In-Depth Analysis of DNA Fragmentation via Gel Electrophoresis
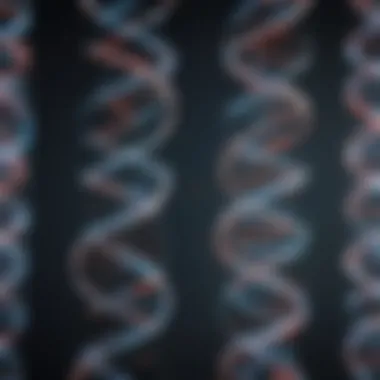
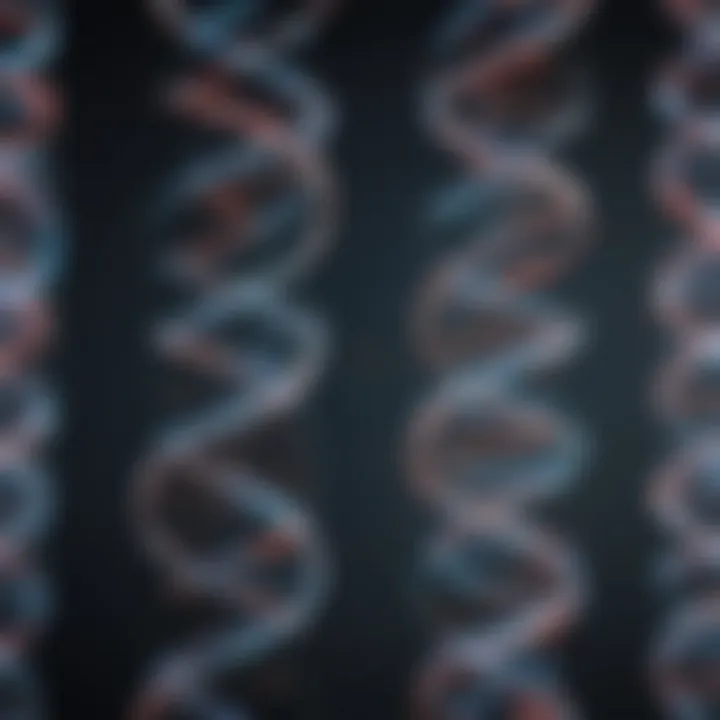
Intro
The process of DNA fragment analysis through gel electrophoresis stands as a cornerstone in the field of molecular biology. Understanding how this technique works opens up numerous avenues for research and application, from unraveling the complexities of genetic material to its indispensable role in biotechnology and forensic science. This guide aims to not only elucidate the methodologies behind this analytical technique but to also reveal its nuances that can often be overlooked.
In many ways, gel electrophoresis is a dance of molecules, with each fragment performing its own unique steps across the gel matrix. The primary principle at play is the movement of charged molecules through a viscous medium when an electric field is applied. This movement is significantly influenced by molecular size and charge, which ultimately dictates how well a fragment can navigate the gel.
By diving into the specifics of the procedure, the materials involved, and potential troubleshooting tips, this article provides a holistic view of DNA fragment analysis via gel electrophoresis. As you will soon discover, this technology is not just a technique; it's a powerful tool that has evolved dramatically, with promising innovations on the horizon.
Intro to Gel Electrophoresis
Gel electrophoresis has emerged as a vital tool in molecular biology, particularly in the analysis of DNA fragments. This technique allows scientists and researchers to separate and identify nucleic acids based on their size and charge. Understanding this process is crucial not only for academic study but also for various practical applications including forensic science, clinical diagnostics, and genetic research.
When discussing gel electrophoresis, several elements and benefits come into play. Primarily, it offers a clear visual representation of the DNA, enabling one to see how different fragments travel through a gel matrix. Additionally, its applications span a broad spectrum—from finding genetic markers linked to diseases to verifying the results of cloning procedures. The versatility of gel electrophoresis can't be overstated; it serves not just in basic research but also in advanced biotechnological applications.
As we delve deeper, the historical context of gel electrophoresis enriches our understanding of its development and implementation. This foundation sets the stage for grasping the principles that govern the technique, which are equally significant for appreciating its utility in both the lab and field scenarios.
Historical Context
The journey of gel electrophoresis began in the early 20th century, with its roots tracing back to protein analysis. The method was initially developed to separate proteins, and over time, its adaptability for nucleic acid analysis became apparent. By the mid-1970s, scientists had refined protocols specifically for DNA, marking a turning point in molecular biology.
The advent of automatic sequencers and the rapidly growing need to analyze genetic material fueled advancements in gel electrophoresis. This period saw innovations that enhanced both resolution and speed, leading to the emergence of high-throughput techniques in the late 20th century. As researchers sought to decode the human genome, gel electrophoresis became inseparable from DNA analysis, laying the groundwork for modern genetic research.
Principle of Gel Electrophoresis
At its core, gel electrophoresis is based on the movement of charged molecules in an electric field. DNA fragments are negatively charged due to their phosphate backbone. When an electric current is applied, these fragments migrate towards the anode, allowing for separation based on size. Shorter fragments travel faster through the gel compared to longer ones, resulting in a distinct banding pattern after the electrophoresis has concluded.
The gel matrix—often made of agarose or polyacrylamide—plays an essential role in this separation process. The choice of gel influences the resolution of the fragments, and the buffer solution used sustains a stable pH during the run. Key considerations like voltage, gel concentration, and duration can greatly impact the quality of results, which underlines the importance of careful optimization in experimental design.
"The more you understand the foundations of gel electrophoresis, the better equipped you are to tackle complex biological questions."
Thus, a solid grasp of these principles not only aids in interpreting results but also in optimizing procedures for varying experimental needs, making it a foundational technique in any molecular biology repertoire.
Understanding DNA and Its Fragments
Understanding DNA and its fragments is paramount in grasping the broader concept of DNA fragment analysis through gel electrophoresis. This section examines the structure of DNA, which provides the foundational knowledge required for the effective study and manipulation of genetic material. Moreover, it sheds light on fragmentation techniques, clarifying how these processes play significant roles in research and application.
DNA, or deoxyribonucleic acid, is the molecule that carries the genetic instructions for life. Its double helix structure, reminiscent of a twisted ladder, is essential to its function. The horizontal rungs of this ladder consist of nucleotide pairs, and the sequence of these pairs encodes the information necessary for the development and functioning of living organisms. Understanding how DNA is structured allows researchers to devise methods for fragmenting it, which is crucial for analysis.
By delving into the unique characteristics of DNA, one can appreciate the nuances in techniques used for preparing DNA samples for gel electrophoresis. Understanding DNA fragmentation provides several important insights:
- Identification of Genetic Material: Fragmentation is a fundamental step before running gel electrophoresis. It helps in isolating specific regions of interest within the DNA for further study.
- Applications in Forensics: In forensic science, the ability to analyze fragmented DNA can lead to breakthroughs in criminal investigations, paternity tests, and identification of remains.
- Advancement in Biotechnology: Fragmented DNA is often used in cloning, gene therapy, and various biotechnological applications, highlighting its importance in modern science.
Structure of DNA
The structure of DNA is often described as a double helix, made up of two strands of nucleotides twisted around each other. Each nucleotide comprises three components: a phosphate group, a sugar molecule (deoxyribose), and a nitrogenous base. There are four types of nitrogenous bases: adenine, thymine, cytosine, and guanine. The specific pairing of these bases—a to t and c to g—padlocks the sequence that encodes genetic information.
This intricate design is not just for show; it is vital for the functions DNA serves. The stability provided by the double helical structure allows it to maintain its integrity within a cellular environment, despite the numerous processes it undergoes. Furthermore, the sequence of bases along the DNA strand directs all genetic traits, influencing everything from physical appearance to susceptibility to certain diseases.
Understanding this structure is paramount for realizing how DNA can be fragmented in meaningful ways. As we progress into fragmentation techniques, the significance of maintaining the integrity and function of DNA becomes evident.
Fragmentation Techniques
Fragmentation is the process by which longer DNA molecules are broken down into smaller, manageable pieces. This process is essential for numerous applications, including cloning, genetic sequencing, and genotyping. Below are two primary techniques utilized in the fragmentation of DNA:
Mechanical Shearing
Mechanical shearing involves physically breaking DNA strands using physical force or devices. This approach can encapsulate various methods, including sonication or using a bead mill. One defining characteristic of mechanical shearing is its ability to produce a range of fragment sizes, which can be crucial depending on the subsequent applications. This variability makes mechanical shearing a popular choice among researchers.
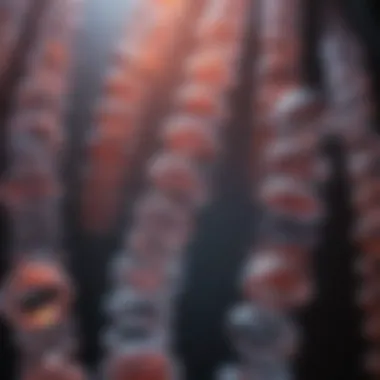
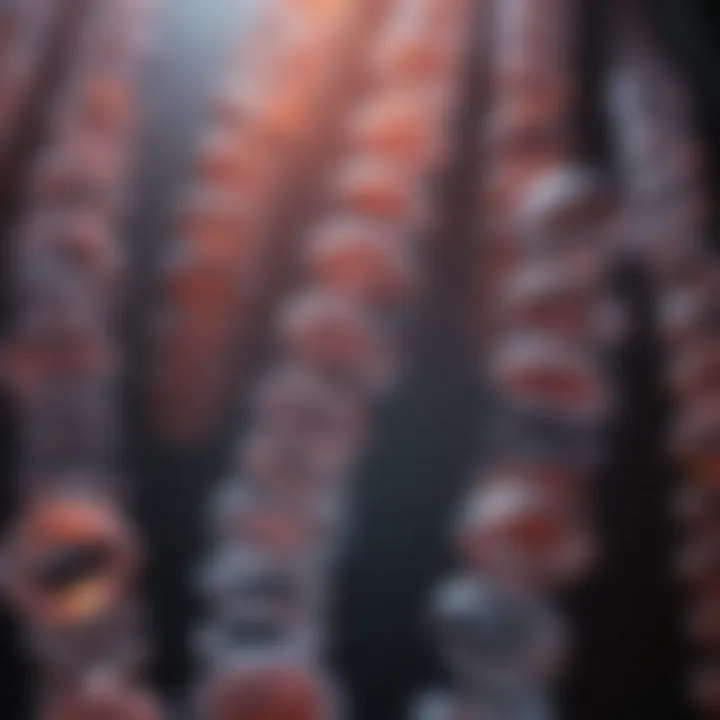
However, while versatile, mechanical shearing presents unique challenges. The fragments produced can be uneven, leading to a mix of smaller and larger DNA pieces that might not be suitable for all types of analysis. Moreover, excessive force can lead to excessive fragmentation, which could potentially harm the integrity of the required sequences.
- Pros:
- Cons:
- Encompasses a range of fragment sizes.
- Can be performed relatively quickly.
- Potential for uneven fragmentation.
- Risk of damaging sensitive DNA sequences.
Enzymatic Digestion
Enzymatic digestion employs specific enzymes, known as restriction endonucleases, to cleave DNA at predefined sequences. A key aspect of enzymatic digestion is its precision; enzymes can target particular sequences, allowing for highly specific fragment sizes that can suit particular research needs. This characteristic renders it a favorable choice for molecular cloning, where precise cuts are necessary to insert genes.
The unique feature of this technique is its ability to preserve the biological functionality of DNA fragments. This attribute is critical, particularly in genetic studies, where the requirement for intact sequences is vital.
However, enzymatic digestion is not without its drawbacks. The necessity of specific enzymes means that this method can be limited based on the DNA's sequences, and reagents can be expensive.
- Pros:
- Cons:
- Provides precise cuts at known sequences.
- Maintains biological functionality of fragments.
- Requires specific enzymes, limiting flexibility.
- Enzyme costs can add up.
Understanding these techniques highlights the diverse approaches for fragmenting DNA and the implications for downstream applications in gel electrophoresis. As we transition to the next sections, this foundational knowledge will inform our exploration of the mechanics involved in gel electrophoresis.
Mechanics of Gel Electrophoresis
The mechanics of gel electrophoresis serve as the foundation for understanding how DNA fragment analysis is carried out. It's not just a procedure; it's a meticulous orchestration of various physical principles that allow the separation of nucleic acids based on size and charge. This section highlights essential components and principles that play a crucial role in any gel electrophoresis experiment.
Components of Gel Electrophoresis
Agarose vs. Polyacrylamide
When we talk about the gel medium for electrophoresis, two materials often come into play: agarose and polyacrylamide. Each has its unique contributions to the analysis of DNA fragments.
Agarose is favored for its simplicity and suitability for separating larger DNA fragments, generally greater than 100 base pairs. Its beneficial characteristic lies in its ability to form a porous gel when it solidifies, allowing larger DNA molecules to migrate through with relative ease. Agarose gels can be cast at various concentrations, which directly affects the size of the pores. A higher concentration yields smaller pores, making it possible to separate smaller fragments more effectively. Meanwhile, polyacrylamide has its own set of characteristics that make it a popular choice, especially in applications requiring high-resolution separation of smaller DNA fragments, often below 100 base pairs. Its unique feature is that it can be prepared to achieve very fine control over pore size, resulting in enhanced resolution. However, the process for creating polyacrylamide gels is more complex and requires additional handling precautions due to its toxic chemical components.
Thus, the choice between agarose and polyacrylamide often boils down to the specific needs of the research.
- Agarose gel: Preferred for larger fragments.
- Polyacrylamide gel: Ideal for high-resolution applications.
Buffer Systems
Buffer systems are another critical element in the mechanics of gel electrophoresis. The buffer solution establishes the pH and ionic environment that enable DNA molecules to migrate effectively when an electric field is applied. Typically, Tris-acetate-EDTA (TAE) or Tris-borate-EDTA (TBE) buffers are used.
One key characteristic of these buffers is that they provide a stable pH during the electrophoresis process, which is essential for maintaining the integrity of DNA during migration. TAE buffer, for instance, is known for its ability to allow for better resolution of larger DNA fragments compared to TBE, which tends to support better overall efficiency and is particularly good for smaller fragments.
Unique to buffers is their role in maintaining the conductivity of the gel and overseeing how far the DNA migrates during the experiment. However, one must be cautious. Using the wrong buffer system can distort results. For instance, TAE tends to degrade during longer runs, causing less effective separations over time.
To summarize:
- TAE: Better for larger fragments, but degrades over time.
- TBE: More efficient, especially for shorter runs but could yield less clarity for large fragments.
Electric Field and DNA Movement
The electric field is what causes DNA to move through the gel, and understanding this principle is vital. DNA is inherently negatively charged due to its phosphate backbone, so when an electric field is applied, the fragments migrate toward the positive electrode.
- Movement of DNA is influenced by size: Smaller fragments travel farther than larger ones.
- The strength of the electric field can impact migration speed: A stronger field increases the rate, but there's always a balancing act as too much strength may create heat and lead to gel distortion.
Practical Applications
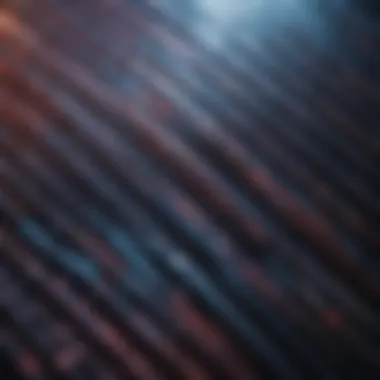
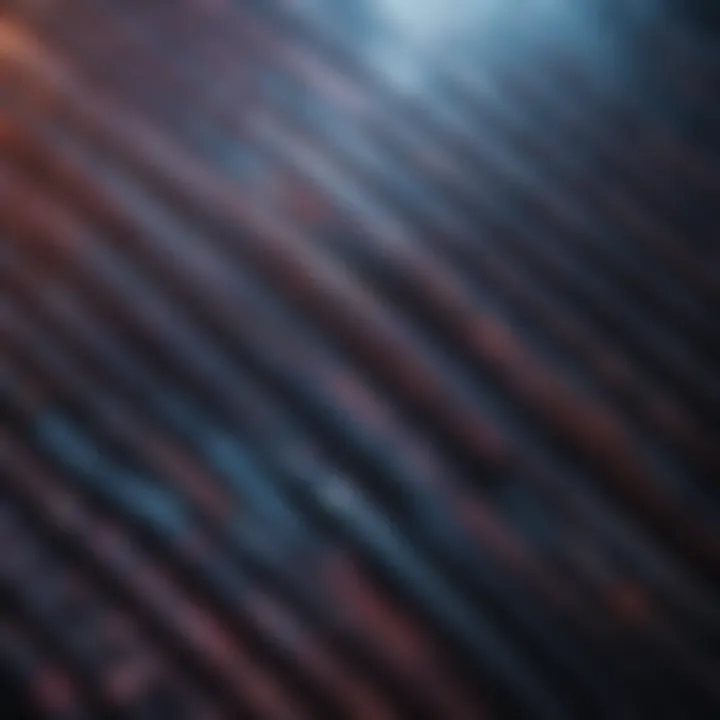
The field of gel electrophoresis boasts a multitude of practical applications that are immensely beneficial across various disciplines. It’s not just a lab exercise but a foundational technique in understanding molecular biology, genetics, and forensics. Practitioners in these fields realize that gel electrophoresis acts as a powerful tool for separating and analyzing DNA fragments. The importance of these practical applications extends beyond basic research; they penetrate into real-world issues, enhancing everything from criminal justice to medical treatments.
Forensic Analysis
In forensic science, gel electrophoresis takes center stage as a crucial methodology for analyzing genetic material. When a crime occurs, biological samples such as blood, hair, or skin cells are often left behind. Scientists utilize gel electrophoresis to identify individuals from these samples by comparing the DNA fragments to a known database.
The reliability of this technique can be life-changing. A DNA profile can often pinpoint a suspect or prove innocence, turning the tide in legal cases. Furthermore, it allows investigators to sift through complex mixtures of DNA, helping to unravel scenes where multiple contributors are involved. The speed and precision with which DNA can be analyzed has markedly improved the efficiency of legal investigations, making it a staple in modern criminal justice.
As noted by many professionals in the field, "The ability to obtain conclusive results from a small sample is one of the most empowering advancements in forensic science."
Genetic Research
Moving on to genetic research, the role of gel electrophoresis cannot be understated. In academic and industrial labs, scientists employ this technique to visualize and separate DNA fragments resulting from various experimental procedures. Whether it's the verification of PCR products or analyzing restriction enzyme digests, gel electrophoresis lends clarity to the complex work of genetics.
This is particularly valuable in understanding hereditary diseases, gene mapping, and evolutionary studies. By assessing the size and integrity of DNA fragments, researchers can glean insights into genetic variation within populations. The ability to quickly and accurately analyze hundreds of samples simultaneously has far-reaching implications in understanding the genetic basis of diseases, thereby informing treatments and preventive measures.
Biotechnology and Medicine
In the realm of biotechnology and medicine, gel electrophoresis opens the door to innovative solutions. The technology is pivotal in biopharmaceutical development, where it is used for quality control of nucleic acids and proteins. Researchers utilize it to ensure that the therapeutic products being developed are both pure and effective. This reliability is crucial, given the stakes involved in developing treatments for severe illnesses.
Moreover, gel electrophoresis aids in vaccine development and microbiological diagnostics by allowing researchers to separate and analyze antigens or pathogens efficiently. By understanding the molecular characteristics of diseased cells, the potential to create targeted therapies increases.
In addition, the continuous innovations in gel electrophoresis technology, such as miniaturization and multiplexing, enhance its applicability in personalized medicine. It reinforces the notion that the future of medicine can be tailored to individual genetic profiles. Thus, the integration of this analytical tool contributes not only to advancements in existing treatments but also paves the way for pioneering breakthroughs in healthcare.
"The intersection of technology and medicine is where the magic happens, and gel electrophoresis is a keystone in that structure."
In sum, the practical applications of gel electrophoresis shape our understanding and manipulation of DNA, influencing fields far and wide. By bridging gaps between research, forensic science, and biotechnology, it underscores the continuing evolution of scientific inquiry and its tangible benefits to society.
Interpreting Gel Electrophoresis Results
Understanding how to interpret the results of gel electrophoresis is vital in the processes involving DNA analysis. The results, typically represented as distinct bands on a gel, provide insight into the size, quantity, and purity of DNA fragments. For researchers and practitioners alike, being able to accurately read these results can mean the difference between successful experiments and those that fail to yield meaningful data.
Understanding Band Patterns
Band patterns observed in gel electrophoresis are a representation of DNA fragments that have been separated based on their size. As a general rule, smaller fragments travel faster and further through the gel matrix compared to larger ones. This difference creates specific bands that indicate the presence of various DNA pieces in a sample.
Successfully interpreting these band patterns requires one to consider numerous factors:
- Size marker: Utilizing a DNA ladder allows for the estimation of the size of unknown fragments. This ladder contains bands of known sizes, serving as a reference for comparison.
- Band intensity: The brightness of each band correlates to the quantity of DNA present. A faint band might indicate less DNA was loaded or that the fragment is degraded.
- Background noise: A clean background is essential for accurate interpretation, as any unwanted artifacts can obscure the true results.
Interpreting these patterns can reveal important information relevant to genetic studies, forensic investigations, or diagnostics in medicine. For instance, discrepancies in band size could suggest mutations in the DNA, while consistent patterns across samples might indicate similar genetic traits or environmental adaptations.
Quantification of DNA Fragments
Quantifying DNA fragments is another crucial aspect of interpreting gel electrophoresis results. This assessment helps researchers gauge how much DNA is present, aiding in multiple applications including cloning and sequencing.
Cellulose Acetate
Cellulose acetate is a popular medium used in electrophoresis due to its unique properties that allow for the effective separation of DNA fragments. One of the standout features of cellulose acetate is its porous nature; this allows DNA to pass through without excessive binding that could distort results. The main benefit of using this material is its resolution capability, making it particularly useful for smaller fragments which can be challenging to detect with other mediums. However, cellulose acetate can present challenges such as lower mechanical strength compared to agarose or polyacrylamide gels, potentially leading to tearing during the analysis process.
Digital Imaging
Digital imaging has become a transformative tool in the realm of gel electrophoresis, facilitating advanced quantification of DNA fragments. The high sensitivity and specificity of digital imaging systems allow for precise capture of band patterns and intensity. A key characteristic of this method is the ability to permanently document results, making re-evaluation and comparison straightforward. Furthermore, digital imaging systems often come equipped with software for automatic quantification, which minimizes human error. Nevertheless, one should consider that reliance on digital imaging may necessitate calibration and controls to ensure accuracy, especially when different imaging systems are utilized—varying light sources can sometimes lead to discrepancies in results.
"Interpreting gel electrophoresis results accurately is a blend of art and science, combining meticulous observation with analytical skills."
In summary, the interpretation of gel electrophoresis results, focusing on band patterns and quantification, requires attention to detail and an understanding of the underlying techniques involved. By mastering these aspects, individuals in genetics, forensic science, and various fields of research can glean the essential information required to advance their studies.
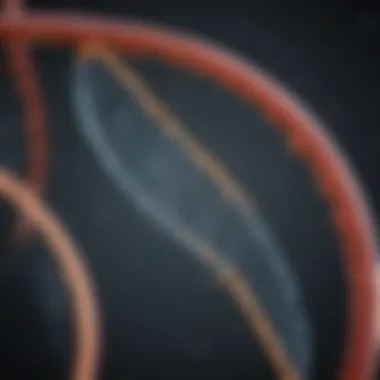
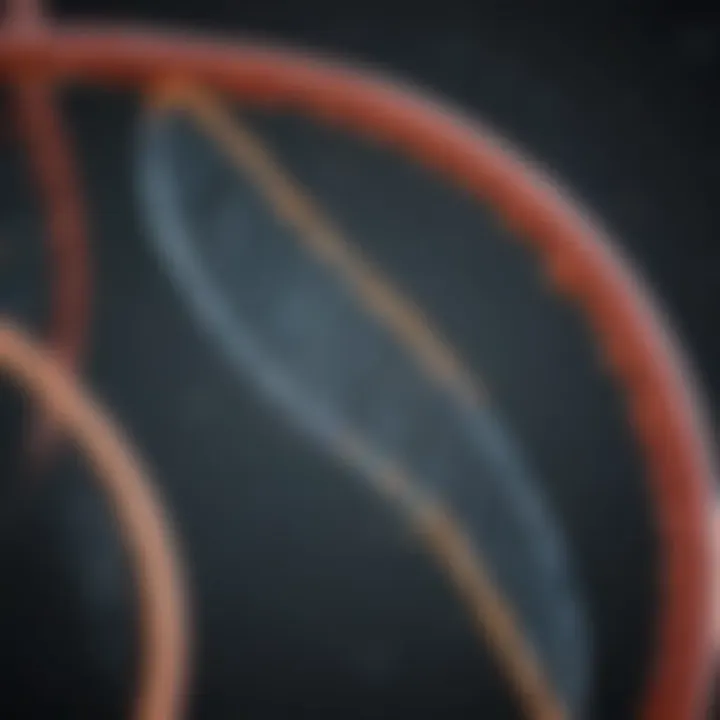
Troubleshooting Common Issues
In the field of gel electrophoresis, troubleshooting common issues is a crucial skill for any researcher or technician. Even the most experienced practitioners can encounter challenges that may impede the accuracy and reproducibility of results. Understanding how to identify and resolve these issues can greatly impact the success of an experiment. It saves both time and resources, making it a vital topic in any detailed examination of DNA fragment analysis. Various artifacts can arise from a number of sources, from the quality of reagents to the settings used during electrophoresis. Moreover, knowing how to optimize conditions effectively can enhance the overall quality of the analysis.
Common Artifacts
Artifacts in gel electrophoresis often appear as misleading bands or smears and can be the bane of any scientist's existence. These anomalies can stem from multiple factors, like sample preparation errors, gel quality issues, or even electric field inconsistencies. For instance:
- Improperly prepared gels can lead to uneven migration patterns, causing bands to appear distorted or spread out.
- Contamination from either reagents or sample handling may create additional, unwanted bands on the gel. This could mislead one into thinking there are more DNA fragments present than what actually exists.
- Degradation of samples can occur if DNA is left exposed to high temperatures or nucleases, resulting in smears instead of distinct bands.
These conditions necessitate close attention during the entire electrophoresis process, as they can drastically alter the interpretation of results.
"Anomalies in gel analysis can shift conclusions, making for a challenging hurdle in research contexts."
Optimization Techniques
Once the sources of artifacts are pinpointed, implementing optimization techniques is the next step towards refining results. Adjusting parameters for gel electrophoresis can significantly improve clarity and separation of DNA fragments. Some useful techniques include:
- Gel Concentration: Altering the percentage of agarose or polyacrylamide can greatly affect resolution. Higher concentrations are often better for separating smaller DNA fragments, while lower concentrations work well for larger sequences.
- Buffer Choice: Using an appropriate electrophoresis buffer, such as TAE or TBE, is essential. Each buffer has unique properties that may influence DNA migration.
- Voltage Settings: Controlling the voltage applied during electrophoresis can help in managing heat generation and ensuring uniform migration. Typically, lower voltages yield better resolution, albeit at the cost of longer run times.
- Sample Volume and Loading: Ensuring the right amount of sample is loaded can minimize smearing. Overloading wells is a common mistake that can obscure band visibility.
By integrating these optimization techniques, researchers can mitigate the issues posed by artifacts, leading to more reliable and informative results in the analysis of DNA fragments.
Innovations in Gel Electrophoresis
The field of gel electrophoresis is not stagnant; it is constantly evolving to meet the demands of modern science. Innovations in this area are essential not only for improving the accuracy and efficiency of DNA fragment analysis but also for enabling researchers to tackle increasingly complex biological questions. As new materials and technologies are developed, they open the door to enhanced capabilities in genomic studies, forensics, and various branches of biotechnology.
Advancements in Materials
When we talk about advancements in materials, it goes beyond just the gels themselves. Traditionally, agarose and polyacrylamide have been the go-to options for creating gel matrices. However, recent developments have introduced hybrid gels that blend elements of both materials, enhancing resolution while reducing the time required for analysis. This cuts down on experiment time without sacrificing quality.
Another noteworthy advancement involves the use of nanomaterials. Nanoparticles, when incorporated into the gel matrix, provide a unique property: they can change the way electric currents interact with the DNA fragments. The result is clearer banding patterns, which offers a cleaner analysis and reduces the potential for artifacts. Moreover, these materials can promote better separation of closely-sized DNA fragments, which is particularly important in applications such as forensic analysis where precision is vital.
Furthermore, chemical modifiers are being more frequently employed to optimize gel performance. The introduction of these additives can affect the pore size of gels, giving researchers the ability to fine-tune conditions to better suit their specific analysis needs. These innovations transform the way we approach gel electrophoresis, making it more versatile and accessible than ever.
Incorporation of Automation
Automation in gel electrophoresis is yet another frontier being explored. The manual setup of electrophoresis can be labor-intensive and prone to human error, affecting the outcome of experiments. By integrating automated systems, researchers can enjoy consistency and reliability that were hard to achieve in manual setups.
Automated systems can handle the preparation of samples, setup of the gels, and even the electrophoresis process itself. For instance, systems like the Bio-Rad's Gel Doc XR+ come equipped with programmable features and accessories. These innovations allow for a greater throughput of samples and minimize the wait time for results.
Moreover, image acquisition and analysis have also been automated. With advanced imaging software, the interpretation of band patterns can be done swiftly and accurately. This is significant for high-volume laboratories and research facilities, where time management is key.
"Automation in gel electrophoresis not only streamlines the workflow but also enhances reproducibility, allowing scientists to devote more time to actual research rather than technical procedures."
With the incorporation of automation, the barriers to entry for high-quality DNA analysis are lowered, making sophisticated techniques available to a broader range of laboratories. This is a game-changer for educational institutions as well, where resources may not be as plentiful.
In summary, the innovations taking place in gel electrophoresis are pivotal. New materials are revolutionizing the way we conduct analyses, while automation enhances efficiency and accuracy. Together, these advancements are ensuring that scientists can keep pace with the demands of modern research and continue to yield meaningful results.
Epilogue
The conclusion of this article brings to light the significant role that gel electrophoresis plays in the analysis of DNA fragments. This technique has become a cornerstone in a variety of scientific fields, showcasing its utility from forensic investigations to medical diagnostics and beyond. Understanding the nuances of gel electrophoresis not only enhances our comprehension of genetic material but also bolsters our ability to develop innovative solutions to complex problems.
Key Elements of the Ending:
- Integration of Knowledge: The article has provided a detailed exploration of the principles and mechanics underlying gel electrophoresis. By synthesizing this information, readers can appreciate how these components come together to enable effective DNA analysis.
- Benefits Highlighted: As we’ve traced the practical applications of gel electrophoresis—from supporting genetic research to enabling forensic analyses—its importance in revealing the intricate details of DNA fragmentation and migration patterns cannot be overstated.
- Considerations and Future Outlook: The field is evolving rapidly. Future directions in gel electrophoresis, including innovative materials and automation, promise to enhance accuracy and efficiency. This trajectory suggests that gel electrophoresis will continue to expand its influence.
Thus, the conclusion serves as a catalyst for encouraging further inquiry into this impactful method. By acknowledging both its current applications and future possibilities, we demonstrate that the journey of understanding DNA and its analysis is far from over.
"In the realm of genetics, every analysis performed is a step closer to deciphering the intricate code of life."
Future Directions
In considering the future of DNA fragment analysis through gel electrophoresis, several fronts emerge that hold the potential for significant advancement:
- Enhanced Efficiency: Continued development in automation technologies could greatly reduce the time required for analysis, making the process more accessible to various fields.
- Improved Accuracy: Innovations in materials used for gel matrices may lead to more precise separations of DNA fragments, aiding in the resolution of complex genetic information.
- Integration with Other Technologies: The potential for collaboration with sequencing technologies, such as next-generation sequencing (NGS), could offer comprehensive insights, combining the strengths of multiple methodologies.
- Artificial Intelligence and Machine Learning: Integrating AI with gel electrophoresis could lead to smarter data analysis, allowing for faster identification of patterns and anomalies in DNA samples.