Recent Advancements in Genetic Research: An Overview
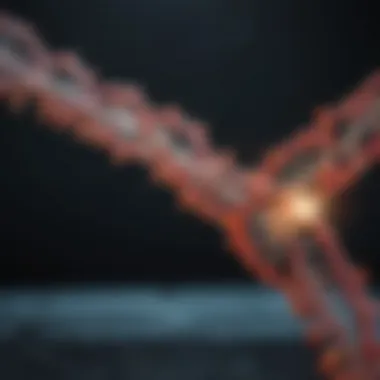
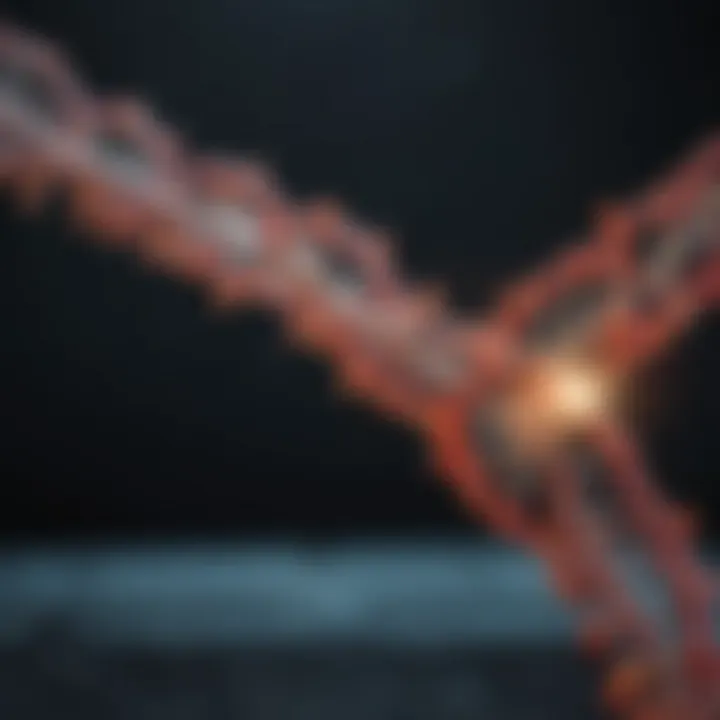
Intro
Genetics remains a pivotal field of study that shapes our understanding of life at a molecular level. With rapid advancements in research tools and methodologies, the landscape of genetics is undergoing significant transformation. This article aims to provide a thorough overview of current developments in genetics, focusing on gene editing technologies, notably CRISPR, and their applications in personalized medicine and agricultural innovations. Ethical considerations and implications also play a crucial role in discussions surrounding these advancements. Understanding the intricacies of genetic research is essential not only for scientists but also for educators, students, and professionals in various fields.
In this narrative, we will dissect the relevant methodologies employed in genetic studies, explore future directions that the field is taking, and highlight the deeper implications of genomic research on health and agriculture. Each section will offer insights that reflect breakthroughs and challenges, contributing to a well-rounded comprehension of contemporary genetics.
Preamble to Recent Research in Genetics
The field of genetics has witnessed rapid evolution in both technology and understanding over recent years. This makes the introduction to recent research in genetics a crucial element of scientific discourse. Genetic research helps elucidate the mechanisms underlying hereditary traits, diseases, and complex biological functions. By focusing on the most contemporary findings, we can better appreciate how genetics intersects with other scientific domains. This intersection is key to groundbreaking innovations in health, agriculture, and natural science.
Historical Context of Genetic Studies
Historically, genetics as a scientific discipline began with Gregor Mendel's experiments in the mid-nineteenth century. His work laid the groundwork for understanding inheritance patterns. For many decades, research in genetics remained primarily foundational, focusing on classical Mendelian principles. As technology advanced, so too did our understanding of the genetic code. The discovery of DNA’s structure in 1953 by James Watson and Francis Crick marked a pivotal moment, linking biochemistry to genetic heredity.
Over the next few decades, various technologies emerged, including polymerase chain reaction (PCR) and DNA sequencing techniques. These innovations set a framework that enabled researchers to explore genetic information at unprecedented scales and to study the genetic basis of diseases more effectively.
As we entered the 21st century, initiatives like the Human Genome Project further transformed our understanding of genetics, enabling the mapping of human DNA sequences. This historical context provides an essential backdrop for discussions about the current focal points in genetic research, emphasizing the importance of both foundational knowledge and recent advancements.
Significance of Current Research
Current research in genetics signifies the culmination of years of accumulated knowledge and technological advancements. The importance cannot be understated, as it encompasses various aspects of life—healthcare, agriculture, and biodiversity, to name a few. For instance, advancements in gene editing techniques like CRISPR-Cas9 have opened new avenues for treating genetic disorders. Similarly, the applications of genomics in personalized medicine tailor treatments to individual genetic profiles, creating more effective health strategies.
Moreover, understanding population genetics is critical for preserving biodiversity and ensuring food security through improved agricultural practices. Current inquiries into genetic factors influencing disease susceptibility have far-reaching implications for public health initiatives.
In essence, the continued evolution of genetic research is vital for numerous real-world applications. Staying abreast of recent findings is essential for scientists, healthcare professionals, and policymakers alike, ensuring that developments in genetics align with ethical standards and community needs.
"Genetics is the blueprint for life, and recent research continues to rewrite that blueprint with every advanced discovery."
This quote underscores the transformative potential of modern genetic research, inviting deeper exploration into the specific innovations reshaping the field today.
Innovations in Genetic Techniques
The realm of genetic research is marked by transformative innovations that redefine how scientists understand and manipulate the building blocks of life. This section elucidates key advancements in genetic techniques, right from groundbreaking technologies to practical applications. Understanding these techniques is crucial, as they not only advance genetic studies but also hold immense potential for future applications in medicine, agriculture, and biotechnology.
CRISPR-Cas9 Technology
CRISPR-Cas9 has taken center stage in genetic modification. This technology allows researchers to edit genes with unprecedented precision. By utilizing the natural defense mechanisms of bacteria against viruses, scientists have adapted this system for genetic engineering. One of the undeniable benefits of CRISPR is its efficiency. Traditional methods of gene editing often require extensive time and resources. CRISPR, on the other hand, streamlines the process.
The implications of CRISPR reach far beyond the laboratory. This tool has opened up possibilities for treating genetic disorders. For instance, researchers are investigating its use in conditions like sickle cell anemia and muscular dystrophy. Targeting faulty genes could ultimately lead to cures that were previously thought unattainable. However, this technology is not without its risks. Concerns regarding off-target effects—the unintentional alteration of unintended genes—highlight the need for rigorous studies to ensure safety.
"CRISPR-Cas9 offers a new frontier in genetically targeted therapies, yet caution in its application is paramount."
Gene Therapy Developments
Gene therapy is another notable advancement in genetic techniques. It involves correcting defective genes responsible for disease development. Unlike traditional treatments that often address symptoms, gene therapy seeks to rectify the problem at its source. This approach has shown promise in treating various genetic disorders, including hemophilia and certain types of inherited retinal diseases.
Recent developments have refined the methods of delivering therapeutic genes. Viral vectors are commonly used to introduce new genetic material into a patient's cells. Researchers are also innovating with non-viral methods, which might reduce the risk of immune responses associated with viral vectors. The use of nanoparticles, for example, is gaining traction.
Moreover, clinical trials are expanding to include more complex diseases. Scientists are beginning to explore gene therapy's potential in combating conditions like cancer. The successes and failures of these trials will significantly shape the future of gene therapy. Evaluating not just efficacy but long-term outcomes is critical as therapies enter the mainstream.
In summary, innovations in genetic techniques, particularly CRISPR-Cas9 and gene therapy, encapsulate a rapidly evolving landscape. These advancements offer impactful avenues for research and application, while also presenting ethical and safety considerations that must be thoughtfully navigated.
Advancements in Genomic Sequencing
Genomic sequencing has seen significant progress over the last few years. This evolution is crucial due to its implications for various fields such as medicine, agriculture, and evolutionary biology. Advancements in this area not only enhance our understanding of genetics but also allow for more precise interventions in health and disease management.
One of the hallmark advancements in genomic sequencing is the advent of next-generation sequencing (NGS). This technology has transformed how researchers gather genetic data. Unlike traditional sequencing methods, NGS can process millions of fragments concurrently, providing a comprehensive overview of an organism's DNA. This ability drastically reduces the time and cost involved in sequencing genomes, making it accessible for a wider range of studies.
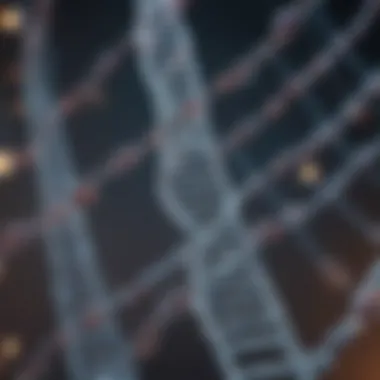
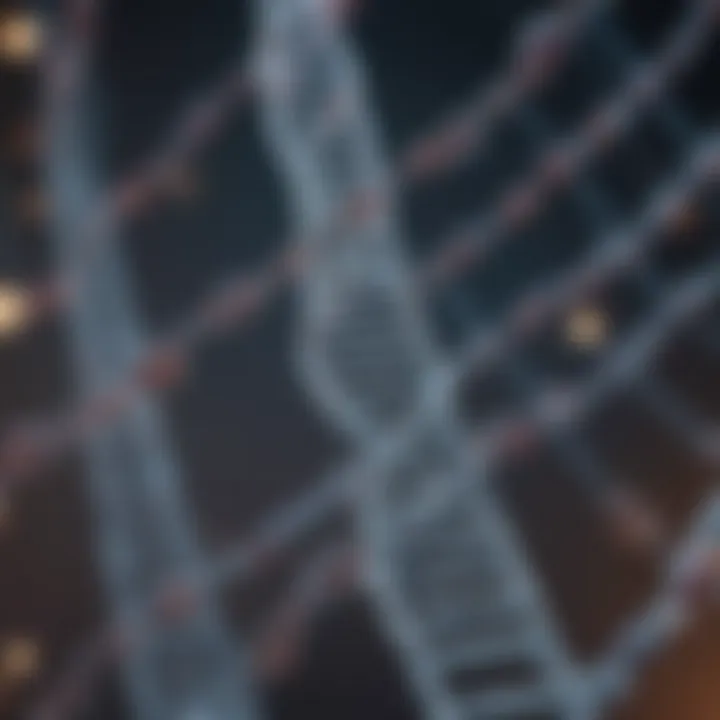
The benefits of NGS are manifold. It enables researchers to explore genetic variations within populations, identify genetic markers linked to diseases, and analyze complex genetic interactions. The result is a nuanced understanding of genetic predispositions and traits. Furthermore, NGS is pivotal in personalized medicine, where tailored treatment plans can be designed based on an individual’s unique genetic makeup.
Next-Generation Sequencing
Next-generation sequencing has established itself as a cornerstone of modern genomic research. It offers three main advantages: speed, accuracy, and cost-effectiveness. With NGS, scientists can sequence an entire genome in a matter of days, compared to months or even years with older techniques. This rapid processing facilitates real-time data analysis, essential for research pushing the boundaries of genetics.
The accuracy of NGS also deserves mention. By employing parallel sequencing techniques, the chances of errors are significantly reduced, leading to more reliable results. Additionally, the cost associated with sequencing has decreased dramatically. The Human Genome Project, for instance, had a budget of nearly $3 billion for sequencing the first human genome; now, the cost is less than $1,000 per genome, opening doors for large-scale genomic studies.
Applications in Disease Research
The applications of advancements in genomic sequencing within disease research are profound. By understanding the genetic basis of diseases, researchers can identify novel biomarkers for early diagnosis and treatment. For example, in cancer research, NGS is pivotal in identifying mutations that drive tumor growth, enabling oncologists to develop more effective targeted therapies.
Genomic sequencing also plays a critical role in understanding infectious diseases. Sequencing the genomes of pathogens provides insights into their evolution and transmission patterns. This information is vital for developing vaccines and public health interventions. In light of recent global health events, such as the COVID-19 pandemic, the ability to rapidly analyze genetic data from viruses is invaluable in tracking outbreaks and identifying potential mutations.
"Genomic sequencing serves as a tool that not only aids in the understanding of health but also transforms the realms of diagnosis and treatment."
Population Genetics and Its Implications
Population genetics serves as a crucial branch of genetics. This field investigates the distribution and change in allele frequencies within populations over time, shaped by various evolutionary forces. Understanding population genetics is vital for comprehending the genetic basis of adaptation and evolution. It also has significant implications for conservation biology, agriculture, and human health.
Understanding Genetic Diversity
Genetic diversity is the variation in the genetic composition among individuals within a population. This diversity is important because it affects a population's ability to adapt to changing environments and challenges, such as diseases and climate change. Higher levels of genetic diversity can increase resilience, giving a population a better chance for survival.
In many species, especially those that are endangered, monitoring genetic diversity helps in developing strategies for conservation. Tools such as genetic markers assist researchers in assessing the genetic variation within and between populations. This information informs management decisions, guiding breeding programs and habitat restoration efforts.
"Conservation strategies often rely on population genetic data to ensure the survival of species amidst environmental changes."
Evolutionary Studies and Adaptation
Evolutionary studies in population genetics focus on how genetic changes occur in response to selective pressures. Natural selection, genetic drift, and gene flow all play critical roles in shaping the evolutionary trajectory of populations. Researchers investigate these mechanisms to understand how species adapt to their environment over generations.
The study of population genetics has led to significant discoveries about human evolution, notably regarding migrations and the impact of historical events on genetic diversity. For example, genetic studies reveal how past populations interacted, migrated, and adapted to new environments.
Furthermore, understanding adaptation at the genetic level can lead to breakthroughs in medicine, agriculture, and biodiversity conservation. By studying the genetic underpinnings of resilient traits, scientists can develop crops that withstand environmental stressors and create targeted medical therapies based on genetic predispositions.
Through these insights, population genetics plays a critical role in various fields, making it an indispensable area of research in genetics.
Ethical Considerations in Genetic Research
Genetic research has brought about significant advancements, yet it also raises pressing ethical questions. This section explores various aspects of ethics relevant to genetics, emphasizing the need for careful and responsible practices in this rapidly evolving field. Understanding the ethical implications is crucial for researchers, policymakers, and the public alike, as they navigate the complexities of genetic manipulation and its consequences.
Debates on Gene Editing Ethics
The advent of gene editing technologies, particularly CRISPR-Cas9, has triggered extensive debates regarding their ethical use. Some argue that the potential benefits of gene editing, such as curing genetic disorders and enhancing health outcomes, outweigh the risks. Others caution against the unforeseen consequences of altering genomes.
Key points of contention include:
- Designer Babies: The idea of creating genetically enhanced humans raises moral questions. Should parents have the right to choose their children’s traits? What are the implications on equality and societal norms?
- Short- and Long-term Effects: While immediate results may be promising, long-term impacts on individuals and generations remain unclear.
- Access and Inequality: As gene editing technologies become available, there is concern about who can access these advancements. Will they only be available to the wealthy?
"The power to edit genes presents a double-edged sword; we must tread carefully."
Regulatory Frameworks and Guidelines
Establishing regulatory frameworks is essential to govern genetic research responsibly. Different countries have varying regulations concerning genetic modification, which can lead to inconsistencies in research practices.
Significant considerations for regulatory frameworks include:
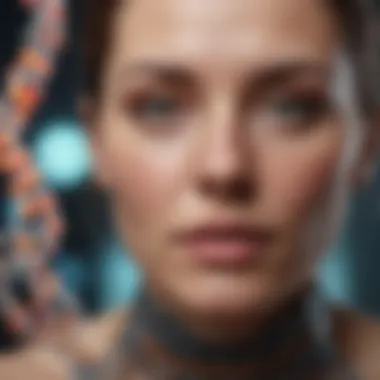
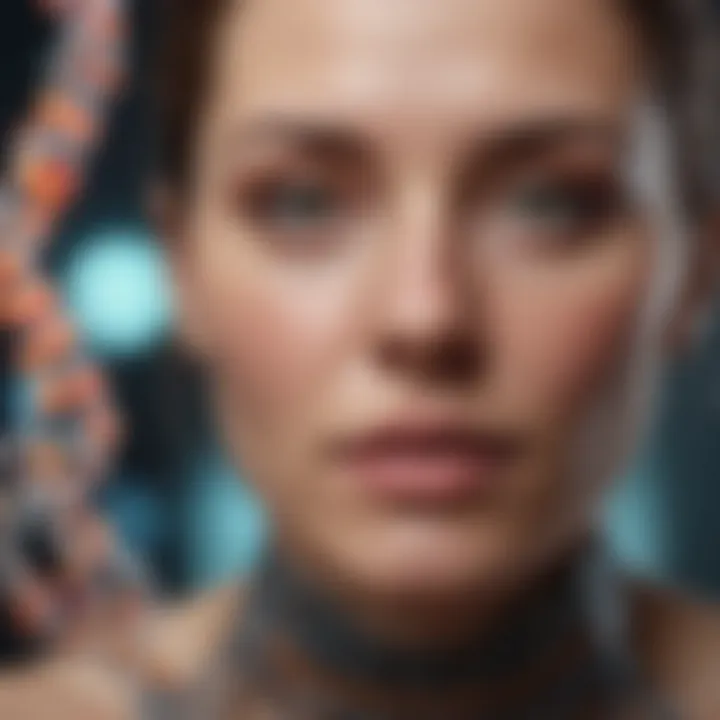
- Safety Assessments: Before any genetic intervention, comprehensive risk assessments must be conducted to ensure safety for individuals and ecosystems.
- Ethical Approval Processes: Research proposals should undergo rigorous ethical review by relevant committees to evaluate the potential impacts.
- Public Engagement: Engaging with the public and stakeholders helps to foster transparency and trust in genetic research. Feedback from diverse groups can shape regulations and ensure they address community concerns.
- International Standards: It is important to develop international agreements and standards that guide genetic research, accounting for the global implications of genetic technology.
Efforts to formalize these frameworks are underway globally, as researchers and policymakers recognize the urgent need to address ethical challenges in genetic research.
Applications of Genetics in Medicine
Genetics has become integral to modern medicine, influencing how we approach disease prevention, diagnosis, and treatment strategies. The application of genetic principles yields significant advancements that reshape healthcare practices and enhance patient outcomes. This section examines the key components of genetic applications in medicine, highlighting personalized approaches and cancer treatment innovations specifically.
Personalized Medicine Approaches
Personalized medicine tailors medical treatment to the individual characteristics of each patient. This approach considers genetic differences, lifestyle, and environmental factors. By analyzing a patient’s genetic makeup, healthcare providers can better predict the effectiveness of treatments and possible adverse effects.
Key benefits of personalized medicine include:
- Improved Efficacy: Treatments that align with a patient's genetic profile can lead to better outcomes.
- Reduced Side Effects: Understanding genetic predispositions allows for the minimization of adverse reactions to drugs.
- Targeted Therapies: Specific therapies can be designed, such as targeted treatments for specific genetic mutations.
For instance, pharmacogenomics, the study of how genes affect a person's response to drugs, is a vital aspect of personalized medicine. It can optimize drug therapy and promotes the concept that one size does not fit all in medical treatment.
"Every patient is unique; personalized medicine underscores the importance of tailoring healthcare to fit individual needs."
Genetics in Cancer Treatment
The application of genetics in cancer treatment is a pivotal development in the fight against this complex disease. Cancer is not a single entity but a collection of diseases with varied genetic underpinnings. Understanding these genetic variations allows for more precise treatments.
Key approaches to genetics in cancer treatment include:
- Genomic Profiling: Analyzing the genetic makeup of tumors enables oncologists to identify specific mutations. This information guides decisions about targeted therapies that aim to combat cancer effectively.
- Immunotherapy: Genetic insights lead to innovations like CAR-T cell therapy, where a patient’s T cells are modified to better attack cancer.
- Predictive Genetic Testing: Families at risk for hereditary cancers can receive valuable information that aids in early detection and preventive measures.
These developments showcase how genetics can personalize oncology, increasing survival rates and improving quality of life. As research continues, the integration of genetic data into clinical practice promises new horizons in cancer treatment.
Agricultural Genetics and Food Security
Agricultural genetics plays a vital role in addressing the challenges of food security worldwide. The increasing global population demands more food production, which places greater stress on agricultural systems. Genetic research can enhance crop resilience, yield, and nutritional quality. Through innovations such as genetic modification and traditional breeding techniques, scientists aim to create crops that can thrive in diverse environments and resist diseases.
Growth in agricultural genetics not only leads to improved food supply but also supports sustainable practices. By reducing the need for chemical inputs and enhancing ecosystem functions, genetically altered crops can contribute to environmental health. Thus, this topic carries significant relevance in discussions about future food policies and practices.
Genetically Modified Organisms (GMOs)
Genetically modified organisms have become a focal point in agricultural genetics. The primary aim of creating GMOs is to introduce desirable traits into plants, such as resistance to pests or tolerance to harsh conditions. For example, Bt corn has been engineered to contain a gene from the bacterium Bacillus thuringiensis, providing built-in resistance to specific pests.
The benefits of GMOs are clearer in certain contexts:
- Reduced pesticide usage: GMOs that express pest resistance can lead to lower pesticide application.
- Increased yields: Crops that withstand various stresses, including drought or disease, can boost production.
- Enhanced nutritional content: Certain GMOs are developed to increase vitamins or minerals in staple foods, promoting better health outcomes in populations facing deficiencies.
However, GMOs also invite criticism. Concerns include potential impacts on biodiversity and the long-term ecological consequences of introducing these plants into the environment. Moreover, public perception of GMOs varies widely, often influenced by environmental or health quality debates.
Impact of Genetic Research on Crop Yield
Research in genetics has a profound impact on crop yields. By utilizing techniques like marker-assisted selection, scientists identify desirable genes more efficiently. This boosts the rate at which new varieties are developed and can significantly improve food production.
Considerations include:
- Drought resistance: Crop varieties are developed to have better water use efficiency, thus maintaining yield even in water-scarce conditions.
- Disease resistance: In regions prone to specific diseases, genetic advancements lead to varieties that can withstand various pathogens, reducing crop loss.
- Nutrient efficiency: Some crops are modified to use soil nutrients more effectively, enhancing growth without depleting the soil.
In summary, the impact of genetic research on crop yield is evident. As climate change continues to pose challenges to agriculture, the importance of these advancements becomes even more apparent. By integrating genetic technologies, stakeholders can contribute to shaping a secure food future.
Technological Integration in Genetic Research
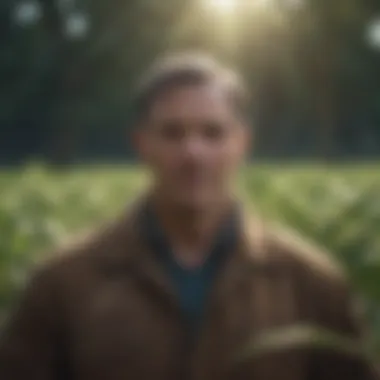
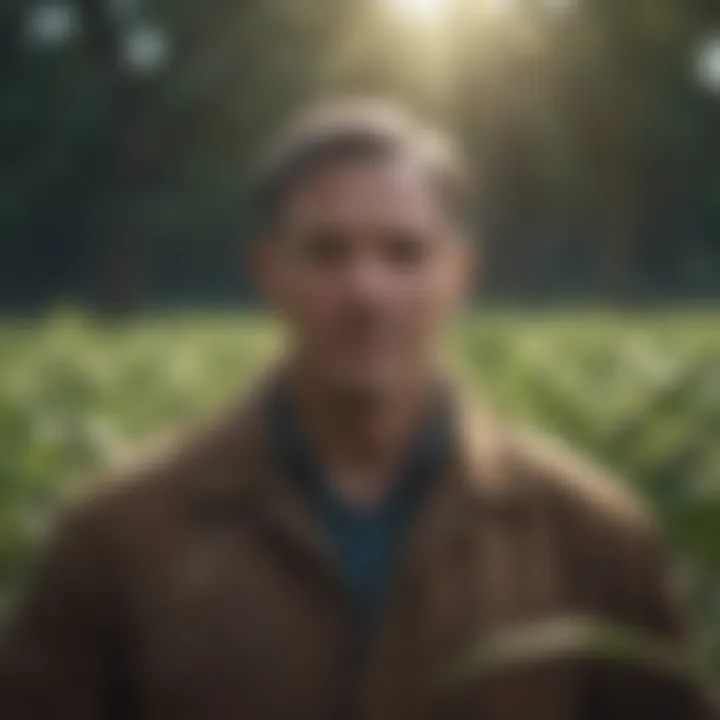
Technological integration in genetic research plays a pivotal role in advancing our understanding of complex biological systems. As genetics increasingly intersects with technology, new methods emerge that enhance research capabilities. This integration facilitates the analysis of vast amounts of genetic data, promoting insights that were previously unattainable. It is essential to recognize that the interplay between technology and genetics is no longer optional but a necessary component of modern research.
Bioinformatics and Data Analysis
Bioinformatics combines computer science, statistics, and biology, forming a vital framework for managing genetic data. The explosion of genomic information from sequencing technologies creates a challenge: how to analyze and interpret this data effectively. Bioinformatics provides tools that help researchers manage these large datasets.
Key components of bioinformatics include:
- Data management: Efficiently storing genetic data for easy access.
- Data analysis: Utilizing algorithms to identify patterns or anomalies.
- Visualization tools: Creating graphical representations of complex data.
By using bioinformatics, researchers can conduct more robust analyses, leading to discoveries in areas such as disease susceptibility and population genetics. Furthermore, it allows for more precise insights into gene interactions, which is crucial for understanding the genetic basis of traits.
Artificial Intelligence in Genomics
Artificial intelligence (AI) is rapidly transforming genomics by providing tools to make sense of the ever-growing data landscape. AI applications in genomics focus on enhancing predictive accuracy and operational efficiency in research. Machine learning, a subset of AI, is particularly notable for its capacity to identify complex patterns within large datasets.
Some applications of AI in genomics include:
- Predictive modeling: Algorithms can predict disease risk based on genetic profiles.
- Gene discovery: AI aids in identifying new genes linked to diseases.
- Personalized treatment plans: AI can help in tailoring specific treatment regimens for patients based on genetic information.
The integration of AI into genetic research can accelerate findings, reduce biases, and enhance the overall understanding of genetic factors in health and disease. As AI continues to evolve, its potential to streamline genetic research is immense, paving the way for innovative solutions in clinical and agricultural applications.
Impacts of Genetics on Public Health
Genetics plays a crucial role in public health. Understanding genetic factors can significantly enhance disease prevention, diagnosis, and treatment strategies. This section will discuss several key components such as genetic screening and the connection between genetics and infectious diseases.
Genetic Screening and Prevention
Genetic screening allows early detection of genetic disorders. It is an essential tool in preventive medicine. By identifying individuals at risk for certain diseases, healthcare providers can implement strategies to mitigate these risks. These strategies may include lifestyle changes, increased monitoring, or even preemptive treatments.
Predisposition testing is an important aspect of genetic screening. For example, BRCA1 and BRCA2 tests help assess the risk of breast and ovarian cancers. If a person tests positive, they can explore options like enhanced surveillance or prophylactic surgery. Early intervention can save lives.
Moreover, newborn screening is a standard practice in many countries. It identifies metabolic disorders and genetic conditions that can lead to serious health issues if not treated early. Examples include phenylketonuria (PKU) and cystic fibrosis. Through early intervention, many infants can lead normal, healthy lives.
While genetic screening offers advantages, it raises ethical questions. Concerns about data privacy and potential discrimination can arise. Patients must be adequately informed about how their genetic data will be used. Ethical frameworks should guide the implementation of screening programs to address these concerns effectively.
Role of Genetics in Infectious Diseases
The relationship between genetics and infectious diseases is complex. Understanding the genetic makeup of pathogens can lead to more effective treatments and preventive measures. For instance, the identification of specific genetic markers in viruses can help in developing targeted vaccines and therapies.
Furthermore, human genetics can influence susceptibility to infectious diseases. Certain genetic factors can determine how robust an individual's immune response is to infections. For example, people with mutations in their immune system genes may have varying responses to pathogens like HIV or tuberculosis.
In the context of public health, genetic studies inform the effectiveness of vaccines across different populations. Genetic diversity within populations can affect vaccine responses. This knowledge helps in creating more effective public health strategies.
"Genetic insights can guide interventions at both the individual and population levels, enhancing our ability to combat infectious diseases."
In summary, genetics has a profound impact on public health. Genetic screening can lead to earlier prevention and treatment of diseases. Meanwhile, insights from genetic research can enhance our understanding of infectious diseases and improve response strategies. Integrating genetic studies into public health frameworks is essential for advancing health outcomes and addressing emerging health challenges.
Future Directions in Genetic Research
Recent progress in genetic research shapes the landscape for future studies, providing new avenues for exploration. Understanding these future directions is crucial for researchers, educators, and medical professionals alike, as it bridges the gap between existing knowledge and innovative applications. Addressing genetic complexities is not only about understanding the structure of DNA but also about recognizing how these innovations can transform medicine, agriculture, and public health. This ongoing evolution presents unique challenges and opportunities that can redefine our approach to genetic research.
Emerging Areas of Focus
Several emerging areas in genetic research deserve attention. These include:
- Gene Editing Enhancements: Beyond CRISPR-Cas9, new methods such as prime editing are developing. These techniques promise more precise gene modifications, minimizing off-target effects. This precision can revolutionize treatments for genetic disorders.
- Synthetic Biology: Researchers are increasingly looking into synthetic biology. They aim to design and construct new biological parts, devices, or systems. This can lead to groundbreaking applications in biofuel production and environmental remediation.
- Artificial Intelligence Applications: AI is now helping to analyze large genomic datasets. It facilitates discoveries of novel genetic patterns and understandings of complex diseases. This partnership between AI and genetics will likely result in more effective personalized treatments.
- Microbiome Studies: The role of the microbiome in health and disease is gaining traction. Understanding how gut bacteria affect gene expression opens potential avenues for managing diseases. This could lead to more tailored therapeutic approaches based on individual microbiomes.
- Ethical Genomic Surveillance: With the rise of genomic research, ethical implications surrounding surveillance are increasing. It’s essential to address how data can be used while ensuring individuals’ rights to privacy. This will shape future research frameworks.
Predictions for Genetic Advancements
Looking ahead, predictions in the field of genetics often revolve around several pivotal themes. Key anticipations include:
- Personalized Medicine: Precision medicine is expected to grow significantly. Treatments tailored to individuals' genetic profiles will improve effectiveness and reduce side effects. This individualized approach has the potential to revolutionize fields like oncology.
- Gene Therapy Evolution: By 2030, researchers anticipate more mature gene therapies that may offer cures, not just treatments. Conditions previously deemed untreatable could see viable solutions.
- Collaborative Research Models: Increased collaboration across global research institutions will likely enhance gene research. Sharing datasets and findings may fast-track discoveries, enabling faster response to emerging health issues.
- Regulatory Developments: As new technologies emerge, regulations will evolve. Governments may implement stronger guidelines to govern genetic research ethics and applications, shaping future practices to be more responsible.
"The landscape of genetic research is continually evolving amidst technological and ethical advancements. Understanding these changes can enhance research strategies and societal impact."