ATP Luminescence: Mechanisms and Applications
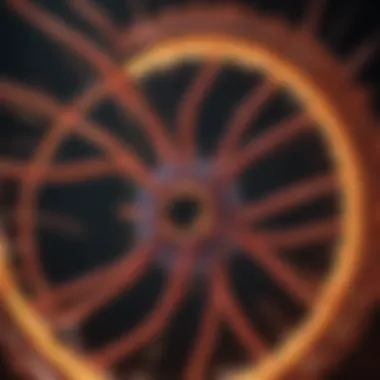
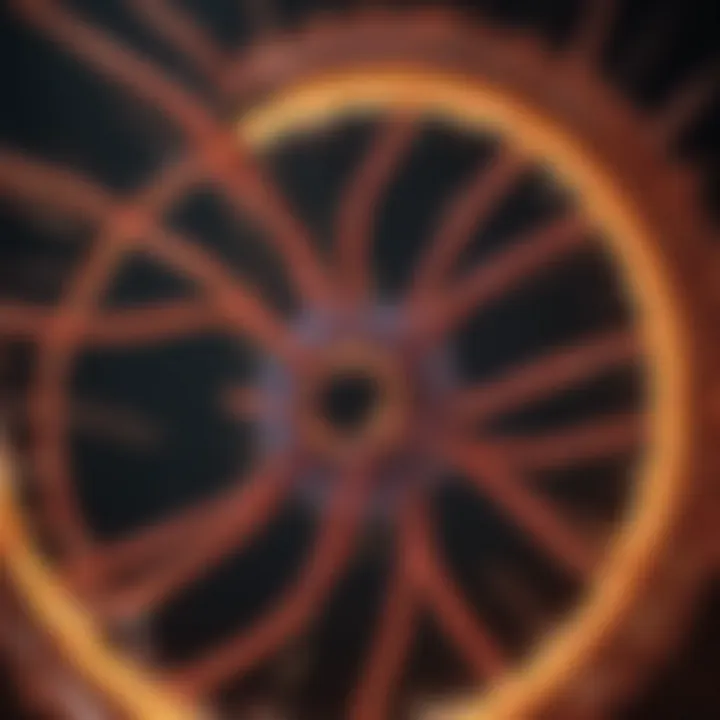
Intro
Adenosine triphosphate, commonly known as ATP, is often referred to as the energy currency of the cell. Its significance spans across numerous biological processes, acting as a key component in cellular metabolism and energy transfer. Recent advancements in biochemistry have unveiled another layer of ATP's role: its luminescence. This phenomenon, where ATP emits light, not only provides insights into metabolic activities but also holds potential for various scientific applications.
The mechanisms of ATP luminescence are intricately tied to its biochemical structure and interactions within biological systems. Understanding these mechanisms is crucial for discerning its applications in fields such as diagnostics, environmental monitoring, and biotechnological research. By revealing the critical roles of ATP luminescence, we can appreciate its transformative potential in contemporary biosciences.
Throughout this article, we will explore the key aspects of ATP luminescence, beginning with the methodologies that facilitate its study and application.
Methodology
Overview of research methods used
Understanding ATP luminescence requires a combination of biochemical assays, spectrophotometric methods, and advanced imaging techniques. Key research methods include:
- Bioluminescence assays: Utilize the natural light-emitting capacities of ATP molecules in conjunction with luciferin and luciferase to monitor metabolic activities.
- Fluorometric measurements: Assess the light emitted by ATP in varying concentrations, allowing for quantitative analysis of cellular processes.
- Imaging systems: Employ high-resolution cameras and sensors to visualize ATP presence in live cells, enhancing our understanding of cellular energy dynamics.
These methods uncover valuable data about ATP levels in different biological contexts, revealing correlations with metabolic health and cellular function.
Data collection techniques
Data collection for ATP luminescence studies often involves:
- Sample preparation: Cells or tissues are treated to extract ATP, ensuring minimal disruption to the metabolic indicators.
- Calibration: Accurate calibration of instruments is essential for precise luminescence measurements, often using known ATP concentrations as controls.
- Statistical analysis: Advanced statistical methods are employed to interpret data, allowing for robust comparisons of ATP luminescence under different experimental conditions.
Proper data collection techniques are integral to validating findings and ensuring reproducibility in research.
Applications
In scientific research
ATP luminescence serves as a sensitive indicator of cellular activity, providing vital information in various research domains, including:
- Cell metabolism studies: Identifying metabolic alterations in response to treatments or disease conditions.
- Drug efficacy testing: Assessing how different compounds impact ATP levels and, consequently, cellular health.
- Microbial monitoring: Evaluating contamination levels in environmental samples by measuring ATP as an indicator of viable cells.
In medical diagnostics
In medical diagnostics, ATP luminescence plays a role in:
- Detection of pathogens: Identifying bacterial or viral infections through quantification of ATP biomarkers.
- Cancer diagnostics: Monitoring tumor metabolism by assessing ATP generation in malignancies.
In environmental monitoring
For environmental monitoring, ATP luminescence is applied in:
- Water quality assessment: Measuring ATP in water samples to ascertain the presence of viable microorganisms.
- Soil health evaluation: Understanding soil microbial activity by tracking ATP levels as indicators of ecosystem vitality.
Future Directions
Upcoming trends in research
The future of ATP luminescence research appears promising, with emerging trends including:
- Integration with nanotechnology: Enhancing sensitivity and specificity through nanomaterials that interact with ATP molecules.
- Automation in assays: Development of automated systems for high-throughput analysis, facilitating large-scale studies.
Areas requiring further investigation
Despite the advancements, certain areas remain underexplored:
- Mechanistic insights: Further investigation into the biochemical pathways involved in ATP luminescence can elucidate its regulatory mechanisms.
- Field applications: More extensive studies are needed to evaluate real-world applications in agriculture and environmental science.
ATP luminescence not only serves as a key indicator of cellular energy status but also as a powerful tool in biotechnological research and environmental monitoring.
Foreword to ATP Luminescence
ATP luminescence represents a significant phenomenon in the realm of biochemical research. It serves as an indicator of metabolic processes and energy status in living organisms. Understanding ATP luminescence is crucial for various scientific domains, including biochemistry, molecular biology, and environmental science. This section aims to provide an essential overview that sets the stage for deeper exploration into the mechanisms and applications of ATP luminescence.
Defining ATP Luminescence
ATP luminescence is primarily defined as the light emission resulting from the chemical reactions involving adenosine triphosphate (ATP). This luminescence occurs when ATP is hydrolyzed, leading to energy release that can excite certain molecules. The subsequent return to a lower energy state results in the emission of light, a process that can be quantified and analyzed. This property has made ATP a valuable tool in scientific methodologies, particularly in measuring biological activity. The clear association between ATP levels and cellular vitality makes ATP luminescence a cornerstone in assessing metabolic states.
Historical Context and Discovery
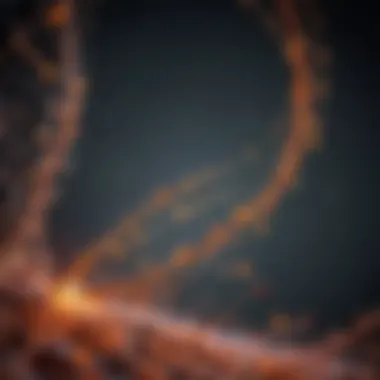
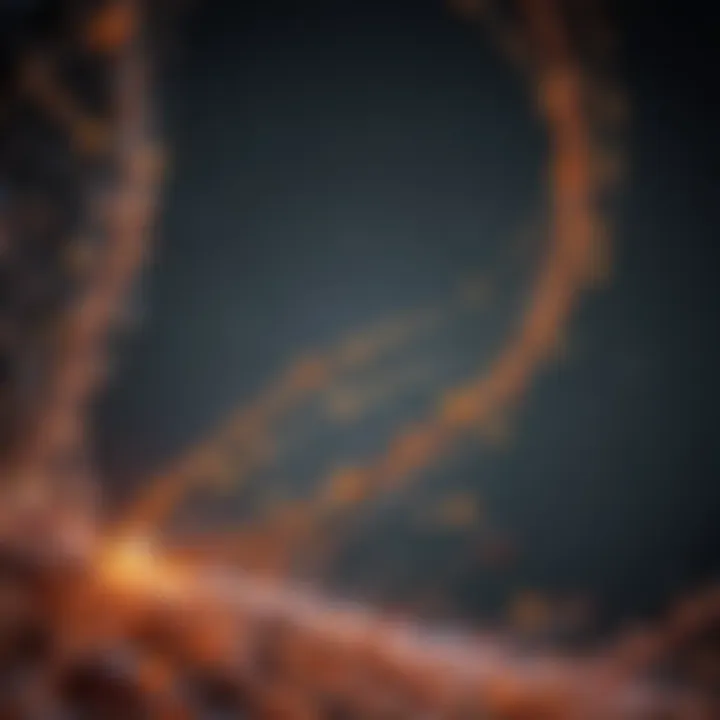
The journey of ATP luminescence began with the discovery of ATP itself in 1929 by Karl Lohmann. Its role as an energy carrier in cells was established through research that connected ATP to vital biological processes. As studies progressed, scientists noticed that ATP could emit light during certain reactions. This observation led to the detailed exploration of luminescence as a measurable indicator of biological activity. Various techniques have since evolved to harness this property, facilitating significant progress in both basic and applied research. Understanding its history includes recognizing the pivotal discoveries that have laid the groundwork for contemporary applications in diagnostics and environmental assessments.
"ATP luminescence provides a real-time insight into cellular processes, making it invaluable in current biomedical research."
Through these historical insights, we see that ATP luminescence is not just a modern tool but a culmination of decades of scientific inquiry. As we proceed, further dissecting the biochemical basis and applications of ATP luminescence reveals its versatility and importance in the biological sciences.
Biochemical Basis of ATP
Understanding the biochemical basis of ATP is essential in comprehending how this molecule powers life. ATP, or adenosine triphosphate, acts as a universal energy currency within cells. Its structure consists of an adenosine molecule bonded to three phosphate groups, which are vital for its role in energy transfer. When ATP breaks down into ADP (adenosine diphosphate) and inorganic phosphate, energy is released. This energy is crucial for a variety of cellular processes, making ATP a central topic in both metabolism and bioluminescence.
Synthesis of ATP
Cellular respiration
Cellular respiration is a primary method through which cells generate ATP. This process involves a series of metabolic pathways, namely glycolysis, the Krebs cycle, and oxidative phosphorylation. Glycolysis, which occurs in the cytoplasm, converts glucose into pyruvate, producing a small amount of ATP in the process. This initial step sets the stage for further ATP generation through the Krebs cycle in the mitochondria, where pyruvate is fully oxidized.
One key characteristic of cellular respiration is its efficiency. This processes allows not only the production of ATP but also other important metabolic intermediates. The unique feature of aerobic cellular respiration is its ability to maximize ATP yield to 36 or 38 ATP molecules per glucose molecule under optimal conditions, making it a clear benefit for high-energy-demand cells like neurons and muscle tissues. However, the dependency on oxygen can limit this process in anaerobic conditions, which is a disadvantage in certain environments.
Photophosphorylation
Photophosphorylation is another method of ATP synthesis, primarily observed in plants and some bacteria. This occurs during photosynthesis, where light energy converts ADP and inorganic phosphate back into ATP. The light-dependent reactions capture photons, utilizing them to generate ATP through an electron transport chain situated in the thylakoid membranes of chloroplasts.
The major characteristic of photophosphorylation is its reliance on solar energy. This distinctly positions it as a renewable source for ATP production, unlike the finite resources of organic substrates in cellular respiration. However, its efficacy can be impacted by light availability and can be less efficient in certain environmental conditions. Despite this, photophosphorylation plays a pivotal role in ecosystems, supporting life through the conversion of light energy into chemical energy.
Role of ATP in Cellular Functions
Energy transfer mechanisms
ATP plays a fundamental role in cellular energy transfer mechanisms. Once synthesized, ATP acts as a molecular shuttle, transporting energy to various cellular processes that require it. The energy released from ATP hydrolysis is used to perform work such as muscle contraction, transmit nerve impulses, and drive biosynthetic reactions.
The distinctiveness of energy transfer mechanisms involving ATP is its ability to provide an immediate energy source that is readily available for cellular functions. This rapid energy supply is crucial for maintaining cellular metabolism and overall homeostasis. Nonetheless, the reliance on ATP can lead to quick depletion under high-energy demand scenarios, requiring effective replenishment methods to sustain activities.
Proteins and enzymatic reactions
Proteins and enzymes depend significantly on ATP for their functions. ATP not only provides energy for these biological catalysts but also serves as a regulator. Many enzyme reactions are coupled with ATP hydrolysis, and ATP can modulate protein activity through phosphorylation, affecting their function and interaction with other biomolecules.
What makes the role of ATP in protein and enzymatic reactions vital is its involvement in regulating metabolic pathways. Phosphorylation can activate or deactivate enzymes, illustrating ATP's regulatory capacity. However, excess ATP can lead to unintended activation of pathways, resulting in imbalances within the cell, illustrating both the power and the potential pitfalls of ATP's involvement in cellular functions.
Mechanisms of ATP Luminescence
Understanding the mechanisms of ATP luminescence is crucial in deciphering the roles of adenosine triphosphate in biological systems. ATP luminescence provides researchers with insights into metabolic activities and cellular health. By studying its mechanisms, one can effectively measure energy levels and monitor various biological processes. It opens avenues for applications in medical diagnostics, environmental monitoring, and biotechnological innovations.
Photophysical Properties of ATP
Electron transitions
Electron transitions in ATP are vital for its luminescent properties. When ATP absorbs light, the electrons within its molecules gain energy, moving to a higher energy state. This characteristic makes ATP a useful molecule for luminescence studies. The unique feature of electron transitions is their ability to indicate energy changes within cells. This often benefits researchers in examining metabolic activities. However, the influence of environmental factors on these transitions can pose challenges for accurate readings.
Emission wavelengths
The emission wavelengths of ATP luminescence also play a significant role. When electrons return to their ground state, they release energy in the form of light. This emitted light has specific wavelengths that can be measured. The key characteristic of emission wavelengths is the ability to differentiate between various biochemical activities based on their spectral signatures. This feature serves as an advantage, allowing for more precise analysis of cellular processes. Nonetheless, variations in emission wavelengths due to changes in pH or temperature may affect measurement reliability.
Chemiluminescence Versus Bioluminescence
Chemiluminescence and bioluminescence are two important concepts in the context of ATP luminescence. Chemiluminescence refers to the light produced through a chemical reaction without the involvement of biological systems. In contrast, bioluminescence is a type of chemiluminescence that occurs in living organisms, including those containing ATP.
- Key Differences:
- Source: Chemiluminescence comes from synthetic reactions, while bioluminescence is generated in nature.
- Applications: Bioluminescence is often used in biological assays, while chemiluminescence is more common in laboratory settings outside of biological contexts.
Both processes highlight the versatility of luminescent reactions in scientific research. Their distinctions help researchers choose appropriate methodologies for studies involving ATP.
ATP luminescence serves multifaceted purposes across various fields, providing essential insights into metabolic activities and overall cellular health.
Applications of ATP Luminescence
In the landscape of biological science, the applications of ATP luminescence represent a powerful methodology with far-reaching implications. This technique harnesses the inherent properties of adenosine triphosphate (ATP) to provide insights into various biological processes. Its utility spans assessments of energy metabolism, the viability of cells, environmental monitoring, and more. The importance of ATP luminescence lies primarily in its sensitivity and specificity, making it an advantageous option in both research and applied sciences.
ATP in Bioluminescence Assays
Cell viability testing
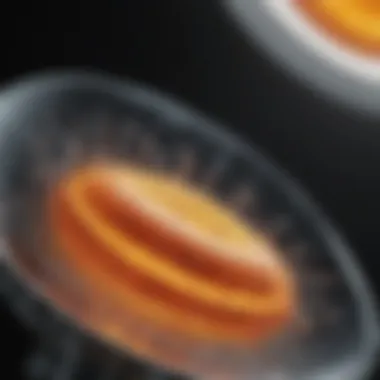
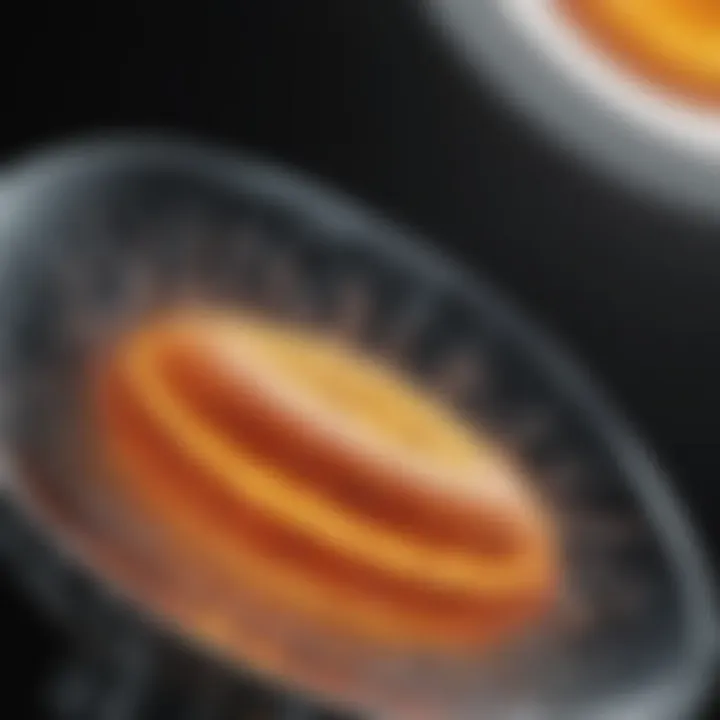
Cell viability testing through ATP luminescence has gained prominence in laboratory settings. This method quantifies the amount of ATP present in a sample, which correlates directly with the number of viable cells. The key characteristic of this assay is its rapidity; results can often be obtained in a matter of minutes. This immediacy is beneficial for researchers aiming to evaluate the effects of drugs or treatments on cells.
A unique feature of this testing method is its non-invasive nature, which preserves the integrity of the sample for further analysis. However, potential disadvantages may include interference from external factors, such as lysed cells releasing ATP, which could lead to misleading results.
Enzyme activity measurement
Measuring enzyme activity using ATP luminescence offers a sensitive approach to visualize enzymatic reactions. This method relies on the generation of ATP as a product of enzymatic activity, linking it directly to the luminescent output. It is popular because it provides real-time analysis of enzyme function, which is crucial in various biotechnological applications and enzyme kinetics studies.
One noteworthy feature is its ability to detect low levels of enzyme activity that would be challenging to capture through traditional methods. However, this technique may require careful calibration to avoid false positives, thus demanding attention to detail in experimental design.
Environmental Monitoring Using ATP Readings
Microbial assessment
Microbial assessment through ATP luminescence presents a practical approach to evaluate microbial presence in a variety of environments. In this context, ATP measurements reflect the metabolic activity of microorganisms, providing a snapshot of their viability. This method is particularly useful in sectors like food safety, where monitoring microbial load is essential.
The key characteristic of microbial assessment is its rapid turnaround compared to culture-based methods, which can take days. Moreover, the unique feature of this approach lies in its capacity to detect viable but non-culturable organisms, thus offering a more comprehensive view of microbial health. However, it might be limited by the specificity of ATP to different microbial groups, requiring companion tests for precise identification.
Contaminant detection
Contaminant detection using ATP luminescence serves as an efficient method to indirectly measure the cleanliness of surfaces and water sources. In ecological studies, ATP readings can help identify the presence of pollutants that affect biological organisms. The sensitivity of this method is a standout benefit; it can detect minute contaminant levels that traditional approaches might miss.
However, this technique has its limitations, including the potential for false positives due to the presence of naturally occurring ATP, leading to potential overestimation of contamination levels in some environments.
Clinical Applications of ATP Measurements
Diagnostic tools
In the clinical realm, ATP measurements are leveraged for diagnostic tools to assess health status. The quantities of ATP in body fluids can indicate metabolic disorders or infection. The key characteristic of this application is its non-invasive nature, offering a less intrusive alternative to traditional diagnostic methods. Additionally, it allows for quick results, facilitating timely decisions in patient care.
The unique feature of ATP-based diagnostics is that they can be integrated with portable devices, enabling immediate testing in various settings. However, one potential disadvantage includes distinguishing normal from pathological levels, which requires comprehensive reference ranges.
Therapeutic monitoring
Therapeutic monitoring through ATP measurements plays a significant role in managing patient treatments. By tracking ATP levels, clinicians can evaluate the efficacy of therapies, especially in conditions that severely affect cellular metabolism. The principal advantage of this approach is that it provides insights into patient response over time. Additionally, it can serve as a guide for dose adjustments in therapeutic regimens.
Nevertheless, a challenge of therapeutic monitoring via ATP is the variability of ATP levels across different individuals and conditions, indicating that personalized baselines are essential for accurate interpretation.
"Understanding the various applications of ATP luminescence not only enriches our knowledge base but also underlines its clinical and environmental significance."
In summary, the applications of ATP luminescence are diverse and integral to many fields within biological sciences. Its ability to provide quick, reliable data enhances decision-making processes across research, environmental monitoring, and clinical practice.
Technological Advances in ATP Detection
Technological advances in ATP detection have greatly influenced the field of bioluminescence and biological research. The ability to accurately determine ATP levels is crucial for a multitude of applications ranging from environmental monitoring to clinical diagnostics. This advancement not only improves measurement precision but also offers significant benefits in terms of efficiency and accessibility.
Innovations in Luminescence Technology
Detection methods
Detection methods for ATP luminescence typically rely on the measurement of light emitted in the presence of ATP and its dependent enzymatic reactions. The use of luciferase enzyme, for instance, is a common method which catalyzes the reaction of ATP and luciferin, resulting in a quantifiable light emission. This is a beneficial approach due to its high sensitivity and fast response time.
The unique feature of this method is its ability to provide real-time data on cellular metabolism, which is vital for assessing viability in cell cultures. However, one must consider the limitations regarding interference from other luminescent substances in complex biological samples. Thus, while effective, the method requires careful calibration and validation.
Instrumentation advancements
Instrumentation advancements play a key role in enhancing ATP detection capabilities. Modern photometers and luminometers offer improvements in sensitivity and accuracy. These instruments are capable of detecting lower levels of ATP against a background of noise, which positively influences the reliability of results obtained. This characteristic is advantageous because it allows for the detection of subtle changes in ATP concentration, reflective of metabolic activity.
The unique aspects of the current instrumentation include automated systems that can handle high-throughput screening, facilitating large-scale studies. However, the challenge lies in the initial cost of these High-end instruments, which may not be affordable for all laboratories. Thus, accessibility remains a concern despite the technological enhancements.
Emerging Research Frontiers
Emerging research frontiers in ATP detection are expanding possibilities, particularly in the realms of nanomedicine and bioengineering applications.
Nanomedicine
Nanomedicine focuses on utilizing nanoscale materials for medical applications. In ATP detection, nanosensors have been developed that can detect ATP at very low concentrations. The key characteristic of these nanosensors is their high sensitivity and specificity, which makes them suitable for various diagnostic purposes.
A unique feature of nanomedicine is the potential for targeted delivery. These sensors can provide localized readings of ATP levels, giving insights into cellular environments. However, the complexity of developing biocompatible nanoparticles poses a significant challenge, potentially impacting their practical application in clinical settings.
Bioengineering applications

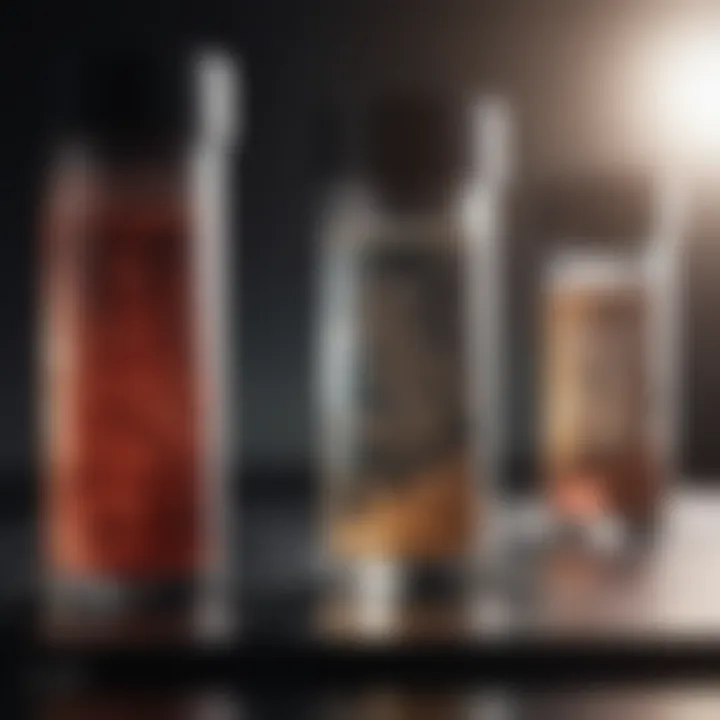
Bioengineering applications incorporate ATP luminescence to design biosensors that provide real-time feedback on cellular activities. Such systems can be adapted for various uses, from monitoring metabolic states to evaluating cell growth. The key benefit of these applications is their ability to integrate seamlessly into existing biological frameworks, enabling dynamic assessments.
The notable feature of bioengineering in this context is the creation of smart biosensors that can respond to changes in ATP levels autonomously. However, complexity in the designs can lead to challenges in reliability and ease of use, which remains a central issue for future developments.
Limitations and Challenges in ATP Luminescence Research
Understanding the limitations and challenges in ATP luminescence research is vital for advancing our knowledge and applications of this area. While ATP luminescence offers several benefits, such as its high sensitivity and relatively rapid response, researchers must navigate certain obstacles that may affect the accuracy and reliability of their measurements. This section delves into these issues, touching on interference factors and standardization, to shed light on the current state of research in this field.
Interference Factors in Measurements
Interference factors can significantly impact the validity of ATP luminescence measurements. These factors can arise from various sources, ultimately introducing noise into the data collected. Here are key elements to consider:
- Contaminants: Extraneous substances within biological samples can interact with the components of the assay, causing deviations in luminescence readings. Substances such as ions, proteins, or other molecular species can reduce precision.
- Environmental Conditions: Factors like temperature, pH, and ionic strength influence the reaction kinetics of ATP with luciferase enzymes. For instance, suboptimal pH levels might alter ATP's luminogenic activity, resulting in underreporting of ATP concentrations.
- Temporal Dynamics: The timing of measurements is crucial. If samples are taken too late post-reaction initiation, the luminescent output may decrease, giving a false impression of ATP levels. Proper timing ensures that luminescence correlates accurately with ATP presence.
To combat these interference factors, stringent control measures must be established. Researchers are adapting methodologies to eliminate contaminants and precisely monitor environmental conditions to ensure that results accurately represent ATP levels.
Standardization Issues
Standardization is another pressing challenge in ATP luminescence research. Given the diversity of methods and protocols employed across laboratories, achieving consistent results remains a hurdle. The following points are essential regarding standardization:
- Diverse Protocols: Different laboratories employ varied protocols for measuring ATP luminescence. This inconsistency can lead to discrepancies in data interpretation, making it difficult to compare findings across studies.
- Calibration Standards: The lack of universally accepted standard calibration conditions further exacerbates the issue. Instead of a single reference point, variations in brand and type of reagents can yield different outcomes. Without standardization, reproducibility is compromised.
- Variation in Equipment: The equipment used for ATP detection also varies. Differences in luminescence readers and detection systems can introduce variability in data. These inconsistencies necessitate a more unified approach to methodology, ensuring that all labs operate under similar conditions.
By addressing these standardization issues, the scientific community can enhance the comparability of research findings, ultimately leading to a more reliable application of ATP luminescence in various fields.
Addressing interference factors and standardization issues in ATP luminescence could pave the way for innovations that enhance the reliability of scientific research.
Future Directions in ATP Luminescence Studies
Exploring the future of ATP luminescence studies is essential. This area holds great potential for advancements in various scientific domains. Understanding ATP luminescence better can lead to significant improvements in biotechnology and medical diagnostics. Researchers are seeking new methodologies and applications to utilize ATP as a tool for deeper insights into biological processes.
Potential for Novel Applications
Integration into Metabolic Engineering
Integrating ATP luminescence into metabolic engineering offers promising opportunities. It can serve as a real-time monitor of cell metabolism, crucial for optimizing metabolic pathways. One key characteristic is its ability to provide immediate feedback on ATP levels during engineering processes of microorganisms or cell cultures. This real-time monitoring is beneficial because it helps researchers adjust conditions for maximum yield in bioproduction.
A unique feature of using ATP luminescence is that it links energy status directly to productivity. This direct correlation can improve efficiency in producing pharmaceuticals or biofuels. However, the dependency on precise measurement can present challenges. Misinterpretations of ATP readings can occur if not properly calibrated.
Smart Biosensors
Smart biosensors represent another innovative application of ATP luminescence. These biosensors can integrate with existing technology to monitor ATP levels continuously in various environments. The key aspect is their ability to be miniaturized and deployed in situ, providing immediate ATP level readings from biological samples. This makes them a popular choice for real-time data collection in environmental and clinical settings.
The unique feature of smart biosensors is their adaptability to different detection platforms. They can be tailored for specific experiments, offering advantages like quick results and easy data integration. Nonetheless, the complexity of developing these sensors may hinder deployment in some scenarios. Calibration and specificity remain critical to ensure accurate readings for varying conditions, which can be a limitation.
Collaborative Research Approaches
Interdisciplinary Studies
Interdisciplinary studies are vital in advancing ATP luminescence research. Combining expertise from biology, chemistry, engineering, and computer science creates comprehensive perspectives on ATP applications. This collaboration enhances innovation and application of ATP luminescence across fields. One notable characteristic is the cross-pollination of ideas, leading to novel research initiatives.
Interdisciplinary collaborations are crucial for addressing complex biological questions. They allow for a more holistic approach, such as integrating computational models to predict biological outcomes. However, coordinating diverse teams can be challenging. Variance in terminology and methodology can lead to misunderstandings or conflicts, requiring effective communication strategies.
Global Research Initiatives
Global research initiatives are gaining importance in the realm of ATP luminescence studies. Collaborative efforts on an international scale allow researchers to share resources, data, and best practices. One critical benefit of these initiatives is the expedited progress in understanding global health issues and environmental impacts.
The unique strength of global initiatives lies in their ability to pool expertise from various regions, fostering innovation and adaptation. These collaborations can enhance the effectiveness of ATP-based methods in diverse settings. Still, logistical challenges in coordination and funding can affect project execution and timelines, which researchers must navigate.
Future investigations into ATP luminescence not only promise to deepen our understanding of biological systems but can also lead to transformative applications that impact healthcare and environmental management.
Culmination
In this article, we have explored the multifaceted roles of ATP luminescence within biological systems. This luminous phenomenon is rooted in the biochemical properties of adenosine triphosphate (ATP), revealing its significance in various cellular processes and applications. The importance of ATP luminescence cannot be overstated; it serves not only as an indicator of energy metabolism but also as a versatile tool across diverse fields such as biochemistry, environmental science, and clinical diagnostics.
Summary of Key Insights
ATP luminescence emerges as a pivotal mechanism to understand cellular vitality and energy state. We examined:
- The biochemical foundation of ATP synthesis and its crucial role as an energy currency in living organisms.
- The photophysical properties that enable ATP luminescence, highlighting the significance of electron transitions and emission wavelengths in the detection process.
- Applicative dimensions ranging from bioluminescence assays to microbial monitoring and clinical diagnostics, demonstrating the vast utility and relevance of ATP measurements in real-world scenarios.
- Technological advancements that have significantly improved the precision and range of ATP detection methodologies, further enhancing its application in research.
These insights collectively underscore the versatility of ATP luminescence as a powerful tool in the life sciences. By quantifying ATP levels, researchers can infer cellular health and activity, providing essential data in various experimental setups.
The Role of ATP Luminescence in Future Research
Looking ahead, ATP luminescence holds promise for several innovative applications:
- Integration into metabolic engineering: Utilizing ATP measurement to enhance bioengineered systems can lead to more efficient metabolic pathways and increased yield of desired products.
- Developing smart biosensors: These devices, powered by ATP luminescence, can provide real-time assessments of metabolic activities in complex biological environments.
- Interdisciplinary studies: Collaborations across fields such as molecular biology, environmental science, and clinical research can facilitate the development of novel technologies that rely on ATP luminescence.