Conversion of Biomass to Energy: An In-Depth Overview
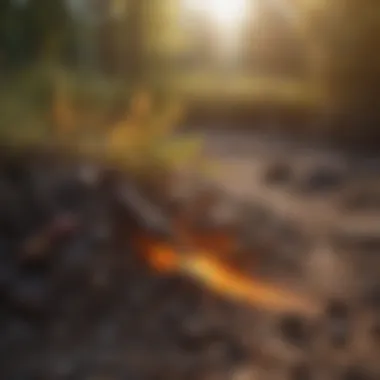
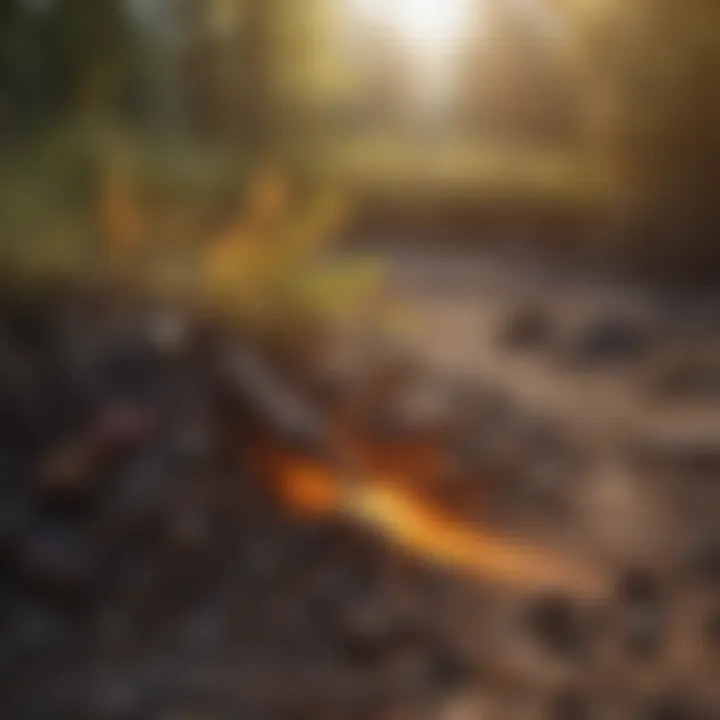
Intro
Biomass conversion to energy is a significant aspect of the larger renewable energy dialogue. It encompasses various methods of transforming organic materials into usable energy forms, such as electricity, heat, or biofuels. The topic of biomass energy appeals to many due to its dual capacity to mitigate waste and provide sustainable energy solutions. The practicality of using biomass over fossil fuels aligns with various environmental and economic imperatives. However, understanding the nuances of this conversion process is crucial for its successful application in contemporary energy systems.
In recent years, advancements in biomass technologies have sharpened the focus on this arena. Researchers and policymakers alike consider it a viable strategy to reduce greenhouse gas emissions and fossil fuel dependence. With the growing need for cleaner energy sources, biomass energy occupies a prominent space in the renewable energy landscape. This prompts an examination of the advantages, challenges, and implications tied to the conversion processes involved.
Methodology
Overview of research methods used
In exploring the conversion of biomass to energy, a diverse range of research methodologies is employed. These methods include both qualitative and quantitative approaches. Articles, case studies, and reviews play a vital role in gathering existing knowledge on biomass energy. Surveys and interviews with industry professionals provide firsthand insights into operational practices and technological advancement.
Data collection techniques
Data collection for this field is varied and may include:
- Literature reviews: This method involves reviewing existing scientific literature on biomass conversion, leading to a comprehensive understanding of prior research findings.
- Experimental trials: Actual trials in laboratory or industrial settings facilitate practical knowledge of the conversion techniques.
- Statistical analysis: The use of statistical tools helps evaluate the efficiency of different conversion methods.
- Field studies: Observational methods in natural settings provide insights into the performance and challenges faced in real-world applications.
By integrating these various techniques, a robust narrative surrounding biomass conversion emerges, presenting both its potential and challenges clearly.
Future Directions
Upcoming trends in research
Looking ahead, several trends are noteworthy in the biomass energy sector. One emerging direction involves the exploration of new feedstock sources. Researchers are aiming to identify more sustainable and efficient raw materials that can be converted into energy. Additionally, developments in biogas and biofuel technologies are gaining traction.
Areas requiring further investigation
While progress is visible, several areas still require considerable attention:
- Efficiency improvements: Research into enhancing the energy conversion efficiency must continue.
- Post-conversion impacts: Understanding the environmental impacts of residuals and byproducts generated during biomass conversion can lead to more effective management strategies.
- Policy and economic frameworks: Establishing supportive policies will be crucial to facilitating biomass energy adoption.
The future of biomass energy conversion strongly ties to innovative research and realistic implementation strategies.
Through ongoing investigation and adaptation, biomass energy can significantly contribute to a sustainable energy future.
Prolusion to Biomass Energy
Biomass energy has gained substantial attention in recent years as a vital component in the shift towards sustainable energy sources. This section outlines why understanding biomass energy is crucial for students, researchers, educators, and professionals interested in renewable energy solutions. Biomass refers to organic materials that can be converted into energy, contributing positively to both energy security and environmental sustainability.
The significance of biomass energy lies in its versatility. Unlike some forms of renewable energy, such as solar or wind, biomass can be utilized for various applications. It is applicable across different scales, from small-scale power generation to large industrial processes. This adaptability makes it an attractive choice for diverse energy needs.
Additionally, biomass can play a pivotal role in waste management. Converting agricultural, forestry, and municipal waste into energy not only reduces landfill use but also creates an opportunity for economic growth in rural communities. By harnessing local resources, biomass energy can lead to job creation and a more resilient energy system.
Incorporating biomass energy into the broader energy landscape aids in reducing reliance on fossil fuels, thus directly mitigating greenhouse gas emissions. This transformation is necessary to tackle climate change and promote a cleaner environment. As global energy demands grow, understanding biomass energyβs potential is essential for developing effective strategies for a sustainable future.
Definition of Biomass
Biomass is defined as biological material that comes from living organisms, primarily plants and animals. This term encompasses a wide array of materials, including wood, agricultural crops, and animal waste. Biomass can be classified as either primary or secondary. Primary biomass comprises raw materials, while secondary biomass refers to products derived from processing these raw materials.
The energy contained in biomass is stored in chemical bonds, which can be released through various conversion processes. This stored energy can then be utilized for generating electricity, producing heat, or even creating biofuels.
Historical Significance of Biomass Use
The use of biomass for energy dates back thousands of years. Early societies relied heavily on wood as their primary energy source for cooking and heating. As civilizations advanced, they began to exploit other forms of biomass, including crop residues and animal waste.
Historically, biomass was the dominant source of energy until the advent of fossil fuels during the Industrial Revolution. This shift offered increased energy density and efficiency, overshadowing traditional biomass use. Nevertheless, biomass remains significant today. It has evolved in response to modern energy challenges.
The resurgence of interest in biomass is closely linked to contemporary concerns about climate change and energy sustainability. Scholars and practitioners are now exploring innovative ways to revitalize biomass energy, integrating it into renewable energy systems that are both effective and sustainable.
Understanding biomassβs historical context allows us to appreciate its potential in todayβs energy landscape and informs our strategies for its effective utilization.
Types of Biomass
Understanding the different types of biomass is crucial for exploring the various pathways of converting biomass to energy. Each biomass category plays a distinct role in energy generation and offers unique benefits and challenges. The significance of Types of Biomass lies in its diversity, which provides multiple avenues for energy production while reinforcing sustainability goals.
Different types of biomass include organic waste materials, dedicated energy crops, and agricultural residues. Each type can be processed through various conversion technologies, impacting the efficiency and cost-effectiveness of energy production. This section delves into these categories, highlighting their implications towards energy sustainability and economic viability.
Organic Waste Materials
Organic waste materials represent one of the most abundant biomass categories. They comprise leftover food, yard waste, and other biodegradable materials that are often discarded. Utilizing organic waste has key advantages. First, it helps in reducing landfill waste. Second, it generates energy that can replace fossil fuels.
Processing organic waste through methods such as anaerobic digestion produces biogas, which can be used for heating or electricity generation. In turn, this contributes to a circular economy by enhancing resource efficiency. Moreover, organic waste materials significantly lower greenhouse gas emissions, offering a cleaner alternative to traditional waste disposal methods.
Dedicated Energy Crops
Dedicated energy crops are specifically grown for energy production. Examples include switchgrass, miscanthus, and giant reed. These crops are chosen for their high yield and fast growth rates, making them particularly appealing for biomass energy.
The cultivation of dedicated energy crops provides several benefits. They can be grown on marginal lands, thus not competing with food production. Additionally, these crops can improve soil quality and capture carbon during their growth phases. Despite these advantages, challenges like water usage and input costs must be considered when integrating them into an energy strategy. Bioenergy sourced from dedicated energy crops has the potential to play a significant role in achieving energy independence.
Agricultural Residues
Agricultural residues, which include leftover stalks, husks, and shells, are another important biomass type. These materials are typically seen as waste after harvest, yet they hold value for energy conversion. By harnessing agricultural residues, energy producers can make use of resources that would otherwise go unused.
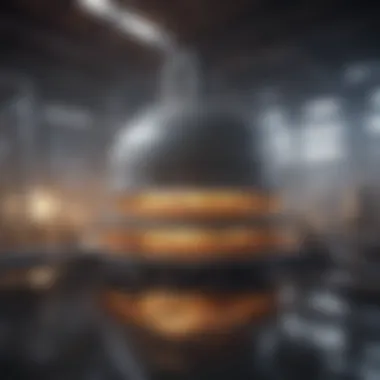
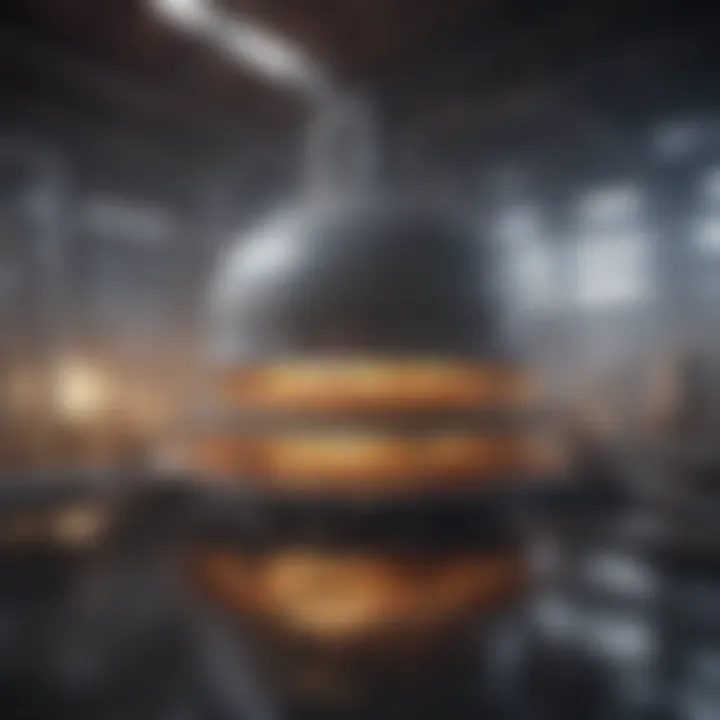
The benefits of using agricultural residues are noteworthy. It helps in achieving better waste management and enhances the sustainability of agricultural practices. Moreover, processing these residues through combustion or gasification can efficiently convert them into heat and electricity. Utilizing agricultural residues supports rural economies by creating jobs and boosting local energy production, which is particularly vital in regions reliant on agriculture.
Biomass energy, derived from diverse sources, presents an essential strategy in the pursuit of sustainability and energy resilience.
Biomass Conversion Technologies
Biomass conversion technologies are vital to understand in the context of transforming biomass into usable energy. These technologies take various forms, each with its own specific benefits and considerations. The efficiency and sustainability of energy production from biomass depend significantly on the choice of conversion technology. They play a crucial role in minimizing waste while maximizing energy output.
Thermochemical Conversion
Thermochemical conversion refers to processes that use heat to convert biomass into energy. This method is essential because it offers a way to extract energy through various reactions, which are effective for different types of feedstock.
Pyrolysis
Pyrolysis is a thermal decomposition of materials at elevated temperatures in the absence of oxygen. This process is noteworthy for its ability to produce biochar, bio-oil, and syngas. The key characteristic of pyrolysis is its efficiency in converting a wide variety of biomass materials into valuable energy products. It is a popular choice in the biomass energy landscape.
The unique feature of pyrolysis lies in its capacity to produce biochar, a solid material that has applications in soil enhancement. This adds an advantageous layer since it helps in carbon sequestration. However, the process can require considerable energy input, which can be a disadvantage.
Gasification
Gasification is another significant thermochemical process. In this conversion method, biomass is converted into syngas, which primarily consists of carbon monoxide, hydrogen, and some carbon dioxide. The primary appeal of gasification is its versatility as it can utilize a range of biomass materials, including agricultural residues and municipal waste.
Gasificationβs unique feature is its ability to produce a clean synthesis gas that can be used for electricity generation or as a substitute for natural gas. However, it can face challenges such as producing tar and other by-products that may require additional treatment.
Combustion
Combustion is perhaps the most straightforward and widely adopted method for energy generation from biomass. It involves burning biomass to produce heat, which can then be used to generate electricity. The key characteristic of combustion lies in its simplicity and the relatively low cost of the technology compared to others.
The unique feature of combustion is its immediacy in producing energy. However, the process must be managed carefully as it can emit pollutants if not properly controlled. Moreover, its reliance on unsustainable biomass sources may limit long-term sustainability.
Biochemical Conversion
Biochemical conversion harnesses biological processes to transform biomass into energy. This section covers conversion methods that use microorganisms to break down organic matter, producing energy-rich products.
Anaerobic Digestion
Anaerobic digestion is an important method where microorganisms break down organic matter without oxygen. This process produces biogas, which is primarily methane, and is a renewable energy source. Anaerobic digestion's key characteristic is its efficiency in treating wet organic waste, making it suitable for food waste and agricultural residues.
The unique advantage of anaerobic digestion is its capacity to reduce methane emissions that would otherwise occur from landfills. However, it requires specific microbial conditions and careful management of input materials, which can be a downside.
Fermentation
Fermentation is a biochemical process in which sugars are converted into alcohol or organic acids by yeast or bacteria. This method is commonly employed to produce ethanol from sugar or starch-based biomass. The key aspect of fermentation is its ability to produce high-energy biofuels effectively, making it a popular choice for liquid fuel production.
A unique feature of fermentation is its potential to utilize various feedstocks, from sugarcane to agricultural wastes. However, it can be sensitive to changes in microbial activity and requires precise control of environmental conditions.
Enzymatic Hydrolysis
Enzymatic hydrolysis involves breaking down complex biomolecules into simpler sugars using enzymes. This method is important for advanced biofuel production as it can increase the efficiency of sugars conversion into ethanol or other biofuels. The key characteristic of enzymatic hydrolysis is its ability to process lignocellulosic biomass, which includes many types of agricultural residues.
Enzymatic hydrolysis's uniqueness is in its specificity and efficiency in converting recalcitrant materials into fermentable sugars. However, the cost of enzymes can be quite high, which may present a limitation in larger-scale applications.
Physical and Chemical Transformation
Physical and chemical transformation processes convert biomass into energy-rich products through non-biological methods.
Transesterification
Transesterification is the process used to produce biodiesel from triglycerides. This is a crucial method for producing renewable diesel fuel. The key characteristic of transesterification is its ability to transform vegetable oils or animal fats into biodiesel, making it an important technology for alternative fuels.
A unique feature of transesterification is its efficiency, allowing a high yield of biodiesel. However, the feedstock's availability and cost can affect the overall economic viability of this process.
Hydrothermal Liquefaction
Hydrothermal liquefaction involves converting biomass into crude oil-like products using high temperature and pressure in the presence of water. This process is beneficial for soggy feedstocks like algae or wet organic waste. The key characteristic is its ability to process feedstocks with high moisture content, which is a common barrier for other conversion methods.
The unique aspect of hydrothermal liquefaction is its potential to create renewable crude oil. However, the technical complexity and energy requirement of this process pose challenges to large-scale application.
Efficiency of Biomass Conversion
Understanding the efficiency of biomass conversion is essential for evaluating the viability of this energy source. Efficiency refers to how well biomass is transformed into usable energy. In a landscape where energy demands continue to rise, assessing biomass conversion efficiency can reveal its potential in meeting renewable energy goals. Various factors contribute to the overall performance of biomass conversion processes, including technology choice, feedstock quality, and operational conditions.
Higher energy efficiency in biomass conversion means less waste and better utilization of resources. This is critical given the increasing focus on sustainable practices to mitigate climate change impacts. Efficient conversion methods can enhance fuel outputs, which translates into more energy generation without amplifying the negative environmental footprint.
Moreover, efficiency affects economic viability. The costs associated with biomass energy production are directly tied to energy yields and conversion rates. When more energy is extracted from the same quantity of biomass, it can lead to lower costs per unit of energy generated. This is crucial for stakeholders and investors in the biomass sector, offering insights into cost-reduction strategies and profit potential.
Energy Yields
Energy yield is a measure of how much energy can be derived from a specific quantity of biomass. It is determined by both the type of biomass used and the conversion technology applied. Some biomass sources, such as dedicated energy crops, typically offer higher energy yields compared to organic waste materials. Understanding these yields helps in choosing appropriate biomass sources for energy production.
The energy yield can vary significantly across different conversion methods. For instance, conversion technologies like gasification and anaerobic digestion may produce different quantities of energy from the same biomass input. Key factors influencing energy yield include:
- Feedstock Type: Some materials inherently contain more energy.
- Moisture Content: High moisture levels can reduce the effective yield.
- Conversion Technology: Each method has efficiency rates that affect output.
Maximizing energy yield remains a focus of ongoing research in the biomass field. Increasing energy recovery could make biomass a more attractive option when compared to other renewable sources.
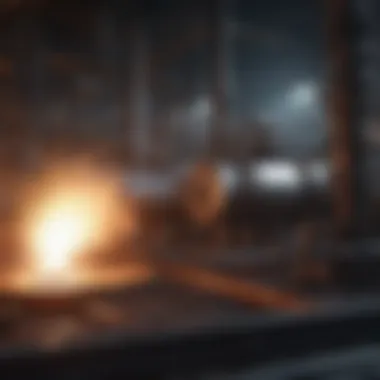
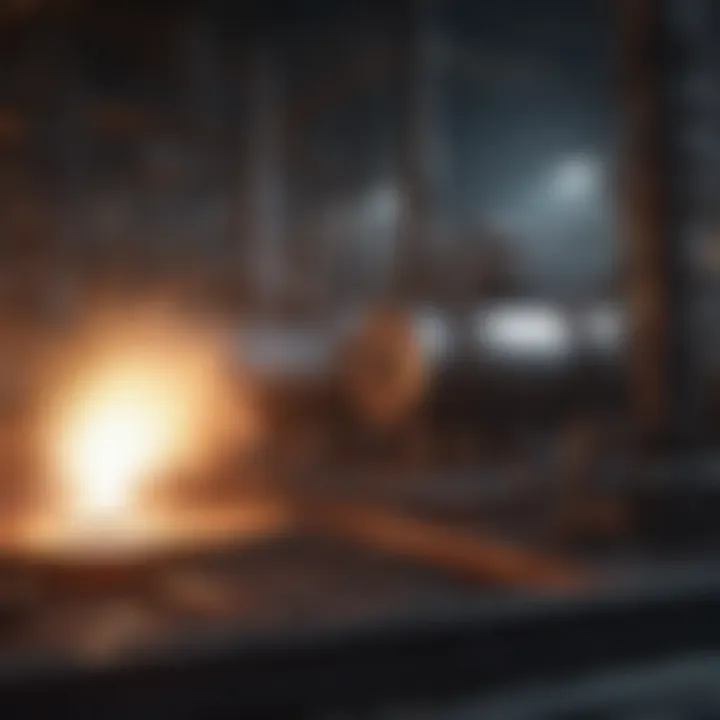
Comparison with Other Renewable Sources
The comparison of biomass conversion efficiency with other renewable energy sources is a significant aspect of evaluating its role in the energy mix. Biomass often competes with solar, wind, and hydroelectric power, each with unique characteristics.
- Solar Energy: Generally harvested through photovoltaic panels. The efficiency of solar panels can reach 20% or more, converting sunlight into electricity.
- Wind Energy: Wind turbines can achieve efficiencies of around 35-45%, but they depend heavily on location and wind resources.
- Hydropower: Has some of the highest efficiencies, often above 90%, but is limited by geographical and ecological constraints.
Biomass, with its energy yields, often ranges from 15-25% efficiency depending on the technology and feedstock. It acts as a complementary energy source when combined with others like solar and wind, especially in providing baseload power.^
A clear advantage of biomass is its ability to utilize waste materials, thus reducing landfill impacts while producing energy. However, its effectiveness can be overshadowed by more established renewable sources when evaluated strictly on efficiency metrics. Yet, advancements in biomass conversion technologies might shift this balance as efficiency improvements are realized.
The sustainability and economic benefits of biomass energy can be considerable, especially when well-managed practices are in place, ensuring minimal environmental impact while maximizing energy recovery.
Environmental Impacts
The exploration of biomass energy conversion brings forth vital considerations regarding its environmental impacts. Understanding these impacts is key to evaluating biomass as a sustainable energy source. It is essential to balance energy production needs with ecological preservation. The environmental footprint of biomass energy encompasses various elements including greenhouse gas emissions, biodiversity effects, and land usage.
Carbon Footprint Analysis
Carbon emissions are a primary concern when evaluating energy sources. Biomass is often regarded as a renewable energy source because the carbon dioxide released during its combustion can be offset by the carbon dioxide absorbed by plants during their growth. Nevertheless, the carbon footprint of biomass conversion varies. Factors such as transportation, processing, and type of biomass used play crucial roles in this analysis.
- Transportation Emissions: Moving biomass from its source to conversion facilities can generate significant emissions. Efficient logistics can mitigate this.
- Processing Impact: Different conversion technologies impact the overall carbon balance. For instance, anaerobic digestion may produce less carbon than traditional combustion methods.
- Sustainable Management: The source of biomass is also important. Sustainably managed forests and agricultural residues tend to have a lower net carbon impact compared to unsustainable practices.
According to research, careful lifecycle assessments are crucial in providing a more accurate calculation of biomassβs net carbon footprint. Ultimately, the aim is to achieve carbon neutrality over time, ensuring that biomass energy can contribute positively to climate goals.
Biodiversity Considerations
The relationship between biomass energy and biodiversity is intricate. While biomass can enhance environmental sustainability, it can also pose threats to ecosystems if not managed appropriately. Key considerations include:
- Land Use Changes: Converting natural landscapes to dedicated energy crops can lead to habitat loss for various species. This emphasizes the need for strategic land use planning.
- Invasive Species: Introducing non-native biomass crops can disrupt local ecosystems. Monitoring plant species is essential to prevent invasive species from taking hold.
- Soil Health: Sustainable practices in biomass cultivation can enhance soil quality. Conversely, unsustainable farming practices may degrade soil health, affecting both productivity and local biodiversity.
"Sustainability in biomass energy production necessitates a holistic approach to ensure that it does not compromise the ecosystems that sustain us."
The significance of biodiversity in biomass energy cannot be understated. While biomass offers many benefits, the need for careful planning and management is paramount to preserve ecological integrity and ensure that it contributes to a genuinely sustainable energy future.
Economic Aspects of Biomass Energy
The economic aspects of biomass energy are integral to understanding its viability and potential in the current energy landscape. As the world shifts towards renewable energy sources, evaluating the costs, benefits, and overall market dynamics surrounding biomass is essential. Economic considerations can influence policy decisions, investment opportunities, and the sustainability of biomass energy practices.
A comprehensive study of biomass energy economics addresses several factors:
- Cost of Technology and Infrastructure: Initial setup costs for biomass facilities can be significant. This includes expenses for equipment, energy crop cultivation, and processing facilities. However, the long-term savings and potential returns on investment can eventually offset these initial costs.
- Job Creation and Local Economies: Biomass energy projects can stimulate local economies by creating jobs in farming, energy production, and transportation. This can lead to improved community resilience and economic diversification in rural areas.
- Market Viability: Understanding the supply-demand dynamics for biomass energy is crucial. Factors like regulatory support, energy prices, and public acceptance play a role in determining its market position. A stable market can attract more investments.
- Environmental Economics: Although primarily focused on financial aspects, incorporating environmental costs can enhance the economic narrative. For example, biomass energy can help mitigate greenhouse gas emissions, thereby reducing future economic liabilities.
"Economic viability is key for biomass energy to compete against traditional fossil fuels and other renewable sources."
Cost Analysis of Biomass Conversion
Cost analysis of biomass conversion examines the financial implications of transforming biomass into usable energy. This process involves various expenses related to collection, processing, and transportation.
Key Considerations:
- Feedstock Costs: The type and source of biomass feedstock directly impact overall costs. Local availability of organic waste or dedicated energy crops influences procurement expenses.
- Processing Methods: Different conversion technologies, such as anaerobic digestion or gasification, come with varying capital and operational costs. Selecting the most suitable technology based on economic feasibility is essential for successful project implementation.
- Subsidies and Incentives: Government programs that subsidize biomass projects can lower costs significantly. Understanding available incentives is vital for accurate cost projections.
Overall, assessing costs related to biomass conversion helps stakeholders determine whether projects can achieve financial sustainability and energy efficiency.
Market Trends and Forecasts
Market trends and forecasts in biomass energy offer insights into its growth potential and challenges. As interest in renewable energy grows, understanding market dynamics becomes increasingly important.
Important Trends:
- Increased Investments: There is a noticeable increase in investments in biomass technology and infrastructure. This reflects a shift in both public and private sectors toward renewable energy solutions.
- Innovation in Technologies: Ongoing research in biomass conversion technologies like efficient biofuels is opening new avenues for market expansion.
- Global Market Growth: The biomass energy sector is projected to see significant growth internationally. Regions focusing on energy independence and sustainability continue to embrace biomass as a viable energy source.
Predictions for the upcoming years suggest that as technology improves and public policy becomes more favorable, biomass energy will play a larger role in achieving global energy goals.
Biomass and Energy Policy
The relationship between biomass and energy policy is crucial for the sustainable development of renewable energy. Effective energy policies can facilitate biomass's role in the energy sector, guiding investment and fostering innovation. Policymakers must consider various elements, including regulatory frameworks, economic incentives, and public perception, to maximize the benefits of biomass energy.
Biomass offers a range of advantages. It not only serves as a renewable source of energy but also contributes to waste reduction and greenhouse gas mitigation. Enhanced energy policies can drive the adoption of biomass in diverse contexts, from small-scale community projects to large industrial facilities. By aligning biomass energy with national sustainability goals, countries can achieve energy security and environmental protection simultaneously.
Regulations Impacting Biomass Energy
Regulations play an essential role in shaping the biomass energy landscape. These laws can establish standards for production, ensure sustainability, and protect public interests. Governments worldwide have implemented various regulations that directly impact the biomass sector. Some key regulations include:
- Sustainability Criteria: Many jurisdictions require biomass producers to comply with sustainability criteria. This may include resource management practices, adherence to local ecological regulations, and considerations of carbon emissions.
- Quality Standards: Regulations often outline the technical standards for biomass fuels. This helps ensure that feedstock quality meets industry needs while promoting safety and efficiency in conversion processes.
- Environmental Assessments: Certain policies mandate environmental impact assessments for large-scale biomass projects. Such assessments evaluate potential effects on ecosystems and local communities, aiming to prevent negative outcomes.
Overall, these regulations aim to create a balanced framework that encourages biomass development while safeguarding ecological and social interests.
Incentives for Biomass Production
Incentives are a powerful tool to promote biomass production. They can take various forms, such as financial subsidies, tax credits, and grants for research and development. These incentives help offset initial investment costs, making biomass projects more attractive to investors. Some examples of incentives include:
- Feed-in Tariffs: These policies guarantee a fixed payment rate for biomass energy producers, ensuring a stable revenue stream. This long-term financial predictability encourages investment in biomass technologies.
- Tax Deductions: Governments may offer tax breaks to businesses that invest in biomass facilities. This reduces the overall financial burden, making it easier for companies to engage in biomass energy production.
- Research Grants: Funding for research and innovation plays a vital role in advancing biomass technologies. Grants can support the development of more efficient conversion methods and the creation of high-yield biomass crops.
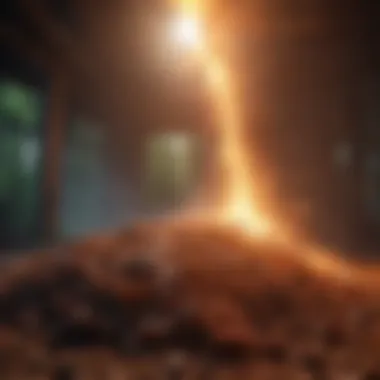
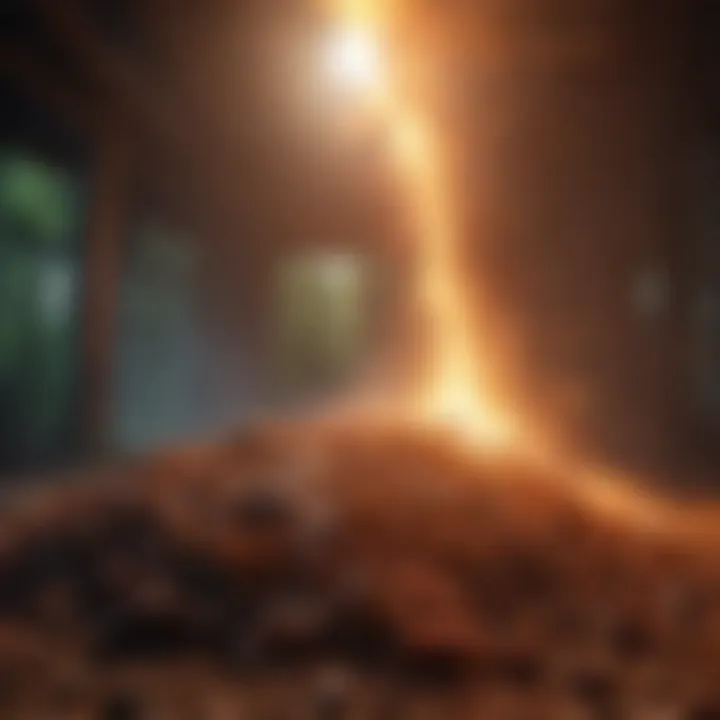
By providing tangible incentives, energy policies can stimulate biomass production, ultimately contributing to a more sustainable energy future.
"Policymakers need to craft regulations and incentives that harmonize economic, environmental, and social goals."
In summary, biomass and energy policy interplay significantly influences the development and adoption of biomass energy. Focused regulations and targeted incentives can unlock biomass's full potential, paving the way for innovative solutions that support a sustainable future.
Barriers to Biomass Energy Adoption
The adoption of biomass energy presents various challenges that can impede its growth and integration into broader energy markets. Understanding these barriers is crucial for identifying effective strategies to enhance biomass energy usage and its potential role in achieving sustainable energy goals. This section will address two primary barriers: technological limitations and public perception. By analyzing these factors, we can appreciate their influence on biomass energy's viability and assess ways to mitigate these challenges.
Technological Limitations
Biomass conversion to energy relies heavily on innovative technologies. However, significant technological barriers exist. The efficiency of conversion processes can vary widely, influenced by factors like feedstock composition and conversion technology. For example, thermochemical processes, though effective, often face challenges with the energy yield and the quality of the produced fuels.
- Lack of Standardization: Currently, no standardized methods exist for biomass conversion processes. This inconsistency complicates the scalability of biomass energy initiatives.
- Investment Constraints: Advanced biomass technologies often require substantial investment in research and development. Companies may hesitate to commit resources without clear economic incentives.
- Limited Infrastructure: The existing infrastructure may not adequately support large-scale biomass energy projects, making it difficult to collect, process, and distribute biomass efficiently.
These limitations can hinder the transition toward a more biomass-centric energy landscape. Solutions may involve increased collaboration between research institutions and industry stakeholders to spur innovation and share knowledge.
Public Perception and Awareness
Public perception of biomass energy plays a pivotal role in its adoption. Misinformation and a lack of awareness can breed skepticism among consumers and policymakers alike. For biomass to gain traction, it is essential to address these perceptions effectively.
- Environmental Concerns: Some view biomass as a lesser alternative to fossil fuels. Concerns about land use, deforestation, and emissions can lead to opposition against biomass projects. Public education campaigns can clarify that sustainably sourced biomass can provide environmental benefits compared to conventional sources.
- Outdated Knowledge: Many people may not be aware of advances in biomass technology. There is a need for comprehensive outreach to inform stakeholders about the efficiency and sustainability of modern biomass energy solutions.
- Community Engagement: Involving local communities in decision-making and development processes fosters trust. If people feel included and informed, they are more likely to support biomass energy initiatives.
Understanding the barriers to biomass energy adoption is essential. While technological limitations present challenges, addressing public perception can significantly impact the industry's future. By overcoming these barriers, we can unlock the full potential of biomass energy as a key player in the global energy transition.
Future Directions in Biomass Energy Research
In an era where energy challenges persist, research in biomass energy conversion stands at the forefront of innovation. The urgency to transition from fossil fuels to renewable energy sources compels scientists and policymakers to explore new pathways and enhance existing technologies. Focusing on future directions in biomass energy research is crucial for several reasons, including improved efficiency, cost-effectiveness, and sustainability. Moreover, it positions biomass as a viable player in the energy landscape, capable of addressing both local and global energy needs.
Innovative Biomass Conversion Technologies
Emerging technologies are reshaping the biomass conversion landscape. Research is increasingly directed towards refining existing processes such as pyrolysis, gasification, and anaerobic digestion. For example, advancements in gasification technology are making it possible to convert biomass into syngas, which can be further processed into diesel or hydrogen.
Researchers are also exploring methods to integrate machine learning and artificial intelligence in optimizing these processes. This could enhance efficiency and reduce operational costs. Additionally, developing biorefineries that can process multiple biomass inputs simultaneously presents exciting opportunities. They can produce a spectrum of bio-based products ranging from energy to pharmaceuticals, thereby maximizing value and reducing waste.
- Key Focus Areas in Innovative Technologies:
- Enhancements in syngas production and cleaning methods.
- Development of modular and scalable bioreactor designs.
- Investigation of thermochemical pathways optimizing energy extraction.
Potential for Advanced Biofuels
As the search for cleaner fuel sources intensifies, advanced biofuels offer a compelling alternative to traditional fossil fuels. Developing second- and third-generation biofuels, which utilize non-food biomass or waste materials, is a primary research focus. These biofuels generally have lower carbon footprints and do not compete with food supplies, making them more sustainable and acceptable.
Moreover, research in genetic engineering of microorganisms holds significant promise. By manipulating the metabolic pathways, scientists aim to produce biofuels more efficiently and with fewer resources. This may eventually lead to commercially viable biofuels that can replace gasoline or diesel, aligned with automotive standards.
"The advancement of biofuels is not just about meeting energy needs; it is about sustainability and environmental stewardship."
- Benefits of Advanced Biofuels:
- Reduced greenhouse gas emissions compared to conventional fuels.
- Energy security through domestic production.
- Diversification of agricultural products for farmers and landowners.
Supporting research initiatives and investments in biomass technology will yield significant environmental and economic gains. A commitment to reaching these goals ensures that biomass energy conversion remains a dynamic area of exploration for future energy solutions.
Case Studies in Biomass Energy
Examining case studies in biomass energy is crucial in understanding the practical applications and real-world implications of converting biomass to energy. These studies offer insight into successful implementations, challenges faced, and beneficial outcomes that can serve as a model for future endeavors. They highlight the innovations that arise from biomass projects and the importance of community involvement, technology development, and policy support.
Successful Biomass Projects Worldwide
Around the globe, numerous projects showcase the potential of biomass energy. These successful implementations demonstrate that biomass can be an effective and sustainable energy source.
- Drax Power Station, UK: Originally a coal-fired plant, Drax has converted a significant portion of its energy generation to biomass pellets, mainly from wood. This transformation reduced the plant's carbon emissions drastically while maintaining energy production levels.
- Biomass Energy Farm, USA: This facility uses agricultural residues, such as corn stover, to produce bioenergy through gasification. The project not only provides renewable energy but also supports local farmers by using waste materials.
- Agarwal Renewable Energy, India: Focusing on urban waste, this initiative converts municipal solid waste into biogas. The resultant energy is used to power local communities, showcasing an effective waste-to-energy system in a densely populated area.
These examples illustrate successful strategies in biomass conversion, indicating strong economic and environmental returns.
Lessons Learned from Early Biomass Initiatives
Early biomass projects provide valuable lessons that can enhance future efforts. Through examining past initiatives, stakeholders can identify key success factors and potential pitfalls. Some lessons include:
- Technology Adaptability: Many projects faced challenges due to rapidly changing technologies. Flexibility in adapting methods and equipment to improve efficiency is essential for sustained success.
- Community Engagement: Early projects often overlooked the importance of stakeholder involvement. Successful initiatives have since demonstrated that engaging local populations can enhance understanding and support for biomass projects.
- Economic Viability: Some early initiatives struggled with funding and financial models. It is critical for new projects to develop feasible economic plans, considering both initial investment and long-term operational costs.
"Learning from our predecessors is crucial for advancing the biomass sector. The insights gained through early initiatives can inform more effective strategies in current and future projects."
In summary, the examination of case studies in biomass energy elucidates both successful implementations and challenges faced in early projects. By analyzing these experiences, stakeholders in the biomass sector can harness past knowledge to propel future innovations, ensuring that biomass becomes an increasingly viable energy resource.
Closure
The conclusion of this article highlights the critical role of biomass energy in the modern energy landscape. As the world faces increasing challenges related to climate change, fossil fuel dependency, and sustainable development, biomass presents a plausible alternative. This conclusion synthesizes the diverse elements discussed throughout the article, emphasizing the multifaceted benefits of converting biomass to energy.
Summary of Key Findings
Biomass conversion technologies offer a robust framework for generating energy from organic materials. The main findings include:
- Diverse Biomass Sources: Various sources can be harnessed, including agricultural residues, organic waste, and dedicated energy crops.
- Efficient Conversion Methods: Different methods, such as gasification and anaerobic digestion, show considerable potential in efficiently converting biomass to energy.
- Economic Viability: The overall cost and market feasibility of biomass energy are increasingly favorable, aided by technological advancements and supportive policies.
- Environmental Considerations: Compared to fossil fuels, biomass energy can significantly reduce carbon emissions, provided that sustainable practices are implemented.
- Future Prospects: Innovations in biomass technology continue to emerge, suggesting a promising trajectory for further advancements in the sector.
Implications for the Future
Looking ahead, biomass energy holds substantial implications for global energy systems. Key considerations include:
- Sustainability Practices: For biomass energy to be a sustainable solution, careful management and sourcing of biomass resources are essential to avoid negative impacts on food security and biodiversity.
- Investment in Research: Increased funding for innovative biomass conversion technologies could lead to more efficient and scalable solutions, reducing costs and enhancing energy yields.
- Integration with Policies: Aligning biomass energy with environmental and energy policies can accelerate adoption and support marketplace competitiveness.
- Public Engagement: Raising awareness about the benefits and potentials of biomass energy can change perceptions and encourage acceptance of this alternative energy source.