Exploring ATP Fluorescence Assay: Methodology and Uses
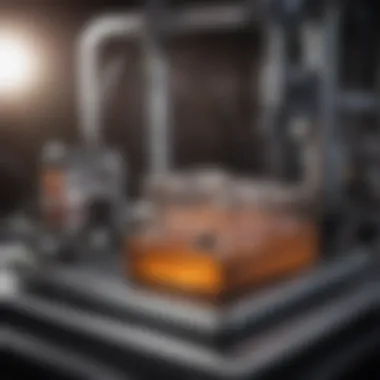
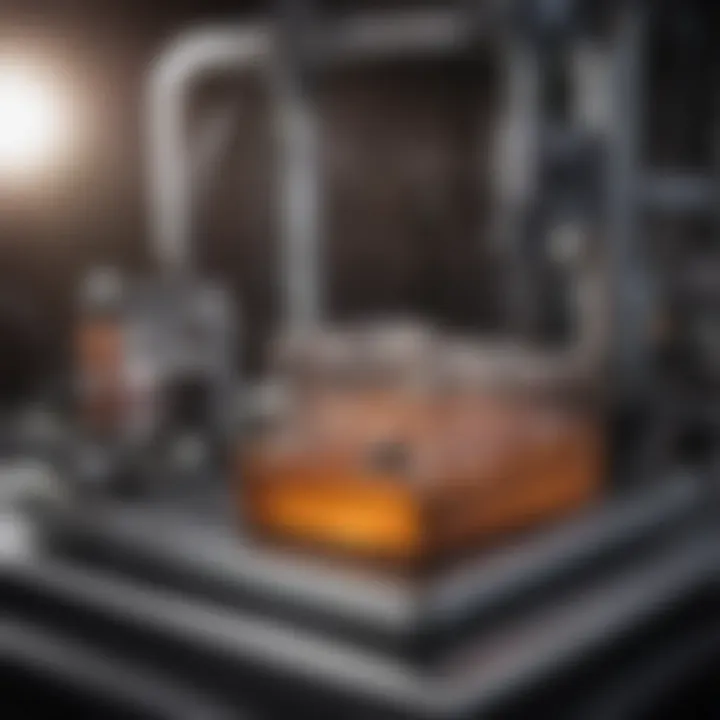
Intro
Adenosine triphosphate (ATP) is often referred to as the cellular energy currency. Understanding its concentration in various biological systems can provide essential insights into cellular health and metabolic activity. The ATP fluorescence assay stands out as an innovative method to quantify ATP levels through the measure of fluorescence intensity. While this technique has gained traction in biochemistry, it's important to unravel its intricacies and appreciate its diverse applications across different scientific disciplines.
The fluorescence assay utilizes specific binding molecules to attach to ATP, resulting in a measurable fluorescence signal. This not only quantifies ATP but also reflects the viability of cells. In the world of research, where every data point can lead to significant discoveries, mastering the ATP fluorescence assay becomes invaluable. The methodology, applications, and future directions of this assay will be explored in detail, paving the way for enhanced understanding and broader adoption among researchers, educators, and professionals in the field.
Methodology
Overview of Research Methods Used
The ATP fluorescence assay encompasses a variety of methodologies tailored to specific experimental needs. Researchers often start with sample preparation, where cells or biological fluids are treated to release ATP. Depending on the samples, this process can vary greatly. After this, specific luminogenic substrates are introduced, which interact with ATP, producing a detectable signal.
Different instruments may be utilized during the process. For instance, a microplate reader or a dedicated fluorescence spectrometer can be employed to assess the intensity of the emitted light. Typically, the assay's sensitivity is influenced by the choice of substrates and conditions tailored to the experimental design.
Data Collection Techniques
Data collection in ATP fluorescence assays requires precision. The fluorescence signal is recorded, often using high-throughput techniques to analyze multiple samples in one go. Various software tools assist in data analysis, transforming raw fluorescence values into ATP concentrations. Once the data is collected, comparison against a standard curve is essential for quantitative analysis. This curve, generated from known ATP concentrations, facilitates the transformation of fluorescence data into meaningful quantifications of ATP levels, illuminating cellular activity across numerous settings.
"Measuring ATP levels through fluorescence isn't just a technique; it’s a window into the biological processes at play within cells."
Future Directions
Upcoming Trends in Research
As technology marches forward, newer assays might be developed that increase the sensitivity and specificity of ATP detection. Advancements in microplate analysis and the introduction of nanoscale measurement techniques could enhance our ability to study ATP in smaller volumes and complex environments. In particular, the integration of artificial intelligence could allow for better data collection and interpretation, presenting a pathway for real-time monitoring of cellular activity. These ongoing developments might open avenues for exploring human health, environmental monitoring, and various types of cellular research.
Areas Requiring Further Investigation
Despite the current robust utility of ATP fluorescence assays, several areas beckon further investigation. The standardization of these assays represents an ongoing challenge. Variability in results across different laboratories underlines the necessity for established protocols.
Furthermore, a critical examination of the assay's application in complex biological matrices, like tumor environments or microbial ecosystems, remains largely underexplored. Addressing these challenges will undoubtedly elevate the reliability and applicability of the ATP fluorescence assay across various fields of research.
Understanding ATP and Its Role in Cellular Functions
Adenosine triphosphate, or ATP, stands as a cornerstone in the biochemistry of life. Its critical function as an energy carrier makes it an essential player in various cellular processes. In this section, we delve into the biochemical significance of ATP, examining its role in metabolic pathways and elucidating why researchers focused on ATP fluorescence assays must have a solid grasp of this molecule's importance.
Biochemical Significance of ATP
ATP is often dubbed the "molecular currency" of intracellular energy transfer. This title isn't merely poetic; it reflects the reality of ATP's function across countless biochemical reactions. ATP consists of adenosine—a nucleoside composed of adenine and ribose—attached to three phosphate groups. The bonds between these phosphates store significant energy, which cells harness for a host of tasks.
When ATP is converted to adenosine diphosphate (ADP) and inorganic phosphate, energy is unleashed. This energy fuels activities such as:
- Muscle contraction, where rapid ATP hydrolysis supports movement and force generation.
- Synthesis of macromolecules including proteins, nucleic acids, and lipids, allowing cellular growth and repair.
- Active transport processes that move substances across cell membranes against their concentration gradients, maintaining cellular homeostasis.
In essence, without ATP, cells would stagnate and organismal life would grind to a halt. Its centrality in biochemistry clearly outlines why methodologies that measure ATP levels offer significant insights into cellular viability and metabolism.
ATP in Metabolic Pathways
ATP’s role in metabolic pathways is akin to a maestro delicately conducting a symphony, directing various processes to ensure harmony within the cellular environment. Energy production begins primarily within the mitochondria, where ATP is generated through processes such as oxidative phosphorylation and substrate-level phosphorylation. Each cycle of these processes marks a pivotal moment in cellular respiration:
- Glycolysis converts glucose into pyruvate, yielding a small amount of ATP along with metabolic intermediates.
- The Krebs cycle continues the transformation, oxidizing acetyl-CoA and generating NADH and FAD, which eventually funnel energy into the electron transport chain.
- In oxidative phosphorylation, the energy stored in NADH and FAD is finally converted into ATP in a coordinated manner.
Moreover, ATP isn't merely a product of metabolic activity; it also serves as a signal. Its levels and consumption rates indicate cellular stress and metabolic health, making ATP measurements invaluable in research settings. Thus, understanding how ATP is generated and utilized in metabolic pathways lays the foundation for comprehending the broader implications of the ATP fluorescence assay in studying cellular functions.
Understanding the role of ATP in cellular metabolism is crucial as it opens up avenues for exploring the effects of various stressors on cellular health. By measuring ATP levels, researchers can decipher cellular responses to environmental changes, drugs, or diseases.
Principles of Fluorescence in Biochemical Assays
Fluorescence is a phenomenon rooted in the interaction of light with matter, specifically how certain compounds can absorb photons and then re-emit them at a different wavelength. This property becomes significantly relevant in biochemical assays, especially for ATP fluorescence assays, allowing for precise measurements in a range of applications, from cellular studies to clinical diagnostics.
The underlying principle is straightforward, yet its implications are profound: when a fluorophore absorbs energy, its electrons are excited to a higher energy state. As these electrons return to their ground state, energy is released in the form of light. The uniqueness of this phenomenon lies in its sensitivity. Even small amounts of a target molecule, like ATP, can be detected effectively when paired with an appropriate fluorophore.
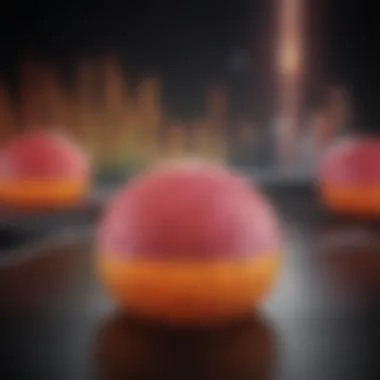
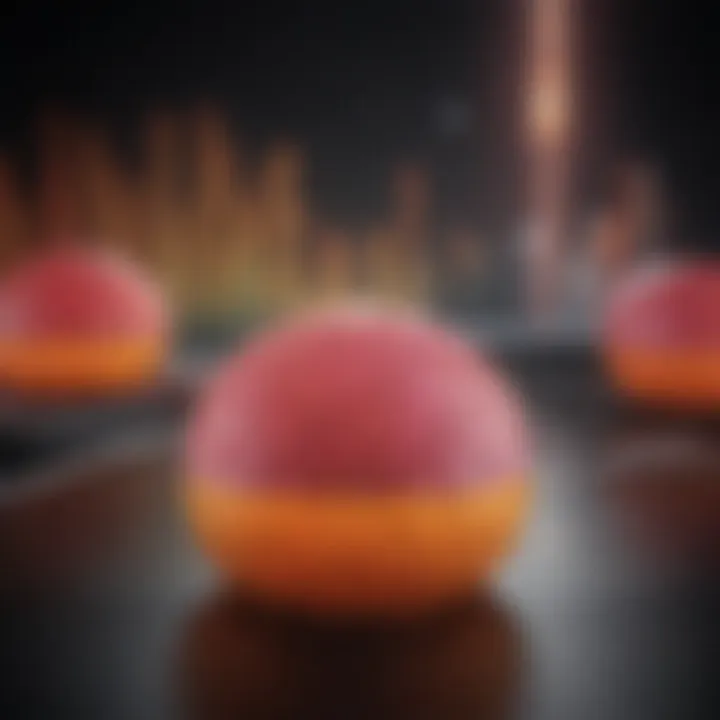
Fundamentals of Fluorescence
To grasp the fundamentals of fluorescence, one must understand the cycles of excitation and emission. When light is directed at a sample, fluorophores within the sample absorb this light, typically in the ultraviolet or visible spectrum. As they transition between energy states, they yield emitted light of a longer wavelength, usually visible to the naked eye.
The efficiency of this process can be influenced by various factors:
- Concentration of Fluorophore: Too much or too little can lead to quenching or insufficient signal.
- Environmental Conditions: pH, temperature, and solvent composition can all affect fluorescence intensity.
- Path Length: The distance light travels through a sample affects the amount of emitted light detected.
By maintaining optimal conditions, researchers can maximize the signal-to-noise ratio, ensuring clear and reliable results.
Fluorophores and Their Selection
Choosing the right fluorophore is akin to selecting the right tool for a job—critical for achieving accurate assay results. Several considerations come into play during this selection process:
- Emission Wavelength: The fluorescence must be distinct from background signals, allowing for clear detection amidst noise.
- Quantum Yield: A high quantum yield indicates a greater fraction of absorbed light is re-emitted, thus enhancing assay sensitivity.
- Stability: Fluorophores must withstand the conditions within which they are used, including potential degradation due to photobleaching or chemical interactions.
- Compatibility with Biological Systems: Some fluorophores may interfere with cellular processes, so it is crucial to use those that will provide accurate readings without affecting cell health.
Additionally, there are several categories of fluorophores tailored to specific applications. For instance, fluorescein is often used in microbiological assays due to its high quantum yield, whereas cyanine dyes offer flexibility in applications that require various emission spectra.
Understanding the robust principles of fluorescence and making informed choices about fluorophore selection amplify the utility and reliability of ATP fluorescence assays, paving the way for deep dives into cellular metabolism and viability.
The careful orchestration of fluorescence principles and fluorophore selection stands at the forefront of advancing biochemical assays, unlocking potential for groundbreaking research.
The ATP Fluorescence Assay Methodology
The ATP fluorescence assay represents a critical methodology in biochemical research, particularly in assessing cellular metabolism and viability. Understanding the methodology is essential as it combines intricate chemistry principles with practical applications that can enhance research quality. This section delves into the specific components of the assay, examining the reagents required, the step-by-step protocol, and how to optimize each experimental condition to obtain reliable results.
Reagents and Materials Required
In any successful assay, the selection of high-quality reagents cannot be overstated. For the ATP fluorescence assay, several key materials are indispensable:
- Adenosine Triphosphate (ATP): The primary analyte of interest. It is crucial to use pure ATP because impurities can significantly influence fluorescence readings.
- Fluorophores: These are chemical compounds that exhibit fluorescence. Selecting an appropriate fluorophore is vital for achieving optimal sensitivity. Common choices include firefly luciferase and commercial ATP assay kits that already incorporate these elements.
- Buffer Solutions: Phosphate-buffered saline (PBS) or Tris buffer is often used to maintain the pH environment conducive to ATP stability during the assay.
- Detection Plates: Choosing the right microplate is essential. Black opaque plates are often preferred to minimize background interference when measuring fluorescence.
Understanding the role of each reagent helps in grasping how variations can impact the results.
Assay Protocol Overview
Knowing the protocol is a stepping stone to executing the assay effectively. Here’s a simplified overview of the ATP fluorescence assay procedure:
- Sample Preparation: Cells or tissues must first be harvested, and lysis should be performed to release ATP into the solution. This could involve physical methods (like sonication) or chemical lysis using detergents.
- Addition of Reagents: Next, mix the prepared sample with the ATP-specific luminescent reagent. The quantity of reagents added should follow the manufacturer's recommendations to ensure consistent results.
- Incubation: Allow the mixture to incubate at room temperature for a specified time to ensure a complete reaction. This period can vary based on the kit used, typically ranging from 10 minutes to several hours.
- Measurement: Using a fluorescence reader, measure the intensity of fluorescence. The results are often expressed in relative light units (RLU), which correlate directly to ATP concentration within the samples.
This process underscores not just the careful coordination of each step, but also the precision necessary to extract meaningful data.
Optimization of Experimental Conditions
To refine the assay and yield the most reliable results, optimization of experimental conditions is non-negotiable. Considerations include:
- Temperature Control: ATP stability can vary with temperature, so maintain a consistent temperature throughout the assay. Typically, room temperature is sufficient, but keeping samples cold during preparation reduces degradation.
- Time Management: Allow adequate incubation time for the reactions to occur; however, be cautious not to overstretch the incubation duration, as this can lead to signal decay.
- Calibration: Regular calibration of the fluorescence reading equipment ensures accuracy. Using standard ATP concentrations can help in generating a reliable calibration curve, vital for interpreting results accurately.
- Sample Dilution: Overly concentrated samples may result in quenching of fluorescence. Diluting samples can help reach optimum detection ranges and reproducibility.
By honing in on these experimental parameters, researchers can significantly boost the assay’s sensitivity and reproducibility, which are crucial for drawing accurate conclusions.
It’s essential to document each alteration in conditions to create a reliable experimental record, which can guide future experiments.
Technical Considerations in ATP Assays
When delving into the world of ATP fluorescence assays, understanding the technical considerations is of utmost importance. These nuances can significantly affect the reliability and accuracy of the results obtained, and grasping them can be the difference between a straightforward analysis and one fraught with errors. In this section, we will explore the critical aspects that ensure effective utilization of these assays. Two primary components to address are sensitivity and specificity, alongside potential interferences and limitations, all of which are essential for researchers and scientists alike.
Sensitivity and Specificity
Sensitivity refers to the ability of the assay to detect even the slightest amount of ATP present in a sample. This becomes especially crucial for studies involving low cellular activity or during the early stages of particular biochemical interactions. A highly sensitive assay can unveil metabolic disturbances that would otherwise go unnoticed. It ensures that researchers can accurately assess the health of cells or the effectiveness of treatments across varied contexts.
Specificity, on the other hand, describes the capacity of the assay to specifically measure ATP without interference from other substances present in the sample. This quality is vital when working with complex biological samples where other nucleotides or cellular components may be present. High specificity means that the results reflect true ATP concentrations, reducing the chances of false positives or negatives in data interpretation.
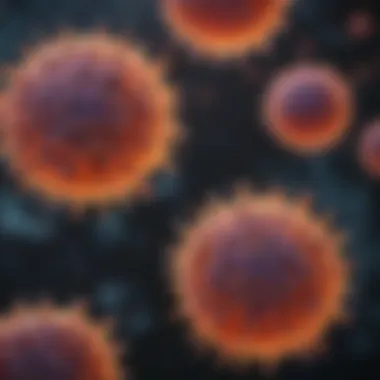
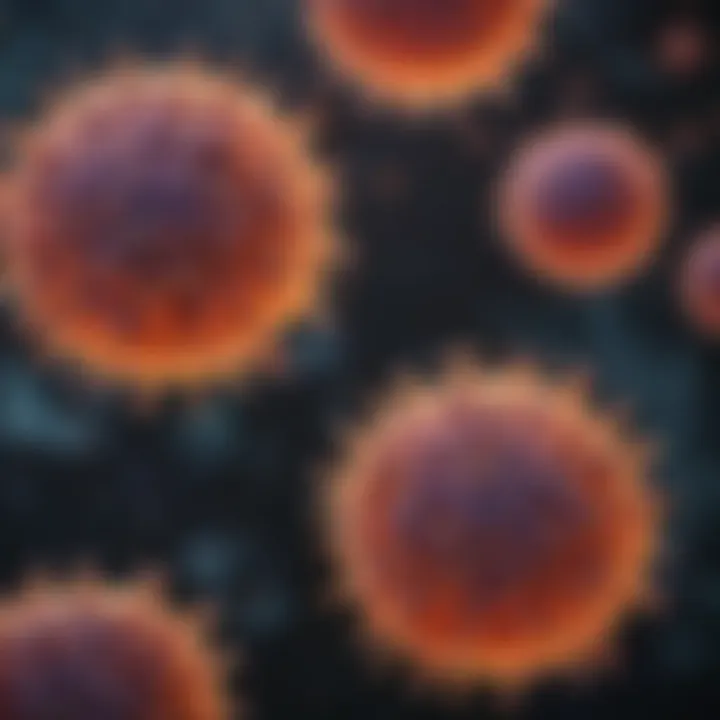
To achieve optimal sensitivity and specificity, careful selection of reagents and assay conditions is paramount. Factors such as the choice of fluorophore, incubation times, and environmental conditions (pH, temperature) all interplay to shape the outcome of the assay.
"In the realm of biochemical analysis, the subtle art of balancing sensitivity and specificity cannot be overstated; it's the foundation upon which trustworthy results are built."
Interferences and Limitations
While the ATP fluorescence assay is a powerful tool, it is not without its challenges. Understanding potential interferences is critical for ensuring experimental accuracy. Substances that can cause interference range from contaminants in samples to substances that can quench fluorescence signals. For example, certain metal ions or high concentrations of other nucleotides may disrupt fluorescence readouts, leading to erroneous conclusions.
Moreover, temperature fluctuations and pH variations can also affect the fluorescent response. For instance, if the environmental conditions deviate from the optimal range, it can lead to reduced fluorescence intensity, confounding results. Therefore, it is advisable to always include appropriate controls alongside experimental samples to identify such interferences.
Limitations must also be acknowledged. While fluorescence assays provide detailed information, they can sometimes lack quantitative precision when dealing with samples saturated with ATP or when cellular compartments are involved. In such cases, researchers may need to consider complementing the ATP fluorescence assay with other methods to fortify their findings.
Key Takeaways
- Sensitivity ensures detection of low ATP levels; specificity ensures true measurement of ATP without interference.
- The choice of compounds, along with environmental conditions, can substantially impact the accuracy of the assay results.
- Always consider potential interferences and limitations during experimental design to achieve more reliable outcomes.
In summary, the effectiveness of ATP fluorescence assays hinges on several technical considerations. Researchers must not only focus on assay design but also remain cognizant of the factors that can influence their results. By intimately understanding sensitivity, specificity, and potential pitfalls, the pathway to obtaining accurate and meaningful data becomes clearer.
Applications of the ATP Fluorescence Assay
The ATP fluorescence assay serves as a critical instrument in diverse research fields due to its effectiveness in providing quantitative insights into cellular and microbial life. This technique is not just another method tucked away in the laboratory toolbox; it is pivotal for understanding cell health, contamination levels, and drug interactions. As researchers strive to untangle the complexities of biological systems, the relevance of the ATP assay is becoming increasingly apparent. Below, we explore some key applications that demonstrate its utility.
Cell Viability Assessments
One of the primary applications of ATP fluorescence assays is in the realm of cell viability assessments. By measuring ATP concentrations, researchers can gauge the health and metabolic activity of cells in various conditions. This is particularly essential in studies where cell death or survival is under scrutiny, such as toxicological testing or cancer research.
- ATP as an indicator of vitality: High ATP levels often indicate active, living cells, whereas lower levels may signal cellular distress or death.
- Rapid assessments: The assay can be performed in a relatively short time, allowing for swift determination of cell status.
- Applicability across cell types: It can be applied to a wide range of cells, from mammalian cell lines to primary cultures, enhancing its versatility in research.
In practical terms, a researcher assessing the effect of a new pharmaceutical compound on cancer cells can utilize the ATP assay to provide valuable data on cell viability post-treatment. This data is crucial for evaluating the efficacy and safety of the treatment.
Microbial Contamination Detection
Beyond cell cultures, ATP assays are employed in the detection and quantification of microbial contamination. The presence of ATP is a reliable indicator of living organisms, thus allowing for rapid assessments of contamination in various environments, such as water treatment facilities, food processing industries, and clinical settings.
- Swift results: Traditional culture methods can be time-consuming, taking days to yield results, while ATP assays can deliver data within minutes.
- Non-selective detection: The assay measures all living organisms, allowing for a broad spectrum detection of contaminants.
- Cost-effective monitoring: This method proves to be efficient for routine monitoring of environments, ensuring safety and compliance with health regulations.
In essence, an ATP assay could determine the effectiveness of a sanitation procedure in a food production line by showing whether microbial ATP levels have been significantly reduced, thereby revealing the success of cleaning protocols.
Pharmacological Studies
Pharmacological research also benefits greatly from ATP fluorescence assays. As drugs target cellular mechanisms, understanding their effects on cellular ATP levels can elucidate their mode of action, efficacy, or potential toxicity.
- Mechanistic insights: By measuring ATP levels before and after drug administration, researchers can track how a compound influences cellular energy dynamics.
- Screening potential: In the development of new drugs, ATP assays can function as a high-throughput screening tool to identify promising candidates based on their effects on cell viability and metabolism.
- Toxicity evaluations: These assays can help in assessing the cytotoxicity of compounds, guiding safety assessments for new therapeutics.
For instance, a study could assess a new anti-cancer drug's impact by measuring ATP levels in tumor cells treated with different concentrations of the drug, providing immediate feedback on its efficacy and safety profile.
In summary, the ATP fluorescence assay stands as a multifaceted tool in scientific research, crucial for cell viability assessments, microbial contamination detection, and pharmacological studies. Its versatility makes it a go-to choice for researchers aiming for precision and insights in their biological investigations.
Comparative Analysis with Other Assays
In the realm of biochemical research, comparing the ATP fluorescence assay with other assay types is crucial for understanding its strengths and limitations. Knowledge of these comparisons allows researchers to select the most suitable method for their specific study goals, maximizing the relevance and accuracy of their results. The varying methodologies employed also highlight the wide-ranging applicability of the ATP assay in different contexts, demonstrating its versatility. By analyzing these other assay techniques, we can better appreciate the unique features of the ATP fluorescence assay and its role in cellular studies.
Luminescence-Based Assays
Luminescence-based assays are often paralleled with ATP fluorescence assays, as both methodologies leverage the bioluminescence phenomenon to measure chemical reactions. One of the key players in luminescence assays is luciferase enzyme, which catalyzes the conversion of luciferin into a product that produces light. In this context, the measurement of light emitted serves as an indicator of ATP concentration.
Key Considerations:
- Sensitivity: Luminescence assays are known for their high sensitivity, often detecting low levels of ATP with ease.
- Dynamic Range: They generally have a wide dynamic range, providing a capacity to quantify ATP over several orders of magnitude.
- Interference: One downside is the potential for interference from endogenous substances within the sample that can affect light yield.
The specificity of luminescence-based assays can vary, primarily due to the influence of other molecules that might alter the luminescent output. This may complicate the interpretation of results, particularly in biological samples where numerous compounds are present. The ATP fluorescence assay, on the other hand, celebrates a simpler analytical approach that can be meticulously validated for different experimental conditions.
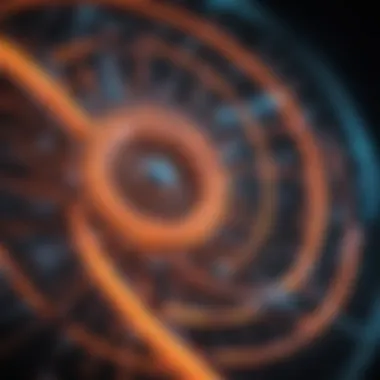
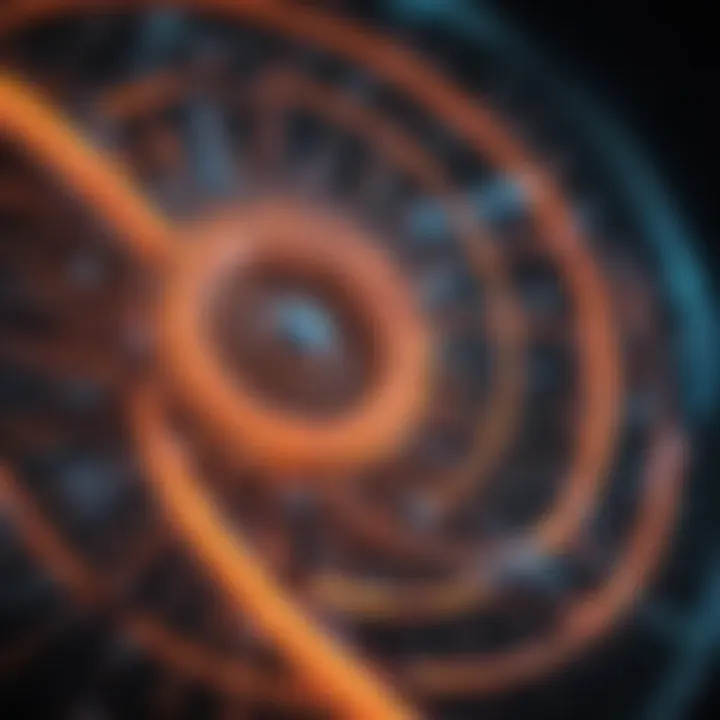
Colorimetric Assays
Colorimetric assays utilize a change in color intensity to infer the concentration of a given analyte, in this case, ATP. These assays typically employ reagents that react specifically with ATP to produce a colored compound. The intensity of the color change, measurable by spectrophotometry, gives a quantitative estimate of ATP levels in the sample.
Key Elements:
- Cost-Efficiency: Colorimetric assays are often less expensive to run than fluorescence or luminescence assays, making them attractive for lower-budget projects.
- Easy Visualization: The results can be quickly interpreted visually or through basic spectrophotometric techniques, useful in various laboratory settings.
- Limitations in Sensitivity: However, colorimetric assays may lack the sensitivity and specificity found in ATP fluorescence or luminescence options. Variations in sample matrix and potential color interference can skew results significantly.
In summary, while luminescence and colorimetric assays offer alternative approaches to ATP quantification, each method has specific strengths and weaknesses that necessitate careful consideration. The ATP fluorescence assay stands out as a robust option in many scenarios due to its reliability and ability to provide detailed insights into ATP levels without requiring extensive sample manipulation or costly reagents. Evaluating these alternatives is vital for researchers aiming to achieve precise data, ultimately enhancing the quality of scientific exploration.
Effective experiment design often revolves around the careful selection of the assay type. The right method can make all the difference in achieving accuracy in a researcher’s findings.
Future Perspectives in ATP Assay Development
The exploration of ATP fluorescence assays has opened a treasure trove of possibilities for advancements in biochemical research and application. The importance of focusing on the future perspectives of ATP assay development cannot be overstated, as it promises a shift in how researchers assess cellular activity and viability. Discussing advancements in technology and how they can be harnessed for better assay designs will provide more accurate, efficient, and versatile applications in various scientific fields.
A primary element that ties future developments to the current state of ATP assays is the ongoing need for improved sensitivity and reaction speed. Emerging technologies can offer benefits, such as real-time monitoring of ATP levels, which presents a chance for researchers to gather more dynamic data, enriching the insights into cellular states and functional conditions. Moreover, this future trajectory includes considerations of expanding the assay's versatility, allowing researchers to adapt it to different contexts, such as studying complex biological systems or high-throughput screenings.
As we look forward, understanding the implications of these innovations and their integration into research practices holds significant merit for scientists and professionals alike.
Technological Innovations
As we step into a new era of research, technological innovations play a crucial role in revolutionizing ATP assays. Instruments equipped with advanced fluorescent detection systems, such as multi-wavelength detection, heighten our capability to analyze ATP levels with unprecedented precision.
Given the intricacies of cellular processes, the introduction of microfluidic devices paves the way to miniaturized experiments that reduce reagent consumption and produce quicker results. This reflects a broader trend in biochemistry towards greener methodologies and resource efficiency.
Below are some notable technological advancements that may shape the future of ATP assays:
- Advanced Fluorometric Readers: These instruments can distinguish between closely related fluorophores, enhancing specificity.
- Integration with Artificial Intelligence: AI could optimize assay protocols and processing times through predictive modeling and data analysis.
- Wearable Biosensors: While still in developmental stages, these can offer real-time ATP readings in living organisms, providing valuable in-vivo insights.
Such innovations indicate a trend toward automation and enhanced capabilities in ATP assays, which could significantly transform research landscapes if fully embraced.
Potential for High-Throughput Screening
The potential for high-throughput screening (HTS) in ATP assays resonates strongly within research circles. HTS is a game-changer, allowing scientists to conduct thousands of tests simultaneously, resulting in expedited breakthroughs rather than nights in the lab counting cells.
The benefits of implementing ATP fluorescence assays in high-throughput environments are manifold:
- Speed: Responses can be evaluated much faster than traditional methods.
- Scalability: A single assay can be adapted for large sample sizes, essential for drug discovery and toxicology assessments.
- Increased Throughput: The ability to run numerous assays in parallel can lead to discovering new compounds or biological insights at a pace previously thought impossible.
Furthermore, the combination of ATP assays with robotics means routine tasks can be automated, permitting researchers to shed the manual labor typically associated with assays. This alignment not only boosts productivity but allows for a more strategic focus on experiment design and result interpretation.
Epilogue and Implications for Research
The ATP fluorescence assay stands as a significant innovation in the toolset available to researchers delving into cellular functions. This assay not only serves as a measurable indicator of cellular metabolism but also provides insights into cellular health and viability. The methodology's ability to reveal quantitative data about ATP concentrations opens up various avenues for research, particularly in fields like microbiology and pharmacology.
As discussed earlier, the robustness and sensitivity of ATP fluorescent measurement can uncover vital information regarding cell health, even in environments previously deemed too challenging for standard assays. Investigators can assess therapeutic efficacy during drug development or explore fundamental biological processes. As a result, the ATP fluorescence assay is not just a methodological choice; it forms the backbone of many experimental designs across disciplines.
"Understanding ATP levels is crucial in accurately interpreting cellular responses to various stimuli, whether they are environmental factors or pharmacological agents."
Considerations for future research highlight the potential enhancements in technology that can lead to even finer sensitivity and specificity in ATP measurements. As assays evolve, the implications for research grow. New techniques may emerge that allow simultaneous measurements of ATP alongside other biomarkers, offering a more comprehensive view of cellular dynamics. Moreover, the scalability for high-throughput environments makes this assay particularly ripe for advancements in drug discovery and environmental monitoring.
Overall, the ATP fluorescence assay is pivotal for shaping our understanding of cellular processes. Its implications stretch beyond simple measurement to inspire new experimental paradigms that can lead to groundbreaking discoveries in life sciences.
Summary of Key Insights
The key insights drawn from the ATP fluorescence assay encompass the following:
- Quantitative Measurement: Provides a direct correlation between cellular metabolic activity and ATP levels.
- Versatility: Applicable across various fields such as microbiology, pharmacology, and environmental science.
- Sensitivity: Capable of detecting hemodynamic changes in cells even at low ATP concentrations.
- Dynamic Range: Its adaptability to different experimental conditions allows for a broad range of applications.
In summary, the ATP fluorescence assay superbly integrates molecular biology with practical applications, making it indispensable for contemporary research.
Broader Impact on Scientific Research
The ATP fluorescence assay's influence extends well beyond individual studies, catalyzing broader shifts in how research is conducted across biological fields. By providing a reliable method to assess cell viability and metabolic function, it has become indispensable for various research domains.
- Drug Development: Researchers can utilize ATP measurements to evaluate drug effects and toxicity, speeding up the developmental process of new therapeutics.
- Pathogen Detection: The assay is instrumental in detecting microbial contamination in clinical and environmental samples, contributing to public health efforts.
- Biotechnology: The ability to monitor cellular responses in real-time supports innovations in bioprocessing and synthetic biology.
- Education and Training: As more institutions incorporate this assay into curriculum, it enhances practical knowledge for future scientists.
The collective impact emphasizes the ATP fluorescence assay's role not just as a lab technique but as a cornerstone for emerging research paradigms. It pushes the boundaries of scientific inquiry by enabling refined studies that tackle complex biological questions, ultimately influencing how we understand life at a cellular level.