Comprehensive Overview of Anatomical Tissues
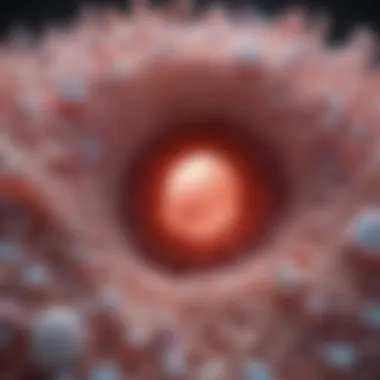
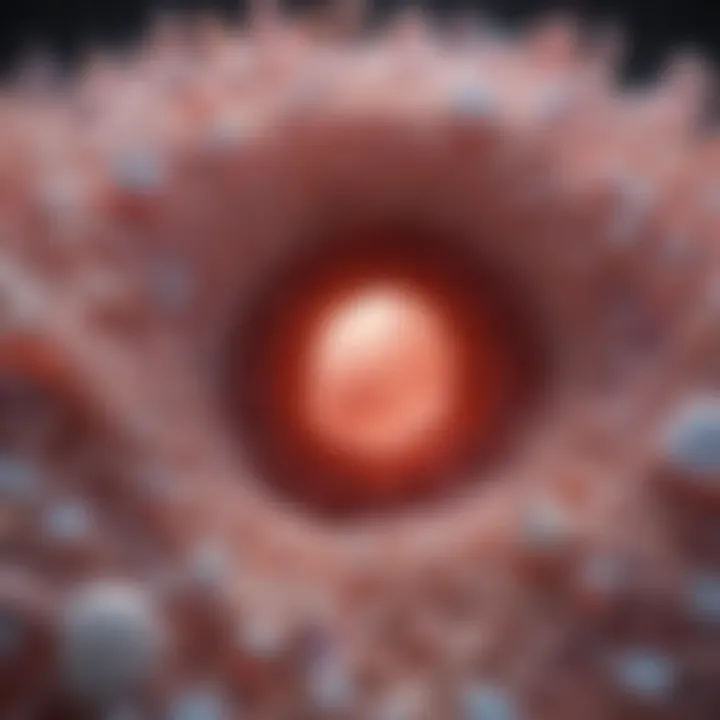
Intro
Understanding anatomical tissues is crucial to grasp the complexities of biological systems. Tissues are groups of cells that work together to perform specific functions. Their classifications into four primary types—epithelial, connective, muscle, and nervous—define their structure and roles. Each type plays a unique part in maintaining the body’s overall health and functionality.
This article will undertake a comprehensive overview of these tissue types, exploring their histological structures, functions, and significance in health and disease. The intricate relationships between tissues will be highlighted, elucidating how they interact within various biological systems.
Methodology
Overview of research methods used
The exploration of anatomical tissues utilizes various methodologies. Both qualitative and quantitative approaches are essential. Microscopy, particularly light and electron microscopy, is fundamental in studying tissue architecture at a cellular level. Additionally, immunohistochemistry allows for the visualization of specific proteins, revealing insights into tissue functionality.
Data collection techniques
Data is primarily collected through laboratory experiments and clinical studies. Histological samples are prepared using appropriate techniques to preserve tissue structure. Data on cellular composition and function is gathered through techniques like flow cytometry and gene expression analysis, providing a comprehensive view of tissue dynamics.
Discussion
Anatomical tissues are integral to the body's structure and function.
Epithelial Tissues
Epithelial tissues line surfaces and cavities within the body. They serve protective roles, as well as roles in secretion and absorption. These tissues are classified into simple and stratified types based on their layers. They are identified further as squamous, cuboidal, or columnar based on cell shape.
Connective Tissues
Connective tissues support, bind, and protect other tissues. They have diverse types, including loose connective tissue, dense connective tissue, cartilage, and bone. Each type possesses unique properties and functions essential for maintaining structural integrity.
Muscle Tissues
Muscle tissues are responsible for movement. There are three distinct types: skeletal, cardiac, and smooth. Skeletal muscles facilitate voluntary movements, while cardiac and smooth muscles control involuntary functions.
Nervous Tissues
Nervous tissues form the brain, spinal cord, and nerves. They are essential for signal transmission in the body. Neurons and glial cells work together to process information and coordinate responses.
"Tissues are not merely groups of cells; they form the very fabric of organismal architecture, determining how physiological processes unfold."
Future Directions
Upcoming trends in research
Future research in anatomical tissues is likely to focus on regenerative medicine and tissue engineering. Advances in biotechnology may enable the creation of synthetic tissues, addressing issues such as organ shortages for transplants.
Areas requiring further investigation
Further investigation is necessary into the functional implications of various extracellular matrices, particularly in the context of disease progression. Understanding the cellular microenvironment is critical for developing therapies against diseases such as cancer and fibrosis.
Understanding Tissues
The study of tissues represents a cornerstone of anatomy. Understanding tissues involves exploring the intricate structures that form the basis of all organs and systems in living organisms. This section establishes the foundation for the analysis of different types of tissues. It emphasizes both the complexity of tissue architecture and their fundamental roles in maintaining organismal function. By analyzing tissues, one can gain insights into both normal physiological processes and pathological states.
Definition of Tissues
Tissues are groups of cells that share a similar structure and function. They come together to perform specific tasks that are vital for the body's operation. In the human body, there are four primary types of tissues: epithelial, connective, muscle, and nervous tissues. Each of these types has distinct cellular compositions and functions that contribute to the overall health and performance of organ systems.
For example, epithelial tissues cover body surfaces and line cavities and organs. Connective tissues provide structural support and transport nutrients. Muscle tissues are responsible for movement, while nervous tissues facilitate communication within the body.
Historical Perspective
The history of tissue study dates back to early anatomical investigations. Ancient civilizations attempted to understand the body, but it was not until the advent of microscopy in the 17th century that the study of tissues evolved into a science. Pioneers like Marcello Malpighi and Giovanni Battista Morgagni contributed significantly to histology, the study of tissues.
Through the years, advances in staining techniques and electron microscopy have allowed for more detailed observations of tissue structures. This evolution has propelled our understanding of anatomy, leading to the classification and identification of various tissue types. The timeline culminates in present-day research, which continually sheds light on the complexities of tissues.
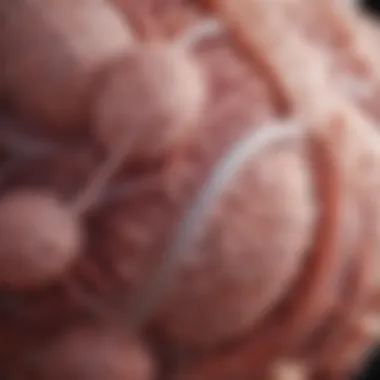
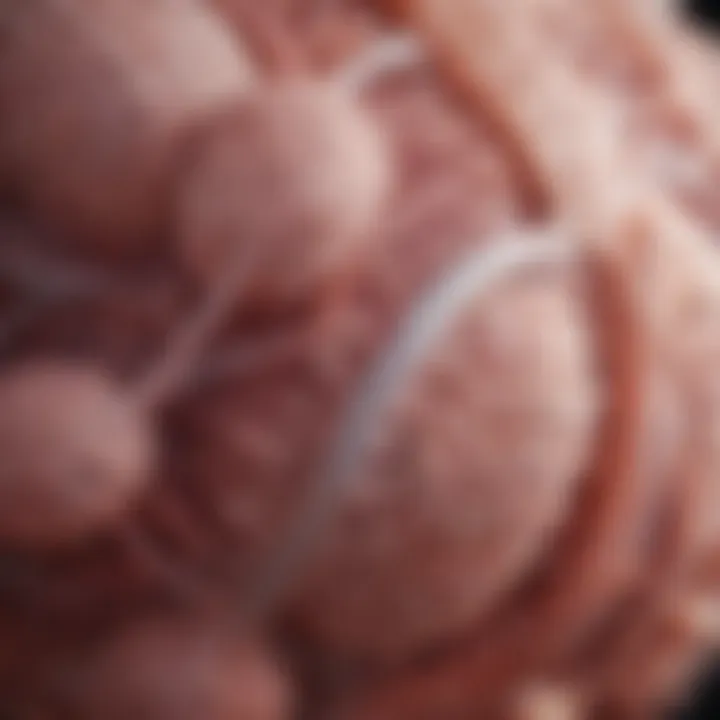
Importance of Tissues in Anatomy
Understanding tissues is crucial for multiple reasons. Firstly, tissues are the building blocks of organs. Their type and organization determine the function of each organ system. For instance, the arrangement of muscle fibers in cardiac muscle is essential for effective heart contractions.
Moreover, studying tissues aids in diagnosing various diseases. Changes in tissue structure can indicate pathological conditions. For example, cancer often involves alterations in epithelial tissues, leading to abnormal growths. Furthermore, knowledge of tissue repair mechanisms can inform treatments for injuries.
"The study of tissues not only broadens our comprehension of biology but also enhances our ability to address health challenges."
In addition, understanding how tissues interact within organ systems is vital for grasping homeostasis. This knowledge helps students and professionals alike develop a holistic view of bodily functions, thereby enriching the field of medical and biological sciences.
Classification of Tissues
Understanding the classification of tissues is fundamental to anatomy and biology. It allows us to categorize various cells that perform distinct but interrelated functions in the body. The four primary tissue types—epithelial, connective, muscle, and nervous—each play critical roles in the biological framework. Grasping these classifications leads to better insights into tissue functionality, connectivity, and overall anatomical networks.
The Four Main Tissue Types
The four main tissue types—epithelial, connective, muscle, and nervous tissues—form the building blocks for all organs. Each type has unique structures and functions:
- Epithelial Tissue: Acts as a protective barrier, covering body surfaces and lining cavities. It is crucial for absorption, secretion, and sensation.
- Connective Tissue: Provides support, binds other tissues, and stores energy. Varieties include adipose, bone, and blood, each serving specialized functions.
- Muscle Tissue: Facilitates movement through contractions. Types include smooth, cardiac, and skeletal muscle, each under control of different regulatory mechanisms.
- Nervous Tissue: Enables communication within the body. Comprising neurons and glial cells, it processes information and coordinates bodily functions.
Subtypes of Epithelial Tissue
Simple Epithelium
Simple epithelium consists of a single cell layer, making it capable of efficient absorption, secretion, and filtration. Its thin nature facilitates swift transport of materials. Key characteristics include the presence of junctions between cells and a basal membrane that supports the structure. As a common epithelial type, it is frequently found in areas requiring rapid exchange, such as alveoli in lungs and intestines. However, the simplicity may limit protection against mechanical and chemical damage.
Stratified Epithelium
Stratified epithelium contains multiple cell layers, enhancing its protective function against physical stress, abrasion, and infection. This type is prevalent in locations like the skin and esophagus. The standout feature of stratified epithelium is its ability to regenerate rapidly. While advantageous for protection, it is less efficient in absorption compared to simple epithelium due to its thicker structure.
Transitional Epithelium
Transitional epithelium is unique for its ability to stretch and change shape, thus accommodating various levels of distension. Typically found in the urinary bladder, its key benefit lies in its functionality during urine storage and passage. However, the complexity of its cellular arrangement might pose challenges for regeneration when damaged.
Subtypes of Connective Tissue
Loose Connective Tissue
Loose connective tissue has a sparse arrangement of fibers, allowing for flexibility and cushioning of organs. It holds structures together while providing a supportive framework. This type is essential for connecting epithelial tissues to underlying structures. Its flexible nature allows protection against mechanical stress, but it may be less effective in providing rigid support compared to dense connective tissue.
Dense Connective Tissue
Dense connective tissue is characterized by tightly packed collagen fibers, providing significant strength and elasticity. It forms tendons and ligaments, crucial for connecting muscles to bones and bones to each other. This density grants resistance to tensile forces, which is vital for structural integrity. However, it has limited blood supply, potentially leading to slower healing processes.
Specialized Connective Tissues
Specialized connective tissues include blood, bone, and cartilage, each serving specific and vital functions. Blood transports nutrients and waste, while bone provides structure and support to the body. Cartilage offers flexibility and cushioning at joints. Each specialized type possesses unique attributes essential for their respective roles, but they may face challenges like immobility or limited nutrient supply compared to more vascularized tissues.
Types of Muscle Tissue
Smooth Muscle
Smooth muscle is involuntary and non-striated, found in walls of hollow organs such as intestines and blood vessels. Its key characteristic is the ability to contract and relax unconsciously, managing essential bodily functions like digestion and blood flow. Smooth muscle's adaptability is a significant advantage, but its slow contraction pace might limit rapid reaction times.
Cardiac Muscle
Cardiac muscle is striated and involuntary, found exclusively in the heart. Its unique feature is the presence of intercalated disks, providing strong connections between cells for coordinated contractions. This syncytial characteristic ensures effective blood pumping throughout the body. While highly efficient, the loss of cardiac muscle cells can severely impact heart function.
Skeletal Muscle
Skeletal muscle is striated and voluntary, responsible for movements of the skeleton. Its fibers connect to bones allowing for conscious control of movement. The distinctive advantage of skeletal muscle lies in the rapid, powerful contractions it can generate. However, it requires extensive energy and is susceptible to fatigue after prolonged use.
Types of Nervous Tissue
Neurons
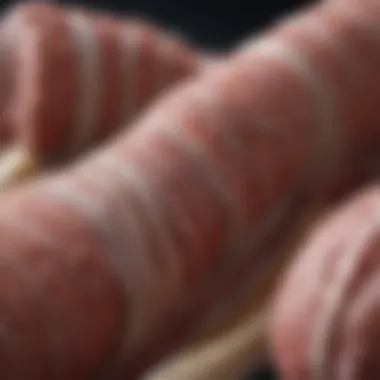
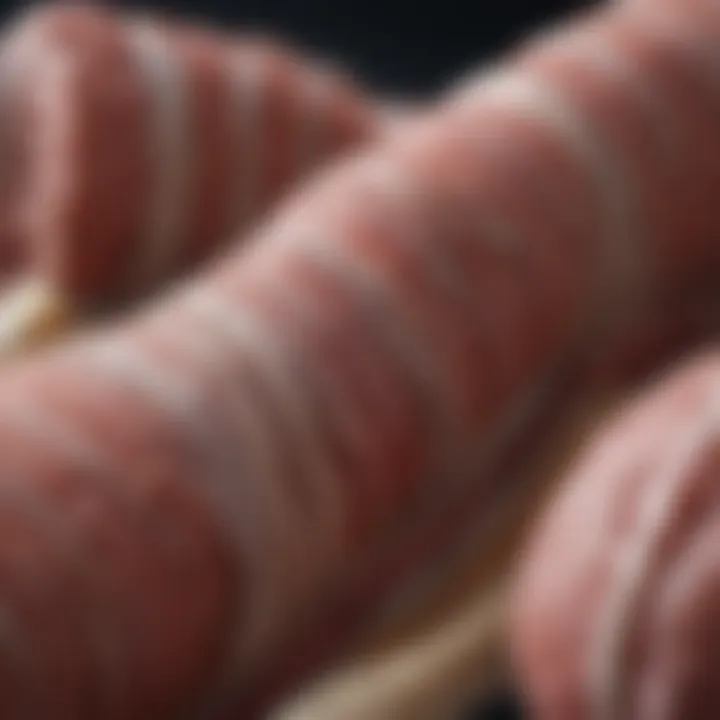
Neurons are the fundamental units of the nervous system, capable of transmitting electrical signals. Their unique structure, with dendrites and axons, allows for efficient communication throughout the body. Neurons play a crucial role in sensory perception, motor control, and cognitive functions. However, their ability to regenerate is limited, posing a challenge in recovery from injury.
Glial Cells
Glial cells support and protect neurons. They are abundant in the nervous system and perform various functions such as supplying nutrients, insulating axons, and clearing debris. While not involved in signal transmission, glial cells are critical for maintaining the homeostasis of the nervous system. Their adaptability and regenerative capacity make them vital for overall neural health.
Histological Examination of Tissues
Histological examination of tissues is a fundamental aspect of anatomy and biology. It allows us to delve deeper into the structural complexities and functions of tissues. This examination involves various techniques that help researchers and medical professionals visualize and analyze tissue samples. Thus, an in-depth understanding of histological methods is essential for anyone engaging with biological sciences.
Techniques of Tissue Staining
Tissue staining plays a crucial role in histological examination. Staining enhances the visibility of different components within a tissue sample. There are several staining techniques available, each serving a specific purpose. Common examples include hematoxylin-eosin staining and immunohistochemistry. Hematoxylin and eosin staining is widely used for routine examinations, as it effectively highlights nuclei and cytoplasmic features. Immunohistochemistry, on the other hand, allows for the targeted detection of specific proteins within tissues, providing insights into cellular functions and pathology.
Tissue Preparation Methods
Before histological examination, appropriate tissue preparation is vital. The process usually involves fixation, embedding, and sectioning. Fixation preserves tissue architecture by stabilizing proteins. Common fixatives include formaldehyde and glutaraldehyde. After fixation, the tissue is embedded in paraffin or resin to create a block for sectioning. The sections are thinly sliced to facilitate better observation under the microscope. These preparation methods are crucial for obtaining high-quality samples that yield reliable results during examination.
Microscopic Analysis of Tissues
Microscopic analysis is the cornerstone of histology. It involves observing tissue samples under a microscope to identify structural characteristics.
Light Microscopy
Light microscopy is a predominant method in tissue analysis. It provides a clear view of tissue architecture at a cellular level. The key characteristic of light microscopy is its use of visible light to illuminate samples. This method is popular due to its accessibility and ease of use in laboratories. One unique feature of light microscopy is its ability to produce color images of sections, making it easier to differentiate between various types of cells. However, light microscopy may not reveal the finer details of ultra-structure found in some biological specimens.
Electron Microscopy
Electron microscopy is another powerful tool for histological examination. It utilizes beams of electrons instead of light waves, allowing for much higher resolution and detailed visualization of tissue. The key characteristic of electron microscopy is its ability to magnify samples thousands of times greater than light microscopy. This makes it a beneficial choice for studying cellular organelles and detailed structures. However, it requires complex sample preparation and specialized equipment, making it less accessible than light microscopy.
Understanding the various histological examination methods enriches our comprehension of anatomical tissues. The ability to visualize tissues through different techniques provides considerable insights into biological functions, making it an essential part of scientific study.
Functional Implications of Tissues
Understanding the functional implications of tissues is crucial for comprehending how these biological structures collaborate to maintain life. The complexity of bodily functions hinges significantly on the attributes and roles of various tissue types. Each type of tissue contributes to specific physiological processes, forming the foundation upon which organ systems operate effectively. Through examining these implications, we gain insights into both normal physiological processes and the potential deviations observed in disease states.
Mechanical Properties of Tissues
Tissues possess unique mechanical properties that dictate their function and resilience. Elasticity, tensile strength, and compressibility are key mechanical aspects governing how tissues respond to external forces. For example:
- Connective tissue provides support and strength, facilitating load-bearing functions in structures such as cartilage and bone.
- Muscle tissue is designed to contract and generate force, allowing movement and stability.
- Epithelial tissue serves as protective barriers and is adaptable to mechanical stress, ensuring integrity in organs like the skin and lungs.
These properties are vital for maintaining structural integrity and function. If the mechanical characteristics of tissues change, it may lead to conditions such as fibrosis or atrophy, impacting overall health.
The Role of Tissues in Organ Systems
The collaboration of various tissues results in the formation of organ systems, each with distinct functions. For instance, the heart consists primarily of cardiac muscle tissue, supported by connective tissues that provide structure and flexibility. This organization enables the heart to efficiently pump blood throughout the body. Other notable examples include:
- The digestive system, where epithelial tissues facilitate nutrient absorption while connective tissues provide structural support.
- The nervous system, relying on the specialized roles of nervous tissue for signaling and communication.
These interactions exemplify how tissues contribute not only to the function of individual organs but also to the integration of systems vital for homeostasis and bodily function.
Tissue Interactions and Homeostasis
Tissues do not act independently; they interact in complex ways to maintain homeostasis. This balance is crucial for the body's overall stability and health. For example:
- Epithelial and connective tissues work together in organs like skin. The epithelial layer protects against environmental factors, while underlying connective tissue supports and nourishes it.
- Nervous tissue facilitates communication between tissue types, allowing for coordinated responses to internal and external stimuli.
A disruption in these interactions can lead to pathological conditions. For instance, chronic inflammation may alter tissue function and contribute to diseases such as arthritis. The intricate balance between different tissues highlights the significance of their functional implications in sustaining health.
"Tissues interact dynamically to facilitate homeostasis, underscoring their interdependence and complexity."
In summary, the functional implications of tissues are foundational for the understanding of anatomy and health. Each tissue type contributes uniquely to the integratedness of organ systems, emphasizing the importance of evaluating tissues not merely in isolation but as interconnected components of a larger biological framework.
Health and Disease Related to Tissues
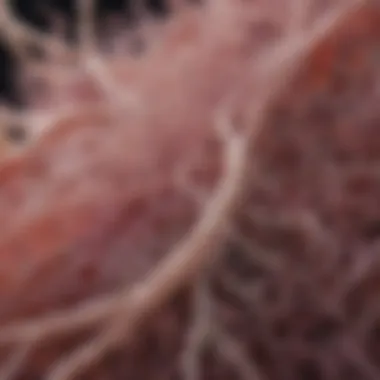
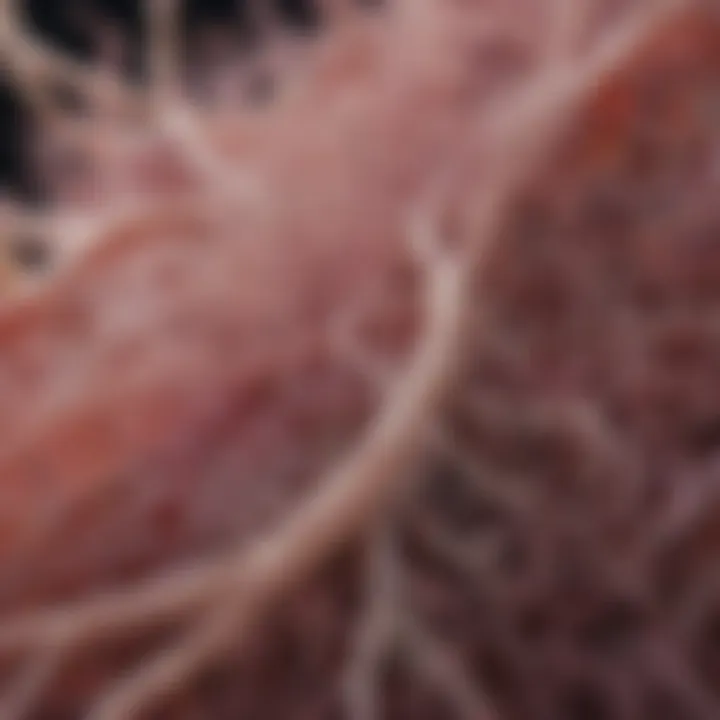
Understanding the relationship between health, disease, and anatomical tissues is crucial in the biological sciences. Tissues serve as the foundational blocks of all organs and systems in the body. Their proper structure and function are vital for maintaining overall health. Any alteration in a tissue's composition can lead to significant disease processes. This section outlines the importance of tissue health, mechanisms of tissue regeneration, the impact of diseases on tissue structure, and how cancer influences tissue differentiation.
Tissue Regeneration and Repair
Tissue regeneration and repair are natural processes that allow the body to heal after injury. This capacity varies significantly among different tissue types. For instance, epithelial tissues possess a high regenerative affinity, which enables quick recovery from minor injuries. Conversely, cardiac muscle tissues have limited regenerative abilities, often leading to scar formation after damage.
The regeneration process typically involves several stages: inflammation, tissue formation, and remodeling. Inflammation, the first response, helps to clear away damaged cells and pathogens. Following this, proliferating cells work to form new tissue. Finally, the remodeling phase restores the tissue's original structure and function. Advances in medical science, such as tissue engineering, are focused on enhancing the natural repair processes, using techniques like stem cell therapy to promote better healing outcomes.
Impact of Disease on Tissue Structure
Diseases can profoundly affect the structural integrity of tissues. Conditions such as diabetes can lead to damage in connective tissues, affecting their strength and elasticity. Additionally, autoimmune diseases may result in the destruction of specific tissue types, leading to systemic complications. For example, rheumatoid arthritis leads to the breakdown of joint tissues, causing pain and dysfunction.
Moreover, chronic inflammation can permanently alter tissue architecture, contributing to fibrosis or scarring. Fibrotic tissues lose their regular structure and function, impairing the overall health of organs affected, such as the liver in cirrhosis.
When evaluating the effects of diseases on tissue structure, a critical focus is on cellular changes observed in histological examinations. Healthy tissues exhibit well-organized structures, while diseased tissues show disorganization that correlates with functional impairments.
Cancer and Tissue Differentiation
Cancer represents a profound challenge in understanding tissue health. It arises from abnormal cell growth and differentiation, which disrupts the normal biological processes within tissues. Tumor cells often demonstrate uncontrolled proliferation, leading to the formation of masses that compromise the structural integrity of normal tissues.
Importantly, cancer can alter the characteristics of various tissues. Tumors can induce changes in the extracellular matrix, which affects not only the tumor itself but the surrounding tissue as well. This phenomenon contributes to metastasis, where cancer cells migrate to distant sites and establish secondary tumors.
"Understanding how cancer interacts with tissue differentiation can be essential for developing targeted therapies."
Recent research in cancer biology emphasizes the need to differentiate between normal and cancerous tissues to create effective treatment strategies. This includes examining pathways of differentiation and determining how certain conditions may predispose tissues to malignancy.
Research Advances in Tissue Science
Research in tissue science has emerged as a pivotal element in understanding human biology and treating diseases. This section will explore recent advances, focusing on aspects that have profound implications for medicine, regenerative therapies, and our comprehension of cellular architecture.
Innovations in Tissue Engineering
Innovations in tissue engineering mark a significant breakthrough in the medical field. This technology combines principles from biology and engineering to create artificial tissues and organs. Researchers utilize scaffolds made of biocompatible materials—like polylactic acid or hydrogels—to support cells as they grow into functional tissues.
- Use of 3D Printing: One groundbreaking technique is 3D bioprinting. This method allows us to print tissues layer-by-layer, closely mimicking the natural structure of actual human tissues.
- Personalized Medicine: Tissue engineering contributes to personalized medicine. Custom tissues can be created using a patient's own cells, reducing risks of rejection and improving integration.
"The ability to engineer tissues opens doors to therapies that were once merely theoretical, including the regeneration of complex organs."
Stem Cell Research and Tissues
Stem cell research has made extraordinary strides in recent years. Stem cells possess the unique ability to develop into various cell types, making them essential in tissue repair and regeneration. Advances in understanding these cells are crucial for enhancing therapies.
- Induced Pluripotent Stem Cells: The discovery of induced pluripotent stem cells, or iPSCs, enables the reprogramming of adult cells into pluripotent cells. This technique permits the generation of patient-specific tissues.
- Clinical Trials: Ongoing clinical trials explore the effectiveness of stem cells in treating degenerative diseases. They may aid recoveries from injuries or illnesses affecting the heart, liver, and nervous system.
Future Directions in Tissue Studies
As research continues, future directions in tissue studies look promising. Increased knowledge will likely lead to novel therapeutic approaches and a deeper understanding of tissue functionality.
- Integration of AI and Machine Learning: Integrating artificial intelligence into research may accelerate discovery in tissue sciences. AI can analyze extensive datasets to identify relationships between cell behavior and tissue function.
- Exploration of Extracellular Matrix: The extracellular matrix plays a critical role in maintaining tissue structure. Future studies aim to better understand its complex interactions, which could lead to improved tissue regeneration strategies.
In summary, advances in tissue science profoundly influence both clinical practices and our comprehension of human biology. The integration of engineering, biology, and technology creates a foundation for future breakthroughs in health and medicine.
The End
The exploration of anatomical tissues serves as a critical foundation in the study of biology and medicine. Understanding tissues not only provides insights into their structure but also emphasizes their dynamic roles within the organism. This article illuminates the classification of tissues, their functional implications, and the intricate relationships they maintain.
Summary of Key Points
In summary, the key points highlighted throughout this article include:
- Classification of tissues: The four primary tissue types—epithelial, connective, muscle, and nervous—each fulfilling distinct yet interconnected roles.
- Histological examination: Various techniques, including staining and microscopy, reveal the complex architecture of tissues, crucial for deeper biological understanding.
- Functional importance: Tissues contribute to mechanical properties, maintain homeostasis, and play vital roles in health and disease.
- Research advancements: Innovations in tissue engineering and stem cell research are paving the way for new therapeutic approaches in medical science.
These points encapsulate the multi-faceted nature of tissue study, underscoring its relevance across various disciplines within biological sciences.
The Ongoing Importance of Tissue Study
The study of tissues continues to hold significant importance for several reasons:
- Medical applications: Knowledge of tissue structure and function aids in diagnosing diseases and developing treatments.
- Research advancements: Ongoing research in tissue engineering and regenerative medicine is crucial for developing solutions for tissue damage and organ failure.
- Education and training: For students and professionals alike, understanding anatomical tissues forms a vital part of the curriculum, fostering future innovations in health sciences.
"Through an in-depth understanding of anatomical tissues, we unlock pathways to effective disease management and pioneering medical breakthroughs."