Exploring the ELISA Test for Protein Detection
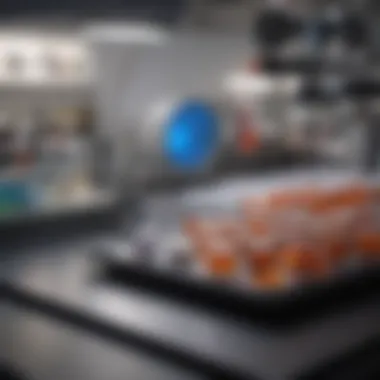
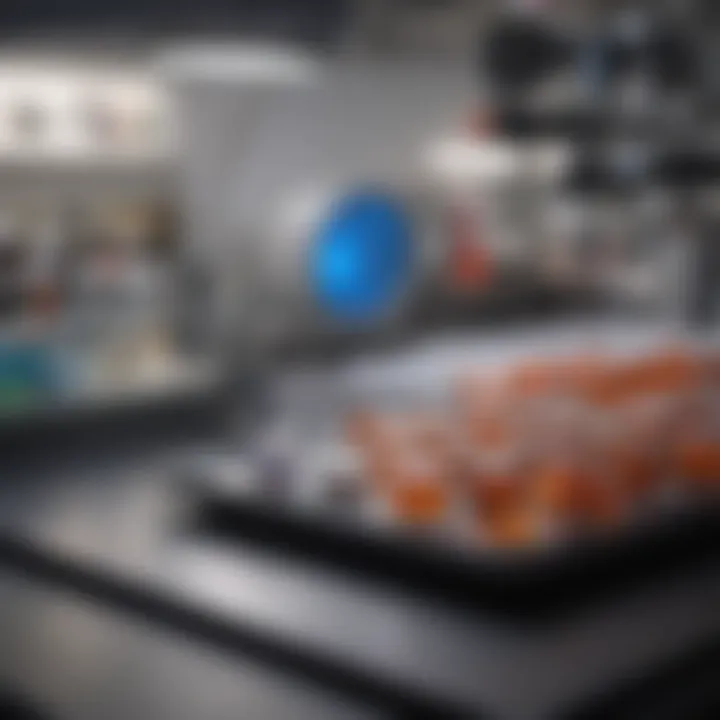
Intro
The Enzyme-Linked Immunosorbent Assay (ELISA) serves as a foundational technique in molecular biology, enabling researchers and clinicians to detect and quantify proteins with precision. Though its origins trace back several decades, the applicability of ELISA continues to expand across diverse fields such as immunology, diagnostics, and biochemical research. Understanding ELISA not only reveals its intricate mechanics but also emphasizes its critical role in enhancing scientific knowledge and clinical decision-making.
This exploration aims to dissect the precise mechanisms behind the ELISA test, highlighting its methodologies, types, and applications. By enriching the reader's comprehension, this piece targets students, educators, and professionals keen on grasping the subtleties of protein assays and their significance in various research contexts.
Methodology
Overview of research methods used
The methodology of the ELISA test hinges on its capacity to bind specific proteins using antibodies. It utilizes passive adsorption and surface chemistry principles to facilitate the adherence of antigens to a solid phase substrate, usually a microplate. Following antibody binding, subsequent detection involves labeling, which may employ enzyme-linked antibodies that generate a measurable signal.
Data collection techniques
Data from ELISA assays is typically gathered through optical density readings. After the enzymatic reaction occurs, a substrate is added that interacts with the product, producing a color change. The intensity of the color correlates with the concentration of the target protein. Standard curves are created to determine the concentration by comparing test results against known standards, ensuring accuracy and reliability of results.
Future Directions
Upcoming trends in research
The increasing sophistication of ELISA technology points to several future directions. Innovations include multiplex assays that can detect multiple proteins simultaneously, enhancing efficiency in both research and clinical diagnostics. Furthermore, the integration of nanotechnology may improve sensitivity and reduce sample sizes needed for analysis.
Areas requiring further investigation
Despite advancements, there remain challenges. Specificity issues, cross-reactivity among proteins, and reproducibility across different laboratory settings are significant concerns that necessitate ongoing research. Addressing these gaps could significantly enhance the ELISA's reliability and breadth of applications.
In summary, the ELISA test is not merely a technique but a robust analytical tool that continues to evolve. Its practical applications in research and clinical environments underscore its value, making understanding its methodology and future trajectories imperative for advancing scientific knowledge.
Foreword to ELISA
The Enzyme-Linked Immunosorbent Assay, commonly known as ELISA, represents a pivotal technique in the realm of protein detection and quantification. Understanding this method is essential for students, researchers, educators, and professionals who rely on accurate protein analysis in their work. ELISAโs significance transcends simple detection; it plays a crucial role in diagnostics, monitoring biological processes, and facilitating research across diverse fields.
Definition and Purpose
ELISA is defined as a quantitative immunoassay that utilizes the specific binding between an antibody and an antigen. The purpose of this assay is to detect or measure the presence of proteins in a sample. This is achieved by using enzyme-linked antibodies that produce a measurable signal, usually a color change. The sensitivity and specificity of ELISA make it an invaluable tool in both clinical diagnostics and laboratory research.
Key purposes of ELISA include:
- Diagnostic applications: Identifying diseases, infections, and allergies by detecting specific proteins in serum or other biological samples.
- Research applications: Assessing protein levels in various experiments to understand biological processes and mechanisms.
Historical Context
The development of ELISA can be traced back to the 1970s, a period marked by significant advancements in biochemical techniques. Initially, the method was introduced as a means to measure antibody concentrations in serum. The first paper describing ELISA appeared in 1971, and since then, the technology has undergone notable progress.
Today, ELISA has become a standard technique in laboratories worldwide. Its ability to process multiple samples simultaneously and produce quantitative data has revolutionized diagnostics and research methodologies. The evolution of ELISA comprises various formats, each tailored to specific applications.
"ELISA has placed a profound impact on the landscape of laboratory medicine, significantly enhancing the ability to diagnose and study diseases."
In summary, the introduction to ELISA sets the foundation for a deeper discussion on its principles, methodologies, and various applications. Understanding the definition, purpose, and historical context of this assay is critical to fully appreciating its role in science.
Principles of the ELISA Test
The principles underpinning the ELISA test are crucial for its successful application in various scientific fields. Understanding these principles enables researchers and clinicians to appreciate the strengths and limitations of the assay. The quantitative and qualitative aspects of protein detection elevate the need for a firm grasp of how the test operates. This understanding is vital for ensuring accurate results and enhancing the reliability of findings in both research and diagnostic contexts.
Basic Concept
The basic concept of ELISA revolves around the specific binding between antigens and antibodies. This highly selective interaction serves as the foundation for the assay. In a typical setup, a solid phase, usually a microtiter plate, is coated with the antigen of interest. When a sample containing antibodies is added, they bind specifically to the coated antigen. After washing away unbound substances, a secondary, enzyme-linked antibody is introduced. This step amplifies the signal. The resulting enzyme-substrate reaction produces a measurable change, usually a color change. This indicates the presence and amount of the antibody.
This fundamental principle allows for high sensitivity and specificity, making ELISA a preferred method in many applications.
Antigen-Antibody Interaction
The antigen-antibody interaction is at the heart of any ELISA test. This interaction is both specific and high-affinity, key characteristics that distinguish it from other methods. Antibodies recognize unique epitopes on the antigen, leading to a strong binding event.
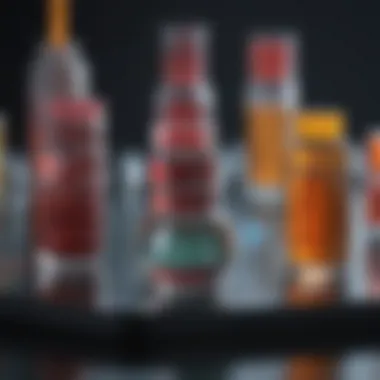
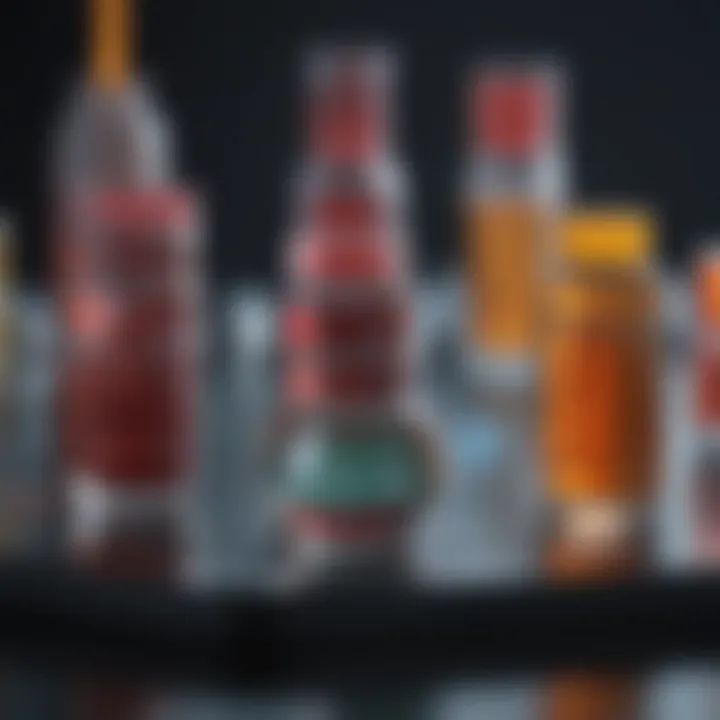
Different types of ELISA involve various approaches to harness these interactions. For instance, in direct ELISA, the target antigen binds directly to the plate, while in sandwich ELISA, an antibody captures the antigen before it is detected by another antibody. Understanding these interactions informs best practices for assay design, reagent selection, and interpretation of results. Successful antigen-antibody interactions are central to obtaining reliable and reproducible data.
"The specificity of the antigen-antibody interaction is what makes ELISA a powerful tool in both clinical diagnostics and research settings."
Types of ELISA Assays
Understanding the various types of ELISA assays is pivotal for anyone engaged in biochemical research, diagnostics, or related fields. Each ELISA type has unique features that suit different applications. By learning these distinctions, researchers can choose the most appropriate assay design for their specific needs. Different assays offer varied sensitivity levels, throughput capabilities, and specificities. This section delves into the intricacies of each ELISA type, providing clarity on their functions and advantages.
Direct ELISA
Direct ELISA is one of the simplest forms of the assay. This format involves coating the wells of a microplate with the antigen of interest. Following the coating, a primary antibody, which is labeled with an enzyme, is added. The detection process relies on a substrate that reacts with the enzyme to produce a measurable signal, typically a color change.
This assay is beneficial when the goal is to detect a particular protein without any interference from other entities. The direct nature allows for a quick methodology. However, there are limitations, such as potential issues with sensitivity; if the antigen is present in low concentrations, the results may not be as detectable when using this method.
Indirect ELISA
In contrast, indirect ELISA employs a two-step labeling technique. Initially, the target antigen is coated onto the microplate. A primary antibody that is not labeled is added next, allowing it to bind to the target antigen. This is followed by a secondary antibody that carries the enzyme label and binds specifically to the primary antibody.
This method enhances the sensitivity of the assay considerably since multiple secondary antibodies can bind to a single primary antibody. Therefore, this assay is particularly useful for detecting proteins present in low concentrations. However, it can introduce added variability due to the presence of multiple antibodies, which could lead to non-specific binding.
Sandwich ELISA
Sandwich ELISA is distinguished by its dual binding strategy. This assay begins with the coating of a microplate with a capture antibody specific to the target antigen. After washing, the sample containing the antigen is added. The antigen binds to the capture antibody. Subsequently, a second, detection antibody is introduced. This pair of antibodies, integrated with an enzyme label, is crucial for the sandwich format.
This method is particularly effective for complex samples, such as serum or plasma, where non-target proteins might interfere with the results. Sandwich ELISA excels in specificity and sensitivity, making it suitable for quantifying low-abundance proteins. However, careful optimization of antibodies is required to prevent interference and ensure efficiency.
Competitive ELISA
In competitive ELISA, a measurable amount of antigen competes with the sample antigen for binding to a specific antibody. The microplate is coated with the antibody before the addition of both the labeled antigen and sample. In this scenario, the more target antigen is present in the sample, the less labeled antigen will bind. The resulting signal correlates inversely with the concentration of the target antigen.
This method is advantageous when the available antibodies are limited or when analyzing small molecules, such as hormones or drugs. However, competitive ELISA may result in less sensitivity compared to other methods, and quantifying results may require a more complex interpretation of the data.
Selecting the appropriate ELISA type hinges on understanding the specific context and requirements of the study or diagnostic application.
ELISA Methodology
The methodology behind the ELISA test is crucial to understand. It encompasses various steps that ensure the accuracy and reliability of results. Each step is methodically designed to minimize errors and enhance detection capabilities. By comprehending these components, one can appreciate the test's robustness and its wide application in scientific inquiries.
Sample Preparation
Sample preparation is the initial and crucial step in ELISA methodology. Proper sample handling influences the integrity of the proteins to be analyzed. Samples may come from different sources including blood, serum, or even cell cultures. It's essential to keep samples at optimal temperatures and avoid prolonged exposure to unsuitable conditions. This ensures that the proteins maintain their structure and functionality. Additionally, using appropriate diluents can improve the concentration and presence of the target protein, which is vital for accurate quantification during testing.
Plate Coating
Once samples are ready, the next step is plate coating. In this process, the wells of the ELISA plate are coated with the antigen or antibody of interest. The choice of the coating agent depends on the type of ELISA being performed. It's important to achieve uniform coating to ensure consistent binding. Too low or too high concentrations can result in poor detection limit. After coating, the plate needs to be incubated to allow sufficient binding time. This step is often performed at 4ยฐC overnight, which helps in stabilizing the interactions.
Blocking Step
Following plate coating, a blocking step is undertaken. This aims to prevent non-specific binding of antibodies in later stages. Blocking solutions usually contain proteins like BSA or non-fat milk. They inhibit the binding sites that are not occupied by the coated antigen. The result is a reduction in background noise, leading to a clearer signal for the target protein. Selecting the right blocker is vital as it should not interfere with the specific interaction being measured.
Detection
Detection is arguably the most critical part of the ELISA methodology. It determines how the results are interpreted and analyzed. Two essential components of this phase are enzyme labeling and substrate reaction.
Enzyme Labeling
Enzyme labeling involves attaching an enzyme to the antibody. Common enzymes used are horseradish peroxidase and alkaline phosphatase. This labeling is beneficial due to the enzyme's ability to produce a measurable signal after the addition of a substrate. A key characteristic of enzyme labeling is its sensitivity; even minute amounts of proteins can generate detectable signals. However, one must be cautious of potential interference from the sample matrix that could affect enzyme activity. Overall, enzyme labeling is a popular choice due to its ability to amplify signals effectively, which is crucial in protein detection.
Substrate Reaction
The substrate reaction occurs post-enzyme labeling. This step involves adding a substrate that the enzyme will convert into a detectable signal, usually a color change. The substrate must be carefully selected to elicit a strong response without excessive background signals. One unique feature of substrate reactions is that they offer real-time quantification. This property is advantageous, as the intensity of the resulting signal can be measured against known standards. However, the timing is critical; overly long incubation with the substrate may lead to saturation and inaccurate readings. Hence, monitoring the reaction is essential for achieving reliable results.
Factors Influencing ELISA Results
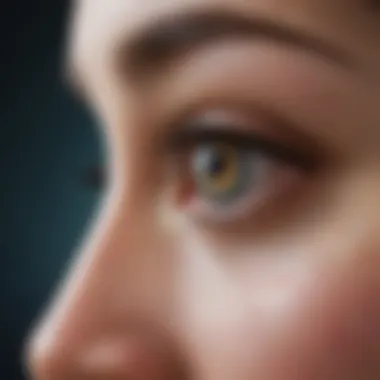
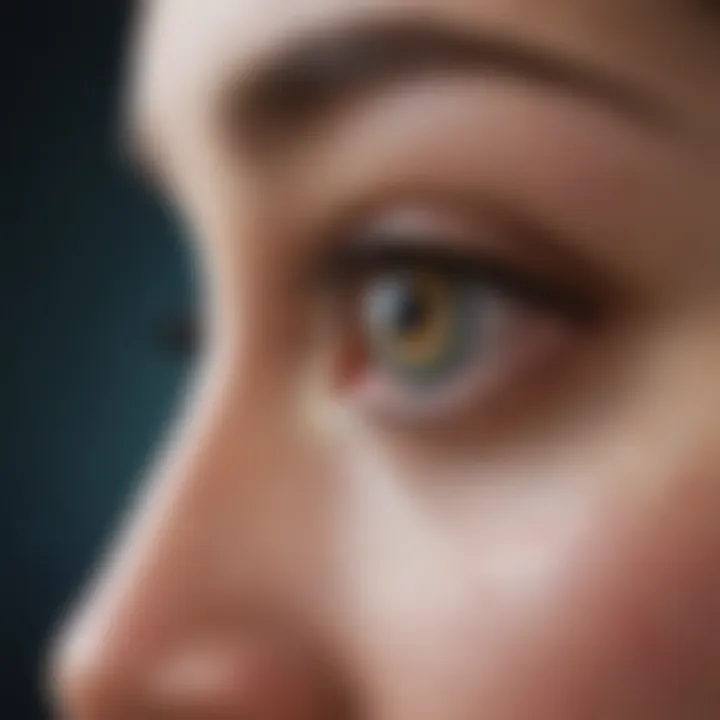
The performance of an ELISA test is not only a reflection of its underlying methodology but is highly dependent on various influencing factors. Understanding these factors is crucial for ensuring accurate and reliable results. The quality of reagents, the conditions under which the test is performed, and the duration of incubation all play significant roles in assay outcomes. Each of these elements must be considered carefully to ensure that the results reflect true biological conditions rather than artifacts of the testing process.
Reagent Quality
Reagent quality is often the backbone of a successful ELISA. Poor quality reagents can lead to high background noise, weak signals, or contradictory results. The primary reagents in an ELISA include antibodies, antigens, and substrates. Using proteins that have been validated through rigorous quality controls is essential.
- Antibodies: Monoclonal and polyclonal antibodies differ considerably. Monoclonal antibodies provide specificity, while polyclonal antibodies can enhance signal strength. Both should be sourced from reputable suppliers.
- Antigens: The integrity of the antigen used, including its purity and concentration, impacts the binding efficiency. A well-characterized antigen provides more accurate results.
- Enzymes and substrates: The enzymatic labels and substrates must be compatible. For example, horseradish peroxidase is a common enzyme that must be paired correctly with its substrate for effective signal generation.
In summary, careful selection and validation of reagents help mitigate variability and improve the reliability of test results.
Temperature and pH
Controlling temperature and pH is crucial for maintaining optimal conditions during the ELISA process. Both of these factors influence enzymatic reactions and antigen-antibody binding kinetics. Striking the right balance can mean the difference between a successful assay and significant experimental errors.
- Temperature: Typical incubation temperatures range from 4ยฐC to 37ยฐC. Each temperature affects enzyme activity. Raising the temperature can increase reaction speeds, but high temperatures may denature proteins. Maintaining a stable temperature throughout the experiment is key.
- pH: Most antibody-antigen interactions are optimally performed at a particular pH. For instance, a neutral pH is generally favorable. Deviations from the ideal pH range can hinder binding efficiency, resulting in lower sensitivity or accuracy.
Careful monitoring of these parameters is essential during each stage of the assay to minimize variability that could compromise the results.
Incubation Time
Incubation time directly relates to the effectiveness of protein binding in ELISA. Too short of an incubation period may result in insufficient binding of proteins, while excessive incubation can lead to increased background noise due to non-specific binding.
- Optimization: Specific incubation times depend on the assay design, but generally, longer incubation times can enhance signal strength. However, they also risk increasing non-specific interactions.
- Stepwise adjustment: Therefore, it is beneficial to optimize incubation times for each specific assay context, bearing in mind the complexity of the sample and the specific proteins of interest.
By adopting a methodical approach to reagent quality, temperature, pH, and incubation time, one can significantly increase the reliability and accuracy of ELISA results. This attention to detail is vital for students, researchers, educators, and professionals who aim for rigorous scientific standards.
Quantification Methods in ELISA
Quantification methods in ELISA are pivotal for the assayโs accuracy and reliability. These methods allow researchers to determine the concentration of proteins in samples, which is essential for both clinical diagnostics and research applications. High precision in quantifying protein levels can lead to better understanding of disease mechanisms, effective diagnosis, and treatment monitoring. Thus, grasping the quantification methods helps in utilizing ELISA more effectively.
Standard Curve
The standard curve is a foundational element in the quantification process of ELISA. This curve is generated by plotting known concentrations of the target protein against their corresponding optical density measurements from the assay. A standard curve allows for the estimation of unknown sample concentrations based on their optical density.
To establish a standard curve, one typically performs the following steps:
- Prepare serial dilutions of the known concentrations of the target protein.
- Run these dilutions through the ELISA protocol as if they were samples.
- Measure the absorbance for each dilution.
- Plot the values to generate a curve, often displayed in a linear or semi-logarithmic scale.
The standard curve is crucial as it enables quantitative analysis, offering a point of reference to compare unknown samples. Precision in the curve influences the quantification accuracy, and irregularities can lead to significant errors in protein concentration assessments.
Controls
Controls are integral to ensuring the validity of the ELISA results. There are broadly two types of controls: positive controls, which contain a known amount of the target protein, and negative controls, which lack the protein. Both types serve to establish a baseline for interpreting the test results and to identify any anomalies that could compromise the assayโs reliability.
Key points regarding the use of controls include:
- Positive controls confirm that the assay is functioning correctly. They provide a benchmark against which to measure the samples.
- Negative controls help in determining the specificity of the assay. They reveal any background signals or cross-reactivity issues that might arise.
- Regular inclusion of controls mitigates the risk of false positives or negatives, thereby enhancing reproducibility.
Applications of ELISA
Enzyme-Linked Immunosorbent Assays, commonly known as ELISA, play a pivotal role in various fields, particularly in clinical diagnostics, research and development, and environmental testing. The versatility of ELISA makes it a critical tool for scientists and healthcare professionals alike. By providing a mechanism to detect and quantify specific proteins and antibodies in different samples, ELISA enhances our ability to diagnose diseases, monitor health, and understand complex biological processes.
Clinical Diagnostics
Clinical diagnostics is perhaps the most prominent application of ELISA. The assay is frequently employed to detect and quantify biomarkers for diseases, such as infections, autoimmune disorders, and cancers. For instance, HIV testing often relies on ELISA to measure antibodies against the virus in patient serum. The sensitivity of ELISA means it can detect even low concentrations of antibodies, thus enabling early diagnosis and timely intervention.
In many clinical settings, the rapid turnaround time and the ability to process multiple samples simultaneously make ELISA an efficient choice. Its cost-effectiveness is another factor contributing to its widespread use. However, it is important to validate the results with confirmatory tests, as false positives or negatives can have significant clinical implications.
Research and Development
The application of ELISA in research and development is vast and considerable. Researchers frequently utilize ELISA for various purposes, including evaluating drug efficacy, studying disease mechanisms, and developing new therapeutics. For example, scientists can use ELISA to measure the levels of specific cytokines in a sample to understand immune responses in clinical trials.
Furthermore, in vaccine development, ELISA assists in the assessment of immune responses by quantifying antibodies generated in response to vaccination. The ability to customize ELISA assays makes it a flexible tool, catering to different research needs across various disciplines, such as molecular biology, biochemistry, and pharmacology. Its capability to generate reliable quantitative data is invaluable in advancing scientific knowledge.
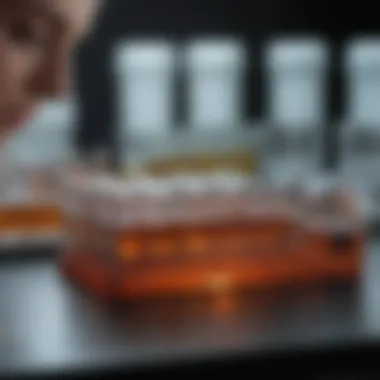
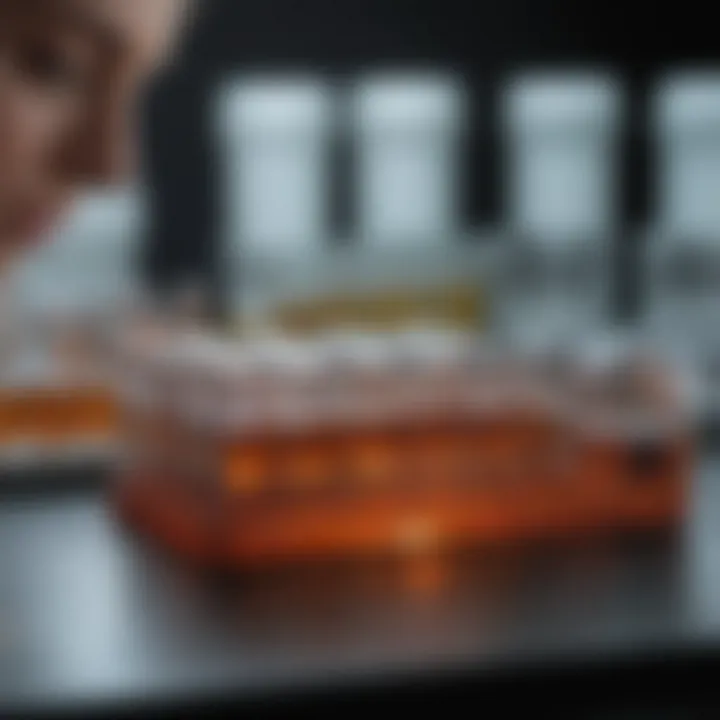
Environmental Testing
Environmental testing is another significant area where ELISA finds its application. The assay is utilized to detect various proteins or toxins present in environmental samples, such as water, soil, and air. For instance, ELISA can be employed to monitor pollutants, including pesticides, heavy metals, and toxins produced by microorganisms.
This application is particularly critical for ensuring public health and environmental safety. By enabling the quick and accurate detection of harmful substances, ELISA supports regulatory compliance and helps in assessing the impact of human and industrial activities on ecosystems. The ability to perform these tests in situ further enhances the practical appeal of ELISA in environmental science.
"The adaptability of ELISA across multiple domains highlights its significance as a research tool and a diagnostic method."
In summary, the applications of ELISA are extensive and impactful. By delivering reliable data across clinical diagnostics, research, and environmental safety, ELISA serves as a crucial component in the ongoing efforts to advance health care and scientific understanding. Its continued evolution will likely yield further innovations and improvements in its methodology, solidifying its importance in these fields.
Advantages and Limitations of ELISA
The Enzyme-Linked Immunosorbent Assay offers a wealth of benefits that make it a preferred choice in many laboratories. Understanding these advantages is essential for anyone engaged in protein research or clinical diagnostics. However, it is equally important to recognize the limitations that can influence the outcome of tests. This section outlines both aspects to provide a balanced view of the ELISA test.
Advantages
ELISA has several advantages that contribute to its widespread adoption in various fields:
- Sensitivity: ELISA tests can detect low concentrations of proteins, making them suitable for early diagnosis of diseases.
- Specificity: By utilizing specific antibodies, ELISA effectively targets the protein of interest, reducing background noise from other substances in the sample.
- Quantitative Results: ELISA provides numerical data that can indicate the exact concentration of proteins in a sample, crucial for many research applications.
- High Throughput Capability: Many ELISA tests are designed for automation, allowing multiple samples to be processed simultaneously. This increases efficiency in laboratory settings.
- Versatile Applications: ELISA can be used for a wide array of proteins, including hormones, cytokines, and antibodies. This versatility enhances its utility across various research and clinical domains.
- Cost-Effective: Compared to some other analytical methods, ELISA is generally more cost-effective, especially when considering the availability of reagents and materials.
- Ease of Use: The straightforward protocol simplifies the testing process, making it accessible for labs with varying levels of expertise.
"The flexibility of ELISA to adapt to different types of proteins and contexts amplifies its significance in diagnostics and research."
Limitations
While the advantages are substantial, there are notable limitations to consider when using ELISA:
- Cross-Reactivity: Non-specific binding can lead to false positives. This requires careful selection and validation of antibodies used in assays.
- Matrix Effects: Components present in biological samples can interfere with the assay, skewing results and necessitating sample purification prior to testing.
- Limited Range: Some ELISA kits are designed for a narrow range of protein concentrations, which may not be suitable for all samples.
- Time-Consuming: Although the ELISA protocol is relatively simple, the overall procedure can be time-consuming, especially when preparing samples and incubation times are considered.
- Storage Stability: Antibodies and reagents used in ELISA can lose effectiveness over time, which could affect test outcomes if not stored properly.
- Dynamic Range: ELISA may struggle with samples containing extremely high concentrations of target proteins, leading to saturation and inaccurate quantification.
Future Directions in ELISA Research
The field of Enzyme-Linked Immunosorbent Assay (ELISA) is continuously evolving, prompting researchers and practitioners to consider future directions for the methodology. ELISA's established role in protein detection and quantification cannot be overstated, yet the thirst for more efficient and effective diagnostic tools drives an array of innovative research efforts. This section will explore aspects such as innovative developments and potential enhancements that form the crux of future ELISA applications.
Innovative Developments
Recent advancements in technology are redefining how ELISA assays are conducted. Automation stands out as a key factor, streamlining processes that were once labor-intensive. The integration of robotics in high-throughput screening allows for the simultaneous analysis of many samples, which improves efficiency in both clinical and research settings.
Another innovative development is the emergence of multiplex ELISA. This technique allows for the simultaneous measurement of multiple analytes within the same sample, significantly saving time and resources. By utilizing specific antibodies for various proteins, researchers can collect more data from a single sample, enhancing the robustness of experimental results.
Moreover, there has been increasing interest in developing point-of-care (POC) ELISA tests. These tests are designed for use outside traditional laboratories, bringing diagnostic capabilities directly to patients. This shift could drastically change the landscape of medical diagnostics, especially in low-resource settings.
Potential Enhancements
Enhancing the sensitivity and specificity of ELISA is vital for addressing current limitations. New labeling strategies using advanced fluorescent dyes or nanoparticles show promise in improving detection limits. Such innovations could allow for the identification of proteins at very low concentrations, which is crucial in early disease detection.
Development of novel substrates that match the characteristics of specific enzymes can also refine the assay's performance. This is important as the initial enzyme-substrate interaction is a critical step influencing the overall sensitivity of the ELISA method.
Furthermore, advancements in assay design, such as microfluidic systems, merit attention. These systems can reduce sample volumes and enhance reaction kinetics, leading to more efficient assays. The miniaturization of conventional ELISA could open doors to more accessible and user-friendly diagnostic tests.
"The possibilities for future developments in ELISA techniques are vast, with advances that transform how protein assays are conducted and interpreted."
As researchers and clinicians continue to collaborate, the future of ELISA appears promising. By embracing these innovative developments and potential enhancements, the methodology can evolve to meet growing challenges in diagnostics while maintaining its essential role in protein detection.
End
The conclusion of this article encapsulates the crucial role of the ELISA test within scientific research and clinical diagnostics. It facilitates a reliable method for the detection and quantification of proteins, showcasing its versatility across diverse fields.
Summary of Key Points
To highlight the essential areas covered, we can summarize that:
- The ELISA method is paramount for protein analysis, enabling high specificity and sensitivity.
- Various types of ELISA assays, including direct, indirect, sandwich, and competitive formats, serve distinct purposes based on the requirements of the study or diagnostic goal.
- The methodology involves key steps such as sample preparation, plate coating, blocking, and detection, each influencing the final results.
- Understanding factors that influence ELISA results, like reagent quality and incubation times, is essential to ensure accuracy and reproducibility.
- The applications of ELISA span clinical diagnostics, research, and environmental testing, making it applicable for researchers and medical professionals alike.
- Despite its advantages, such as high throughput and ease of use, limitations require consideration to mitigate any potential errors.
Importance of ELISA in Modern Science
The significance of the ELISA test in modern science cannot be overstated. It provides a pathway for advancements in clinical diagnostics, especially concerning infectious diseases and autoimmune disorders. In research contexts, it supports the study of biomarkers and drug development, playing an instrumental role in translational medicine.
Additionally, its ability to provide quantifiable data helps in establishing correlations between proteins and various biological states. This data is vital for understanding pathophysiological processes and developing targeted therapies.
Research continuously evolves, and in the landscape of precision medicine, the sophistication and adaptability of the ELISA will be pivotal. As scientists strategize to enhance assay performance further, the quest for innovations in sensitivity, multiplexing capabilities, and throughput ensures that ELISA stays relevant.
In sum, the history, development, and future potential of ELISA underscore its foundational role in scientific inquiries and healthcare. Ensuring accuracy, standardization, and continual advancement will be paramount as the scientific community relies on this elegant tool.