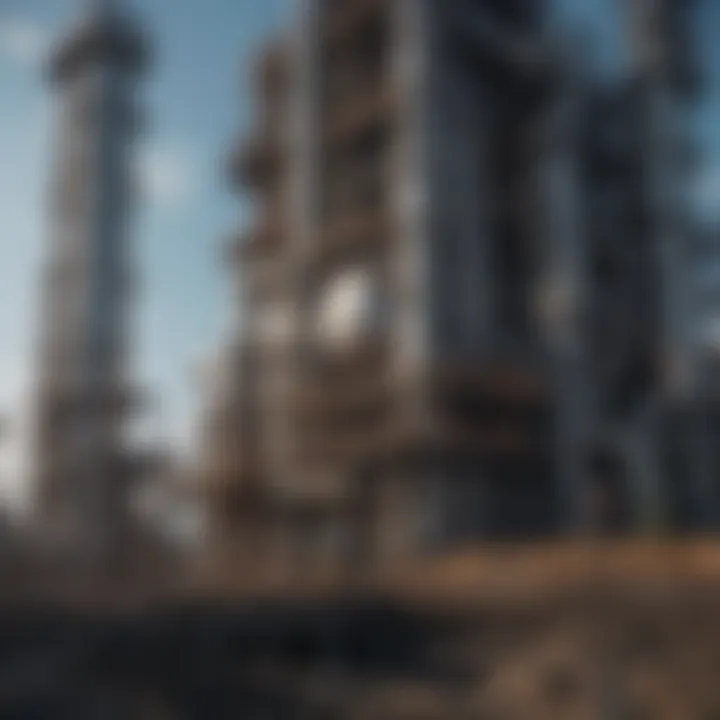
Additionally, fluctuating carbon pricing and varying regulations can induce uncertainties in financial planning. Without stable and predictable policy environments, industries might hesitate to invest in long-term solutions such as carbon capture.
Public Perception and Acceptance
Public perception plays a pivotal role in determining the future of carbon capture. Society's understanding of climate issues and the potential of carbon capture technologies can significantly influence political will and funding. If the public views these technologies positively, support for policies promoting carbon capture can increase.
However, skepticism remains a challenge. Concerns may arise about the safety and effectiveness of carbon capture systems. Misunderstanding of the technology and its implications may lead to resistance among local communities, especially if proximity to industrial sites is an issue. For instance, fears about potential leaks or health implications can hinder acceptance.
Engagement and educational initiatives are essential in addressing these perceptions. Successful communication around the benefits of carbon capture, coupled with transparency in operations, can dispel fears and build trust.
Implementing effective carbon capture requires overcoming a complex interplay of technical, economic, and public challenges. Understanding these barriers is crucial for stakeholders involved in climate policy formulations.
In summary, the challenges in implementing carbon capture from flue gas are multifaceted. Focusing on resolving technical difficulties, minimizing economic barriers, and addressing public perceptions is crucial for the successful adoption of these systems.
Future Prospects of Carbon Capture
The future of carbon capture technology is vital for meeting climate targets and reducing greenhouse gas emissions. As the world grapples with the threatening impacts of climate change, innovative strategies become necessary to address this issue. Carbon capture from flue gas is one such strategy that shows promise. The integration of emerging technologies, evolving research, and the synergy with renewable energy sources will shape the future landscape of this field.
Emerging Technologies
New advancements in carbon capture technologies continue to emerge. These innovations hold the potential to enhance efficiency and reduce costs significantly. For instance, developments in direct air capture and innovative materials for absorbents present exciting opportunities.
- Direct Air Capture: This method draws carbon dioxide directly from the atmosphere. Technologies in this area are beginning to refine their processes, aiming for lower capture costs and increased effectiveness.
- Advanced Sorbents and Membranes: New materials that can better selectivity capture CO2 while minimizing energy consumption are under development. These materials offer improved performance over traditional technologies.
- Integration of Artificial Intelligence: AI is being used to optimize processes in real-time. Machine learning algorithms can predict and enhance the performance of carbon capture systems based on varying operational conditions.
These emerging technologies are critical in progressive carbon capture, ensuring it is not just economically viable but also effective in reducing emissions.
Research and Development Trends
Continuous research and development are needed to advance the field of carbon capture from flue gas. Current trends show a strong focus on enhancing existing technologies while exploring novel concepts.
- Nanotechnology: The use of nanomaterials in capture processes is gaining attention. These materials can have enhanced surface areas and reactivity, which could lead to higher capture rates at a reduced cost.
- Hybrid Systems: Research is increasingly looking at combining multiple technologies, such as adsorption and absorption, to capitalize on their strengths and mitigate weaknesses.
- Life Cycle Assessment: Comprehensive evaluations of environmental impacts are becoming standard practice in R&D. Understanding the life-cycle impacts of these technologies ensures that efforts lead to genuine benefits for the environment.
Through dedicated research efforts, the promise of improved efficiency and performance in carbon capture technologies can be realized.
Integrating Carbon Capture with Renewable Energy
The integration of carbon capture technologies with renewable energy sources represents a substantial opportunity. By coupling these systems, many benefits can occur:
- Enhanced Efficiency: Renewable energy can power carbon capture systems. This reduces the carbon footprint of the capture process itself, creating a more sustainable overall operation.
- Storage of Renewable Energy: Carbon capture can serve as a method for storing excess renewable energy by using it to drive capture processes. This could address intermittency issues associated with solar and wind power.
- Support for Carbon Neutral Goals: By combining these technologies, industries can make significant strides towards carbon neutrality, addressing both emissions and the renewable energy supply chain.
The collaboration between carbon capture and renewable energy illustrates an essential pathway forward in the fight against climate change. It ensures that carbon capture is not an isolated solution but part of a broader, integrated approach to sustainability.
In summary, the future prospects of carbon capture are promising, driven by new technologies, research advancements, and integration with renewable energy. These factors collectively contribute to an evolving landscape that addresses pressing environmental challenges.
Implications of Carbon Capture
Carbon capture operations carry significant implications for both the environment and policy frameworks surrounding climate control. By understanding these implications, we gain a clearer insight into our path forward in addressing global warming. This section discusses the critical aspects of carbon capture's impact on the global carbon footprint and its influence on climate change policies.
Impact on Global Carbon Footprint
The global carbon footprint is primarily shaped by carbon dioxide emissions from energy production, industrial activities, and transportation. Carbon capture technology holds the potential to drastically reduce these emissions. By capturing emissions at their source, carbon capture not only minimizes the amount of CO2 released into the atmosphere but also allows industries to continue operating while adhering to increasingly stringent environmental standards.
- The technology can capture up to 90% of the CO2 produced by large point sources, such as coal-fired power plants and cement factories. This high capture efficiency directly translates into significant reductions in the total emissions contributed by these sectors.
- Beyond just mitigation, captured CO2 can be utilized in various processes, such as enhanced oil recovery or as a raw material for producing chemicals. This not only helps in diverting carbon from the atmosphere but also contributes to a circular economy by transforming waste into value-added products.
Furthermore, the introduction of carbon capture at scale can lead to a more sustainable industrial framework. This involves that companies may improve their public image by demonstrating a commitment to sustainability. This can also create new markets and job opportunities focused on low-carbon technologies.
"The implementation of carbon capture technologies stands as a beacon of hope in our urgent climate response efforts, reflecting both innovation and responsibility in tackling global warming."
Influence on Climate Change Policies
The implications of carbon capture extend into the realm of policy and regulation. As societies strive to meet international climate commitments, such as those outlined in the Paris Agreement, carbon capture gives countries an additional tool in their climate action arsenal. Its integration into national strategies can significantly alter the effectiveness of emissions reduction goals.
- Policymakers can promote carbon capture by establishing clear regulations and providing incentives, like tax credits, for companies investing in these technologies. This will accelerate the adoption of carbon capture solutions across various sectors.
- Additionally, carbon capture can aid in fulfilling Net Zero commitments. Countries aiming to balance their emissions through offsetting cannot realistically achieve this without capturing and storing a portion of emissions that cannot be eliminated.
- Integrating robust carbon capture technologies into climate policies encourages private sector investment and innovation. This creates an environment where technological advancements can flourish, leading to ongoing optimization of carbon capture processes.
While the potential benefits of carbon capture are notable, they also highlight the challenges of implementation and regulation. Policymakers must weigh the costs associated with developing these technologies against the environmental benefits and necessity for urgent action. The dynamic interplay between carbon capture and policy frameworks is crucial as we progress towards sustainable practices in addressing climate change.
Finale
The conclusion serves as a vital component in the discussion of carbon capture technology, specifically regarding its importance and implications for climate policy and future sustainability. The key elements discussed throughout the article help in understanding the multifaceted nature of carbon capture from flue gas. These elements illuminate not only the technological advancements and mechanisms involved but also the broader impact on climate change initiatives.
Summary of Key Points
- Carbon Capture Technologies: Various methods, such as absorption, adsorption, and membrane separation, demonstrate differing efficiencies in capturing carbon from flue gas.
- Effectiveness and Efficiency: The discussion highlights that the capture efficiency is influenced by multiple factors including technology choice and operational conditions.
- Challenges in Implementation: Despite the promising technologies, significant technical and economic hurdles still exist that need addressing for successful deployment.
- Future Prospects: Emerging technologies and integration with renewable energy sources present exciting opportunities for enhancing carbon capture efforts.
- Policy Implications: Understanding carbon capture's role informs better regulatory frameworks essential for climate change mitigation.
This summary emphasizes the complex interplay between technology, policy, and the urgency of climate change. It reinforces that a successful transition to efficient carbon capture processes is key to reducing the global carbon footprint.
The Path Forward for Carbon Capture Technology
The path forward for carbon capture technology is both challenging and promising. Progress depends on several critical factors:
- Research and Innovation: Continued investment in research can spur the development of more efficient and cost-effective carbon capture solutions.
- Collaboration: Engaging stakeholders from academia, industry, and government fosters innovation and knowledge sharing.
- Regulatory Support: Clear and supportive policies can help scale technologies and integrate them into existing industrial processes.
"The commitment towards future innovations in carbon capture can significantly alter our carbon emissions trajectory."
Moving forward, the focus should be on addressing existing challenges while capitalizing on emerging technologies. Enhanced collaboration, regulatory support, and continuous research will be essential for advancing carbon capture solutions.
Ultimately, the integration of these technologies into our industrial framework is critical for achieving climate objectives and promoting sustainability globally.