The Dynamics of Electronic Cells: Structure and Functionality
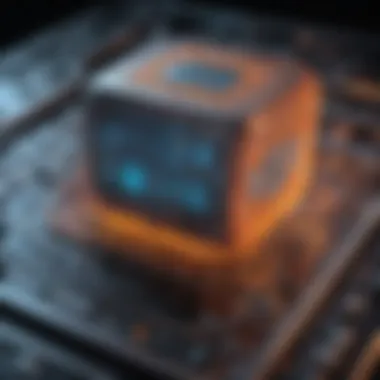
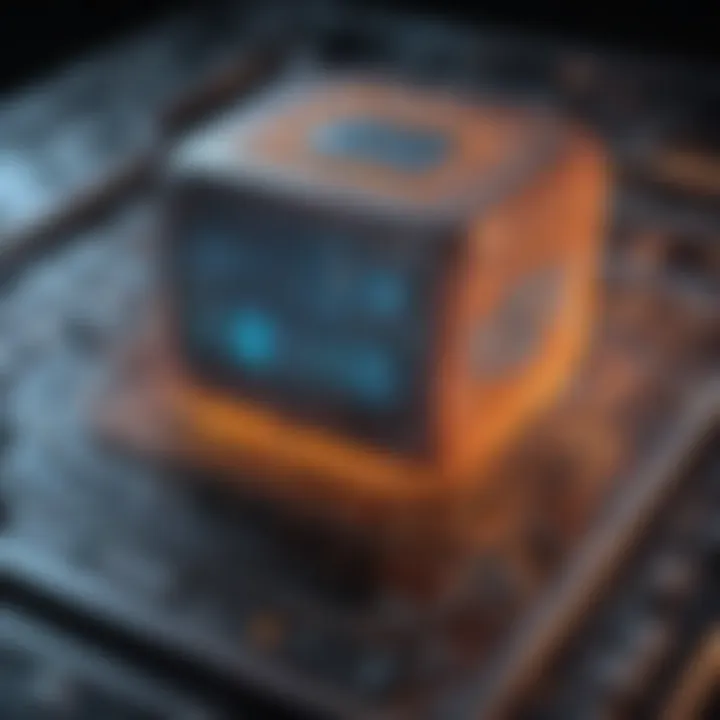
Intro
In recent years, the study of electronic cells has gained significant attention in scientific communities. These cells, which integrate electronic components within biological or chemical systems, present innovative solutions in various fields, from energy storage to biomedical devices. Understanding their intricate structure and functionality is essential for harnessing their full potential. This article intends to provide a comprehensive overview of electronic cells, delving into the principles that govern their operation and examining their practical applications.
The scope of this exploration engages students, researchers, educators, and professionals alike. They will find a detailed account of how electronic cells function, their diverse uses, and the impact they yield in modern research contexts. By connecting theoretical frameworks with real-world applications, the discussions will underscore the relevance of electronic cells in contemporary scientific endeavors.
Methodology
Overview of research methods used
The approach in studying electronic cells typically involves a blend of experimental and analytical methods. Researchers often utilize both qualitative and quantitative techniques to explore various aspects of these cells. This includes theoretical modeling, computational simulations, and experimental validations.
In many studies, microfabrication techniques are employed to create precise electronic cell components, allowing for a detailed exploration of their functionalities. This hands-on approach enables comparison with theoretical predictions, ensuring a more robust understanding of the dynamics at play.
Data collection techniques
Data about electronic cells are collected using various methods, including:
- Spectroscopic techniques: Techniques like infrared and UV-Vis spectroscopy help in analyzing the materials and their reactions.
- Electrical characterization: This method quantifies the electrical properties of electronic cells, shedding light on performance metrics.
- Imaging techniques: Methods such as electron microscopy provide insight into the structural aspects of electronic cells at a micro or even nano scale.
These techniques allow for a comprehensive view, revealing how structure influences functionality and vice versa.
Future Directions
Upcoming trends in research
The field of electronic cells is rapidly evolving, with promising trends emerging. Some noteworthy areas include:
- Integration with artificial intelligence: AI has the potential to optimize the design and performance of electronic cells, leading to advanced applications.
- Sustainable materials: Research is focusing on using renewable resources to create electronic cells, reducing environmental impact.
Areas requiring further investigation
Despite the progress made, several areas remain underexplored. These include:
- Scalability of production: Moving from lab-scale to large-scale production presents unique challenges that need to be addressed.
- Long-term stability: Understanding how electronic cells perform over extended periods is crucial for real-world applications.
Continued research is essential to unravel these complexities, driving innovation in the field and unlocking new possibilities.
Prolusion to Electronic Cells
Electronic cells play a crucial role in modern technology. Their importance spans various scientific fields, impacting energy storage and management, biomedical applications, and environmental monitoring. Understanding electronic cells is essential for appreciating their functionality and real-world applications. They are the backbone of many devices and systems that define contemporary life, from batteries in mobile phones to sensors in environmental studies.
Definition and Overview
Electronic cells, also known as electrochemical cells, convert chemical energy into electrical energy through electrochemical reactions. At their core, they consist of two electrodes: an anode and a cathode, separated by an electrolyte that facilitates ion movement. This movement is integral, as it enables the flow of electric current. The simplest definition of an electronic cell is that it is a unit that generates electric power from chemical reactions.
Key characteristics of electronic cells include:
- Types: There are primarily two types: primary cells and secondary cells. Primary cells are non-rechargeable, while secondary cells can be recharged.
- Components: Components such as electrodes, electrolytes, and separators determine the efficiency and functionality of the cell.
- Applications: Their applications include powering devices, storing energy, and playing vital roles in medical technologies, among others.
Historical Development
The history of electronic cells is rich and varied, dating back to the early 19th century. The initial exploration into what would become electronic cell technology began with Alessandro Volta, who invented the voltaic pile in 1800. This stack of alternating plates, made of zinc and copper, was the first practical method of generating electric current.
Subsequent developments marked significant advancements:
- Daniell Cell (1836): This was an improvement over Volta's design, introducing a more stable process through a more efficient electrolyte.
- Lead-Acid Battery (1859): Invented by Gaston Plante, this type remains widely used today.
- Nickel-Cadmium Battery (1899) and later innovations further established the path for modern batteries. The development of lithium-ion cells in the late 20th century marked a pivotal point, as these cells became essential in portable electronic devices.
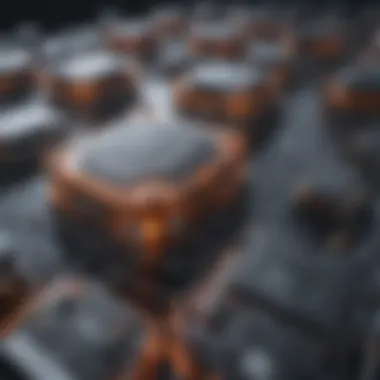
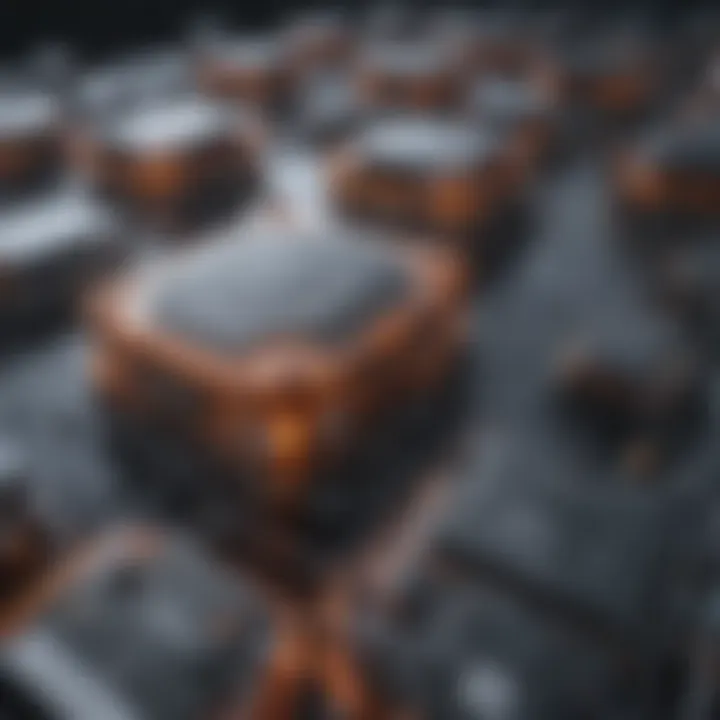
Understanding this historical context enriches oneโs comprehension of electronic cells. Each innovation built on previous knowledge, paving the way for the advanced electronic cells we use today. The evolution reflects ongoing research and dedication to improving energy efficiency and sustainability.
Fundamental Principles of Electronic Cells
Understanding the fundamental principles of electronic cells is crucial for grasping how these devices operate. They serve as the backbone for energy storage and conversion technologies. This insight ties together theory and application, ensuring one comprehends both function and importance. The principles involve physics and chemistry that govern energy transformation processes, thereby impacting various applications from consumer goods to industrial functions.
Working Mechanisms
Electronic cells, often referred to as batteries or fuel cells, work on specific electrochemical processes. These mechanisms can be distilled into two principal reactions: oxidation and reduction. During discharging, oxidation occurs at the anode, where electrons are released. This reaction generates an electric current. Simultaneously, at the cathode, a reduction reaction takes place, consuming those electrons. The electrolyte facilitates ion movement between the electrodes, essential for maintaining the flow of electricity.
The conversion efficiency and energy output hinge significantly on the design and materials used in these processes. Innovations in both have propelled advancements in various sectors, indicating the critical nature of these underlying mechanisms.
Key Components
Key components of electronic cells include electrodes, electrolytes, and separators. Each of these parts plays a vital role in the overall performance and efficiency of the cell.
Electrodes
Electrodes are fundamental to the operation of electronic cells. They serve as the sites for electrochemical reactions. Typically, these are made from conductive materials such as carbon, which provide a robust electrical conduction pathway. One of the key characteristics of electrodes is their surface area; larger surface areas allow for more reactions, enhancing cell performance.
A notable feature of modern electrodes is their ability to be tailored for specific applications, including lithium-ion technology in consumer electronics. However, they have drawbacks. The rapid cycling can lead to degradation over time, impacting longevity and capacity. Thus, while they are beneficial, careful consideration is essential.
Electrolyte
The electrolyte in an electronic cell is crucial for ion transport. It serves as a medium that allows ions to flow between the electrodes. Common choices include liquid solutions or solid-state materials, depending on the cell type. A key characteristic of electrolytes is their ionic conductivity. High conductivity minimizes energy losses, which is desirable for efficiency in devices.
A distinct advantage of modern electrolytes, particularly in lithium-ion cells, is their ability to retain stable performance across a range of temperatures. However, some electrolytes can pose safety risks due to volatility, which is a significant concern in high-capacity applications. Therefore, ongoing research is necessary to improve safety profiles.
Separator
Separators are essential for the function of electronic cells as they prevent direct contact between electrodes while allowing ionic movement. These components typically consist of porous materials, which facilitate the flow of ions. A key characteristic of separators is their mechanical strength and thermal stability.
One unique feature of separators is their role in enhancing cell safety. In modern applications, separators can be engineered to shut down electric flow once temperatures exceed safe limits, reducing the risk of thermal runaway. Nevertheless, some separators may lose effectiveness over time or under extreme conditions, leading to performance loss. This aspect requires ongoing development to increase their reliability.
"Understanding the integral components of electronic cells is not just academic; it is essential for driving innovation in energy technology."
In summary, the fundamental principles of electronic cells provide the necessary knowledge to appreciate their design and functionality. Moving forward, understanding these components and their interactions will be vital for addressing current challenges and advancing technology in energy storage.
Types of Electronic Cells
Understanding the types of electronic cells is crucial in the broader discussion of their structure and functionality. Each category offers distinct benefits, applications, and considerations that shape their use in various fields. This section delineates three primary types of electronic cells: primary cells, secondary cells, and fuel cells. Each plays a vital role in both existing technologies and emerging innovations.
Primary Cells
Primary cells are designed for single-use applications. They generate electrical energy through irreversible chemical reactions. These cells are often used in devices where the demand for power is intermittent or does not require rechargeable capabilities. Common examples include alkaline batteries, lithium batteries, and zinc-carbon batteries.
The key benefits of primary cells are their long shelf life and the convenience they provide. They are widely available and relatively inexpensive, making them suitable for household gadgets, remote controls, and childrenโs toys. However, primary cells do have limitations. Once depleted, they cannot be recharged, creating waste and raising environmental concerns.
Secondary Cells
Secondary cells, or rechargeable batteries, present a more sustainable option compared to primary cells. These cells can be charged and discharged multiple times. Common types include nickel-cadmium, nickel-metal hydride, and lithium-ion batteries. The latter has gained prominence due to its higher energy density and efficiency, making it the preferred choice for portable electronics and electric vehicles.
The major advantage of secondary cells is their longevity, which translates to less frequent replacements and reduced waste. However, they also have downsides. They typically have a higher initial cost and can suffer from capacity fading over time, especially if not maintained properly. Therefore, understanding the correct usage and charging practices is essential to maximize their lifespan.
Fuel Cells
Fuel cells represent a different approach to energy storage and conversion. They generate electricity through electrochemical reactions, typically involving hydrogen and oxygen. The byproducts are usually water and heat, making fuel cells an environmentally friendly option for energy generation.
Fuel cells have various applications, especially in transportation and stationary power generation. They are seen as a key technology for hydrogen-powered vehicles. The main benefits include high efficiency and low emissions, contributing to reduced greenhouse gas levels. However, fuel cells face significant challenges, including high costs related to materials and infrastructure, as well as the lack of widespread hydrogen production methods.
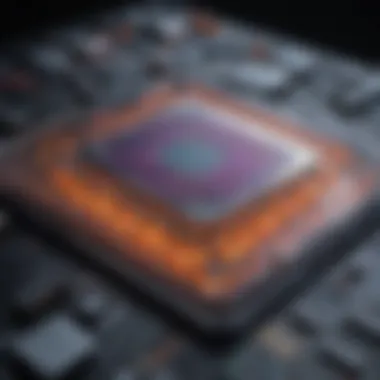
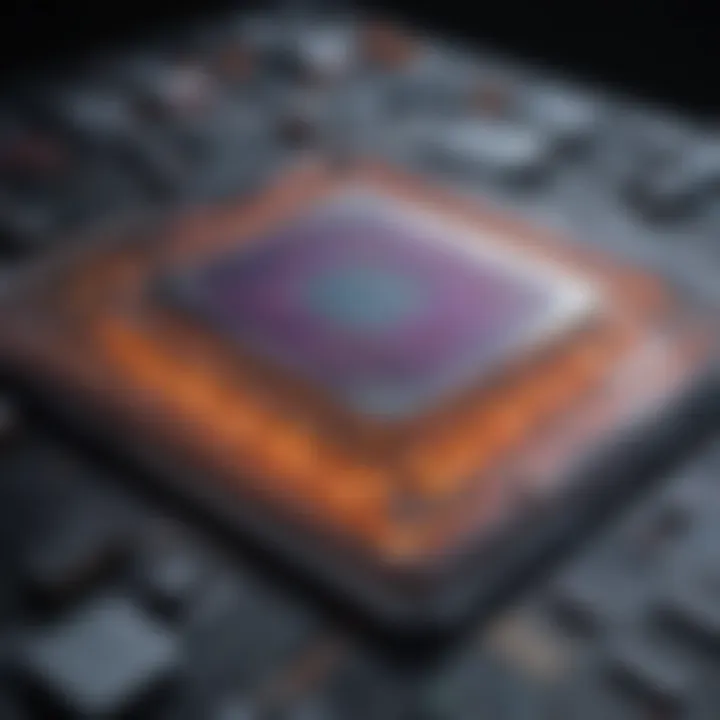
"As technology advances, understanding the different types of electronic cells and their applications will become increasingly relevant for future energy solutions."
Applications of Electronic Cells
The applications of electronic cells are far-reaching and essential in todayโs technologically driven society. They serve as the backbone for numerous devices and systems that enhance efficiency, convenience, and health. Understanding their applications helps in recognizing the importance of electronic cells in various sectors, particularly in energy storage, healthcare, and environmental management.
Energy Storage Solutions
Electronic cells play a crucial role in energy storage solutions. They are integral to systems like rechargeable batteries, which are essential in consumer electronics and electric vehicles. These cells store energy and release it when necessary, ensuring that devices operate smoothly.
- Types of Energy Storage: The two primary types of electronic cells for energy storage are lithium-ion cells and lead-acid batteries. Lithium-ion cells are favored for their energy density and longevity, while lead-acid batteries are widely used in vehicles due to their lower cost.
- Impact on Renewable Energy: Electronic cells are vital in integrating renewable energy sources. They store excess energy generated from solar panels or wind turbines, providing a stable energy supply even when demand peaks or generation dips.
The efficiency of electronic cells can significantly influence the overall performance of energy systems. Advances in this sector will likely enhance energy sustainability, making electronic cells a focal point for future research and innovation.
Biomedical Applications
In the field of biomedicine, electronic cells are transforming patient care and monitoring. They are found in devices like pacemakers, glucose monitors, and drug delivery systems.
- Pacemakers: These small devices help regulate heartbeats by delivering electrical impulses, relying heavily on reliable electronic cells.
- Smart Drug Delivery Systems: Electronic cells enable controlled drug release in response to physiological changes, improving treatment outcomes for patients.
The ability to miniaturize electronic cells has enabled the development of portable devices, offering convenience and real-time data to healthcare professionals. This opens pathways for enhanced patient management and personalized medicine, marking significant advancements in healthcare technology.
Environmental Monitoring
Electronic cells have applications in environmental monitoring by powering devices used to track pollution and ecosystem health. Their ability to provide sustained energy is vital for remote and continuous monitoring solutions.
- Pollution Sensors: Electronic cells power sensors that detect air and water quality, helping to provide data to combat environmental issues.
- Wildlife Tracking: They are also used in tracking devices for wildlife conservation efforts. By powering tracking collars, electronic cells enable researchers to monitor animal movement and behavior in their natural habitats.
With growing concerns for environmental protection, electronic cells contribute significant advancements. This sector not only underlines the applicability of electronic cells but also highlights societal responsibility towards ecological sustainability.
Challenges and Limitations
Understanding the challenges and limitations of electronic cells is critical in optimizing their applications and advancing research in this field. While electronic cells exhibit significant potential across various sectors such as energy storage and medical technologies, they also face serious hindrances that can affect their efficiency, cost, and environmental sustainability. Addressing these challenges is essential to enhance current technologies and innovate future solutions in electronic cell design and integration.
Degradation and Longevity
Degradation is a primary concern for any technology involving electronic cells. Over time, materials used in cells may break down or lose their effectiveness. This leads to a significant decrease in their capacity and overall performance. Most commonly, the electrolyte in lithium-ion batteries suffers degradation due to repeated charge and discharge cycles. Factors like temperature fluctuations, chemical reactions, and mechanical stress further exacerbate this deterioration.
The impact of degradation on longevity results in both increased maintenance costs and potential safety hazards. Hence, researchers must prioritize the development of advanced materials and design protocols that can mitigate degradation effects. For example, solid-state batteries present a potential solution by using a solid electrolyte that could bolster stability and longevity compared to liquid electrolytes used in traditional batteries.
Cost and Accessibility
Another critical issue for electronic cells is their cost and accessibility. High-performance materials, such as nickel, cobalt, and lithium, contribute significantly to the final product cost. The reliance on these materials, especially mined resources, presents challenges like fluctuating prices and geopolitical instability, making the technology sometimes economically unfeasible for widespread use.
In addition, developing economies may find it particularly difficult to access the necessary technology for advancement in electronic cell applications. If these advanced solutions aren't available to all sectors of society, the benefits derived from electronic cells will remain unevenly distributed. More research in alternative materials and cost-effective manufacturing processes is necessary to ensure a fairer access and equitable deployment of this technology.
Environmental Impact
The environmental impact of electronic cells cannot be overlooked. Although these cells play a considerable role in reducing fossil fuel dependence and greenhouse gas emissions when used in renewable energy applications, their lifecycle includes several environmental concerns. The extraction of materials like lithium often leads to habitat destruction and pollution. Moreover, the disposal of spent batteries poses another challenge, as they can release harmful substances into the environment if not managed properly.
Sustainable practices must be adopted to minimize these negative effects. Recycling programs that recover precious metals and materials from used cells can significantly help mitigate environmental hazards.
Future Trends in Electronic Cell Technology
The landscape of electronic cell technology is constantly evolving. Advancements in this field are crucial for multiple applications, such as energy storage and biomedical devices. Future trends focus on enhancing efficiency, reducing costs, and improving the sustainability of electronic cells. Moreover, these trends propel innovation and address the challenges posed by current technologies.
Innovative Materials
New materials are at the forefront of electronic cell development. Researchers are exploring alternatives to traditional materials like lithium and nickel, aiming for improved performance and lower environmental impact. For instance, sodium and magnesium-based cells present a promising frontier. These materials are more abundant and potentially less harmful to the environment. Additionally, solid-state batteries are gaining traction, utilizing solid electrolytes instead of liquid ones. This can lead to increased safety and higher energy densities, making them ideal for various applications.
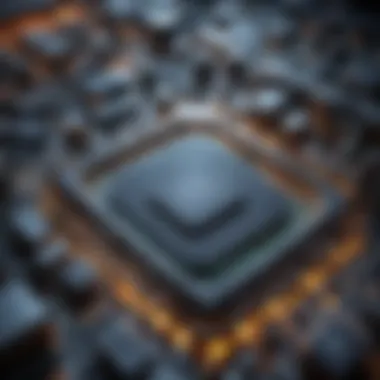
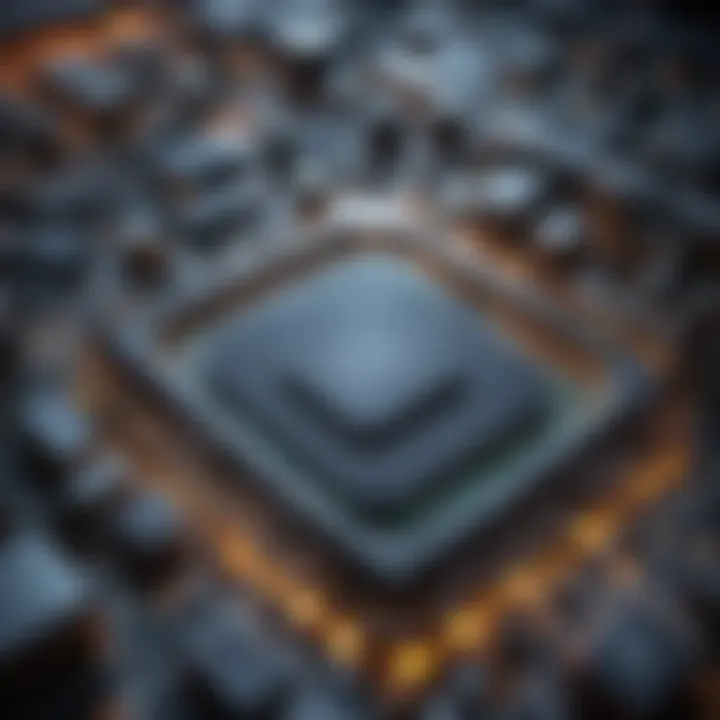
"The push towards using innovative materials is not just about performance; itโs also about sustainability."
Exploring advanced materials often entails trade-offs. While some materials may enhance energy capacity, they might also introduce challenges such as cost or availability. Therefore, ongoing research in material science is vital to find a balance between efficiency and practicality.
Advancements in Design
Design improvements are equally significant for the future of electronic cells. Enhanced cell architecture can maximize performance and durability. Modular designs enable easy replacements and upgrades, ensuring cells remain relevant for longer periods. Furthermore, advancements in nanoscale engineering are optimizing electrode structures, which can dramatically improve charge rates and cycle life. Such sophistication aids in meeting the increasing demand for higher energy storage.
Another design aspect to consider is the integration of smart features. Smart batteries with built-in monitoring systems can provide real-time data on performance and health. This may enhance user experience while extending battery life.
Integration with Renewable Energy Sources
The necessity for integrating electronic cells with renewable energy sources cannot be overstated. As the world transitions to a greener economy, the role of electronic cells becomes critical. Innovations in this area are focused on creating systems that can efficiently store energy generated by solar and wind sources.
Efforts are underway to improve the charging capabilities of electronic cells using renewable energy. For example, developing faster charging technologies can synchronize with peak production times of renewable energy. This integration is essential to facilitate a more sustainable future.
Moreover, seamless interaction between electronic cells and renewable sources can enhance grid stability. Creating a reliable system for energy storage ensures that variable outputs do not disrupt energy supply.
The Role of Electronic Cells in Modern Society
Electronic cells have become foundational in modern society, influencing various aspects of our daily lives. Their significance extends beyond mere power supply; they play a crucial role in enhancing technological advancement and addressing contemporary challenges. This section will unravel the multifaceted impact of electronic cells in different sectors, focusing on consumer electronics, the automotive industry, and aerospace applications.
Consumer Electronics
In the realm of consumer electronics, electronic cells are indispensable. Devices such as smartphones, laptops, and tablets rely on batteries to function. Lithium-ion cells are among the most prevalent in these devices due to their high energy density and ability to recharge multiple times without significant degradation.
Consider the following points:
- Convenience and Portability: The rise of portable devices correlates directly with advances in electronic cell technology. Consumers enjoy the flexibility of using devices on the go, largely attributed to power sources that are lightweight and compact.
- Efficiency: Modern electronic cells feature sophisticated battery management systems that optimize power usage, thereby extending the lifespan of devices. Users can benefit from longer operational times without frequent recharging.
- Sustainability Issues: While electronic cells are essential for consumer devices, they also create waste. The recycling and disposal of spent batteries pose environmental challenges that require ongoing innovation and regulatory attention.
Automotive Industry
The automotive industry is undergoing a transformation, primarily driven by the integration of electronic cells. These cells are central to the development of electric vehicles (EVs) and hybrid models.
Key insights include:
- Reduction of Carbon Footprint: Electric vehicles powered by advanced battery technologies significantly minimize greenhouse gas emissions. With more manufacturers prioritizing transition to EVs, the demand for efficient electronic cells is paramount.
- Performance Enhancements: Modern electric vehicles utilize high-capacity lithium-ion or even solid-state batteries. These innovations provide longer driving ranges and quicker charging times, enhancing the user experience.
- Economic Impact: The shift towards electric vehicles also stimulates job creation in battery manufacturing and renewable energy sectors, contributing positively to economies.
Aerospace Applications
In aerospace, electronic cells play a critical role in powering various systems including satellites and spacecraft. Their functionality is vital for performance and safety.
Consider the following:
- Reliability and Safety: Aerospace applications demand high reliability. Electronic cells designed for this sector undergo rigorous testing to ensure they can withstand extreme conditions of altitude and temperature.
- Weight Considerations: The lightweight nature of advanced electronic cells is crucial in aerospace design. Reducing weight without compromising performance contributes to better fuel efficiency and increased payload capacity.
- Future Developments: Ongoing research aims to develop batteries with improved energy-to-weight ratios and faster charge capabilities, which will enhance both civilian and military aerospace operations.
Electronic cells not only power our devices but also drive progress across various industries, shaping a more connected and sustainable future.
End
The topic of electronic cells serves as a critical component in understanding both current and future technological advancements. This article has explored their structure and functionality, showcasing the multifaceted roles they play across various sectors. By synthesizing findings from distinct areas such as energy storage, biomedical innovation, and environmental monitoring, it becomes evident that electronic cells are not just passive components but active enablers of progress.
Summary of Findings
In summarizing the findings, it is clear that electronic cells exhibit unique characteristics that contribute significantly to modern applications. They have evolved from simple batteries to sophisticated systems that support a plethora of devices. Here are the key takeaways:
- Structural Importance: The architecture of electronic cells, including the roles of electrodes, electrolytes, and separators, is essential in dictating performance and efficiency.
- Diverse Applications: Their applications span consumer electronics, automotive, and aerospace industries. This diversity underscores their universality in today's technology-driven society.
- Challenges Identified: Degradation, cost, and environmental concerns remain significant barriers to the widespread adoption and development of new generations of electronic cells.
"Electronic cells are pivotal in driving forward technological innovations, but careful consideration of their limitations is equally important."
Implications for Future Research
Looking ahead, the implications for future research in electronic cells are paramount. Opportunities exist to further investigate and overcome current limitations, including:
- Innovative Materials: Research into new materials could lead to more efficient and longer-lasting electronic cells. Exploring nanomaterials and novel chemistries presents a promising avenue.
- Design Advancements: Continued evolution of design methodologies is essential. This can enhance the integration of electronic cells into devices, focusing on form factor and energy efficiency.
- Sustainability Focus: As environmental concerns grow, advancing the sustainability of electronic cells becomes critical. This includes recycling programs and the development of greener manufacturing processes.